Introduction
Exposed rock represents only 0.18% of the surface area on the continent of Antarctica (Burton-Johnson et al. Reference Burton-Johnson, Black, Fretwell and Kaluza-Gilbert2016). Outcrops are concentrated in mountain ranges along the Transantarctic Mountains and in peripheral regions such as the Antarctic Peninsula, Ellsworth Mountains and in a few areas around the eastern perimeter of the continent. Because of the lack of exposure, exploration of remote mountain ranges and nunataks (isolated peaks surrounded by snow and ice) provide important sources of data for our understanding of continental-scale tectonic events and Antarctic geologic history. This paper presents new faunal evidence that confirms the age and stratigraphic position of limestone exposures at Postel Nunatak in the Patuxent Range, which had been mapped as middle Cambrian Nelson Limestone (Schmidt & Ford Reference Schmidt and Ford1969); it also provides an interpretation of the depositional setting of the limestone, presents a reconnaissance-level survey of variations in stable isotopes of carbon and oxygen in carbonate rock samples, and discusses the broader implications of these data for regional correlation of Nelson Limestone and adjacent strata of uncertain stratigraphic affinities in the Patuxent Range.
Research efforts on the Cambrian rocks of Antarctica over the last two decades have refined our understanding of the timing and tectonic setting of the Cambrian to Ordovician Ross Orogeny (Rowell et al. Reference Rowell, Rees and Evans1992, Reference Rowell, van Schmus, Storey, Fetter and Evans2001, Stump Reference Stump1995, Storey et al. Reference Storey, MacDonald, Dalziel, Isbell and Millar1996, Curtis Reference Curtis2002, Curtis & Storey Reference Curtis and Storey2003, Curtis et al. Reference Curtis, Millar, Storey and Fanning2004, Federico et al. Reference Federico, Crispini, Capponi and Bradshaw2009). Evidence of deformation during the Ross Orogeny is expressed along the entire margin of East Antarctica from northern Victoria Land to the Pensacola Mountains, in some of the peripheral terranes and across the Australian margin of western Gondwana. Deformation probably resulted from accretion and partial obduction of an arc (back-arc) trench system, a collision which Federico et al. (Reference Federico, Crispini, Capponi and Bradshaw2009) argued occurred during the opening and closing of a back-arc basin, similar to the Cambrian record of the South Island of New Zealand. If this comparison is correct, accretion during this orogeny need not have involved collision with a major terrane or continental landmass. In the Pensacola Mountains, Curtis et al. (Reference Curtis, Millar, Storey and Fanning2004) have proposed a model for episodes of both contractional and extensional deformation related to low-angle and normal subduction of oceanic platforms. Lower and middle Cambrian carbonate lithosomes bracketed (i.e. were deposited before and after) the onset of the Ross Orogeny. The distribution of both lower and middle Cambrian carbonates, their ages, thicknesses and depositional settings therefore assume importance for our understanding of the timing, extent and significance of intra-orogenic processes such as subsidence and relative quiescence along this active tectonic margin.
Location
The Pensacola Mountains are located at the Weddell Sea terminus of the Transantarctic Mountains (Fig. 1). Exposed rocks in the mountain ranges that comprise the Pensacola Mountains are separated more widely than more-or-less contiguous exposures that stretch from northern Victoria Land to the Horlick Mountains. Large areas of relatively well-exposed rock in the Pensacola Mountains include, from south-west to north-east, the Pecora Escarpment, Patuxent Range, Rambo Nunataks, Neptune Range (including the Schmidt Hills and Williams Hills), Forrestal Range, Cordiner Peaks, Dufek Massif and Argentina Range (including the Panzarini Hills and Schneider Hills). The Shackleton Range is not part of the Pensacola Mountains and is located c. 240 km north-east of the Argentina Range. The Thiel Mountains are also separate from the Pensacola Mountains and are located c. 180 km W-SW of the Pensacola Mountains (Fig. 1).

Fig. 1a Map of Antarctica. b. Detailed map showing the Pensacola Mountains and adjacent areas mentioned in the text (see Fig. 9).
The Patuxent Range is shown on US Geological Survey 1:250,000 Antarctic reconnaissance series maps for the Thomas Hills and northern part of the Pecora Escarpment quadrangles. It consists of a series of relatively low-elevation peaks and ridges between the Foundation and Patuxent ice streams (Fig. 2). The main parts include the Thomas Hills, Anderson Hills, Mackin Table, and clusters of nearby nunataks and isolated ridges. One of these isolated outcrops is Postel Nunatak, which is located near the western edge of the Patuxent Range c. 6 km west of the Des Roches Nunataks, the nearest bedrock exposures.

Fig. 2 Map of Patuxent Range including parts of US Geological Survey Antarctica 1:250 000 topographical reconnaissance maps for the Thomas Hills and Pecora Escarpment quadrangles. Distribution of the limestone outcrops mapped as Nelson Limestone and Devonian Sandstone in Brazitis Nunatak is shown in Schmidt & Ford (Reference Schmidt and Ford1969). All other outcrops were mapped as Patuxent Formation.
Previous studies
Field parties from the US Geological Survey first explored the geology of the Patuxent Range in 1962–63 (see Schmidt & Ford Reference Schmidt and Ford1969). Geologic mapping of the remainder of the Pensacola Mountains and Thiel Mountains followed during the 1963–64 and 1965–66 field seasons. Previous studies of the Nelson Limestone are limited to the initial lithologic descriptions of the unit from the Neptune Range (Schmidt et al. Reference Schmidt, Dover, Ford and Brown1964, Reference Schmidt, Williams and Nelson1978), descriptions of fauna collected there and from correlative exotic moraine material (Palmer & Gatehouse Reference Palmer and Gatehouse1972, Palmer & Rowell Reference Palmer and Rowell1995, Lieberman Reference Lieberman2004, Bassett-Butt Reference Bassett-Butt2016), and subsequent sequence-stratigraphic studies (Evans et al. Reference Evans, Rowell and Rees1995). On the geologic map of the Pensacola and Thiel mountains, which included the Patuxent Range, the Nelson Limestone was mapped at the Des Roches Nunataks, Postel Nunatak and an unnamed nunatak with an elevation of 1320 m (see Fig. 3c; Schmidt & Ford Reference Schmidt and Ford1969). Although these limestone outcrops were mapped as Nelson Limestone, no detailed descriptions of the geology nor corroborative evidence correlating these limestone exposures using fauna has been published until now.

Fig. 3 Outcrop images. a. Postel Nunatak from east; beds dip southward. b. Sample locality E93-06. c. Close-up of E93-06. Trilobites were collected from dark grey beds. d. Oblique composite photo of lower Postel Nunatak (person circled). e. Base of stratigraphic section. f. Lower beds c. 6 m. Resistant beds of intraclastic conglomerate and peloid grainstone above top of Jacob’s staff. g. Peloid grainstone and microbialites above burrowed lime mudstone. Boot at 39 m. h. Thick beds of burrowed and fenestral mudstone. Person at 190.5 m. i. Laminated mudstone at 244 m. j. Laminated and fenestral mudstone from upper section.
Most subsequent studies of Palaeozoic rocks in the Pensacola Mountains have focused field efforts in places other than the Patuxent Range, but Curtis (Reference Curtis2002) visited Snake Ridge and Mount Campleman along the northern edge of Mackin Table, where he collected additional structural data from the Patuxent Range. These data were used to develop a high-resolution structural interpretation that suggested multiple phases of deformation and were integrated with an isotope geochronology and kinematic model for shallowly focused deformation in the Pensacola Mountains. In short, the polyphase deformation was interpreted as spanning the earliest to latest phases of the Ross Orogeny with the latest phase of deformation most conspicuous (Curtis & Storey Reference Curtis and Storey2003, Curtis et al. Reference Curtis, Millar, Storey and Fanning2004, see also Rowell et al. Reference Rowell, Rees and Evans1992, Reference Rowell, van Schmus, Storey, Fetter and Evans2001, Storey et al. Reference Storey, MacDonald, Dalziel, Isbell and Millar1996). Understanding the tectonic history relies on isotope geochronology as well as faunal collections and regional patterns of sedimentation.
Cambrian biostratigraphy in Antarctica is based largely on trilobites and several papers have considered this subject in detail. Complete consideration of the literature on this topic would require extensive discussion and goes beyond the scope of the present work. Here, only an entry into the literature is provided. In particular, Palmer & Gatehouse (Reference Palmer and Gatehouse1972) used material, primarily collected from boulders in moraines, to establish a preliminary Cambrian biostratigraphy for parts of the continent, with special focus on the Transantarctic Mountains. Soloviev & Grikurov (Reference Soloviev and Grikurov1978, Reference Soloviev and Grikurov1979) presented additional discoveries of trilobites from this region. Subsequently, Palmer & Rowell (Reference Palmer and Rowell1995) extended this work with a detailed analysis of early Cambrian (Series 2) trilobite faunas from the Schneider Hills. Lieberman (Reference Lieberman2004) provided additional details on the systematics and biostratigraphy of the Cambrian trilobite faunas from the Transantarctic Mountains, especially fossils from the Nelson Limestone, where it crops out in the Neptune Range, and provided biostratigraphic revisions for strata consigned to Cambrian Series 3.
Trilobite faunas have also been recovered from other parts of Antarctica as well. For example, Cooper et al. (Reference Cooper, Jago and Begg1996), Wolfart (Reference Wolfart1994), Jago & Cooper (Reference Jago and Cooper2007), Jago et al. (Reference Jago, Bentley and Cooper2011) and Bentley et al. (Reference Bentley, Jago and Cooper2016) provided information on the systematics and biostratigraphy of Cambrian trilobites from northern Victoria Land. Soloviev et al. (Reference Soloviev, Popov and Samsonov1984) and Jago & Webers (Reference Jago and Webers1992) focused on the systematics and taxonomy of Cambrian trilobites from West Antarctica. Finally, Encarnación et al. (Reference Encarnación, Rowell and Grunow1999) also included information on Cambrian trilobites, and their bearing on the regional biostratigraphy, from the Dronning Maud Mountains.
Methods and materials
Studies of the Pensacola Mountains during the 1993–94 field season focused on collection of igneous rock samples from the Schmidt Hills, Williams Hills and Washington Escarpment in the Neptune Range for isotope geochronology studies (Van Schmus et al. Reference Van Schmus, McKenna, Gonzalez, Fetter and Rowell1997), examination of field relationships associated with the Nelson Limestone, Gambacorta Formation and Wiens Formation (Evans et al. Reference Evans, Rowell and Rees1995, Rowell et al. Reference Rowell, van Schmus, Storey, Fetter and Evans2001), and exploration of the stratigraphic succession in the Patuxent Range. Air transportation support in the Pensacola Mountains was provided by a de Havilland Twin Otter, which made it possible to visit and collect from remote areas that otherwise would have been inaccessible.
On 29 November 1993, McKenna and Evans landed in the Patuxent Range, c. 2.5 km east of Postel Nunatak. Fieldwork at this locality was completed on 30 November 1993. A stratigraphic section was measured and described, and trilobite parts and fragments were collected between 318–322 m. In addition to crack-out collections, c. 15 kg of bulk rock samples were taken from the outcrop. The entire excursion transpired in c. 36 hours. Deteriorating weather conditions required air extraction from the landing site on 1 December 1993 and return to the put-in site and base camp in the Roderick Valley of the Neptune Range.
Bulk faunal sample E93-06 was split and partly prepared by Evans and Rowell in 1994 and is currently housed in the University of Kansas Biodiversity Institute, Division of Invertebrate Paleontology (KUMIP). Specimens have been evaluated further by Lieberman herein. Additionally, 11 hand samples were collected from the measured stratigraphic section and prepared as polished slabs representative of different depositional facies (Table I).
Table I E93-06 (Postel Nunatak) sample lithology descriptions (see Fig. 7) and isotopic composition values (see Fig. 8) of replicates from bottom, middle and top of each sample, respectively.

V-PDB=Vienna-PeeDee Belemnite.
The obverse sides of the polished slabs were prepared for carbonate carbon and oxygen stable isotopic analysis by washing in an ultrasonic bath and rinsing in deionized water prior to drilling to generate powders for analysis. Three samples were drilled from each slab for a total of 33 samples. Homogenous lime mudstone layers were targeted and microdrilled from surfaces without obvious secondary diagenetic features. Carbonate carbon (δ13C) preserved in mudstone has been shown to be resistant to alteration over a range of diagenetic settings encountered in carbonate successions, whereas δ18O is easily reset during diagenesis (O’Neil Reference O’Neil1987).
Measurements of carbon (δ13C) and oxygen (δ18O) isotopic values were made at the University of Missouri biogeochemistry stable isotope laboratory. Approximately 50 µg of powdered carbonate was reacted with 103% phosphoric acid for four minutes at 70 °C using a Kiel III automated carbonate device from Thermo Fisher Scientific. Evolved CO2 gas was cryogenically cleaned and analysed on a Thermo Finnigan DeltaPlus mass spectrometer operated in dual inlet mode. Results were normalized to the National Bureau of Standards (NBS)-19 standard with nominal values of -2.20‰ and +1.95‰ for δ18O and δ13C, respectively. Results for uncorrected measurements of NBS-19 run during the course of the study indicate an external analytical precision of 0.02‰ (±1σ) for δ13C and 0.06‰ (±1σ) for δ18O values. All results are reported in delta (δ) notation relative to the Vienna-PeeDee Belemnite (V-PDB) scale.
Trilobites were recovered from the upper burrowed lime mudstone beds from 318–322 m, in what is referred to as sample E93-06 in the KUMIP collections (Fig. 4). One set of specimens possess the diagnostic and characteristic features of Solenopleura pruina presented in Palmer & Gatehouse (Reference Palmer and Gatehouse1972) and Lieberman (Reference Lieberman2004). Solenopleura pruina has previously been documented from morainal boulders recovered on Mount Spann in the Argentina Range (Palmer & Gatehouse Reference Palmer and Gatehouse1972, Soloviev & Grikurov Reference Soloviev and Grikurov1979), and from several localities and stratigraphic levels of the Nelson Limestone in the Neptune Range (Lieberman Reference Lieberman2004); indeed, it is among the most common trilobites in the Nelson Limestone in the Neptune Range. Palmer & Gatehouse (Reference Palmer and Gatehouse1972) indicated S. pruina was moderately common in the moraine boulder from Mount Spann as well. The species closely resembles other species assigned to Solenopleuridae in general, and Solenopleura Angelin, 1854 in particular, that have been described from northern Victoria Land by Wolfart (Reference Wolfart1994) and Cooper et al. (Reference Cooper, Jago and Begg1996), and from West Antarctica by Jago & Webers (Reference Jago and Webers1992) (see Lieberman Reference Lieberman2004 for further discussion). Solenopleura pruina is rare at Postel Nunatak and represented by KUMIP 416072 and questionably KUMIP 416073.

Fig. 4 Images of trilobites collected in sample E93-06 from c. 318–322 m in the measured stratigraphic section. a. Solenopleura pruina shown ×22. b. Suludella? davnii shown ×5.
Another set of trilobite specimens possess the diagnostic and characteristic features of S.? davnii presented in Palmer & Gatehouse (Reference Palmer and Gatehouse1972). Suludella? has previously been reported from a boulder in a moraine on Mount Spann in the Argentina Range, where it is represented by S.? davnii and S.? spinosa, and from two localities in the Neptune Range, where it is represented only by S.? spinosa (Lieberman Reference Lieberman2004). It was also reported from northern Victoria Land by Wolfart (Reference Wolfart1994), though the material is so incomplete and poorly preserved that it was difficult to verify this taxonomic assignment (Lieberman Reference Lieberman2004). Palmer & Gatehouse (Reference Palmer and Gatehouse1972) also discussed why the Antarctic material could only be questionably assigned to Suludella (a genus defined initially on material from Siberia); since there have not been any phylogenetic or substantive taxonomic revisions to the genus subsequent to that publication, we follow the uncertain generic assignment. Suludella? davnii is more abundant than S. pruina and is represented by several cranidia and librigena (KUMIP 407995, 497996 and 416069–416071) as well as a partial pygidium (KUMIP 407997). No other trilobite species were identified in the material recovered from the Postel Nunatak.
Results
Lithostratigraphy
Beds on Postel Nunatak dip south-east at angles ranging from c. 30° to 50° (Figs 3 & 5). The base for the measured stratigraphic section was established at the north-west end of the nunatak. The line of section followed a series of ridgelines, ran along the sides of prominent cliffs and was offset between scree and snow-covered couloirs (Figs 3, 5 & 6). The uppermost part of the section followed a dip-slope and ridgeline to the south-east. A few faults with minor offset were crossed in the line of section (Fig. 6); however, although the potential existed for more significant offset along bedding planes, bedding was generally continuous on the scale of a few metres to tens of metres along strike. The distribution of Nelson Limestone outcrops in the Patuxent Range would be consistent with one or more long fold axes between older strata to the north and east and younger strata to the south (see Fig. 2).

Fig. 5a Stereoscopic aerial photographs of Postel Nunatak, numbers TMA 1503-336 and -337, taken 17 December 1964. b. Geologic map of Postel Nunatak. Approximate line of stratigraphic section indicated by bold black lines. Location of sample E93-06 shown with an asterisk. East summit (elevation 1449 m) and west summit indicated by plus signs.

Fig. 6 Measured and described stratigraphic section at Postel Nunatak.
The 393 m thick succession consists mostly of lime mudstone and peloid grainstone (Table I, Fig. 7). There is little variation in lithofacies and about ten common carbonate rock types are present. The dominant lithofacies include thick intervals of regular and irregular laminae to thin beds of lime mudstone (Fig. 7b & f), intervals of intraclast conglomerate composed of rip-up clasts (up to 3 cm in length) of laminae from laminated lime mudstone (Fig. 7g), thin intervals of burrowed lime mudstone to wackestone to grainstone (Fig. 7c–e, h, j & k; some of which have yielded trilobites carapaces, Fig. 7h), thin to medium beds of peloid grainstone and wackestone; and thick and massive beds of peloid grainstone, with and without sparry calcite burrow fills and fenestral pores (Fig. 7a & i). At both the top and the bottom portions of the measured section, lithofacies were interbedded on the scale of 0.5–5 m. However, in the middle part of the exposure, a thick interval of monotonous fenestral mudstone seems to record a markedly long period of depositional stasis. One rare cross-bedded peloid grainstone is present in the upper part of the section at 245 m. In the upper part of the section, c. 370 m, beds of laminated lime mudstone show more regular layering and seem to be bundled in upward thinning and upward thickening packages that range to >20 cm thick.
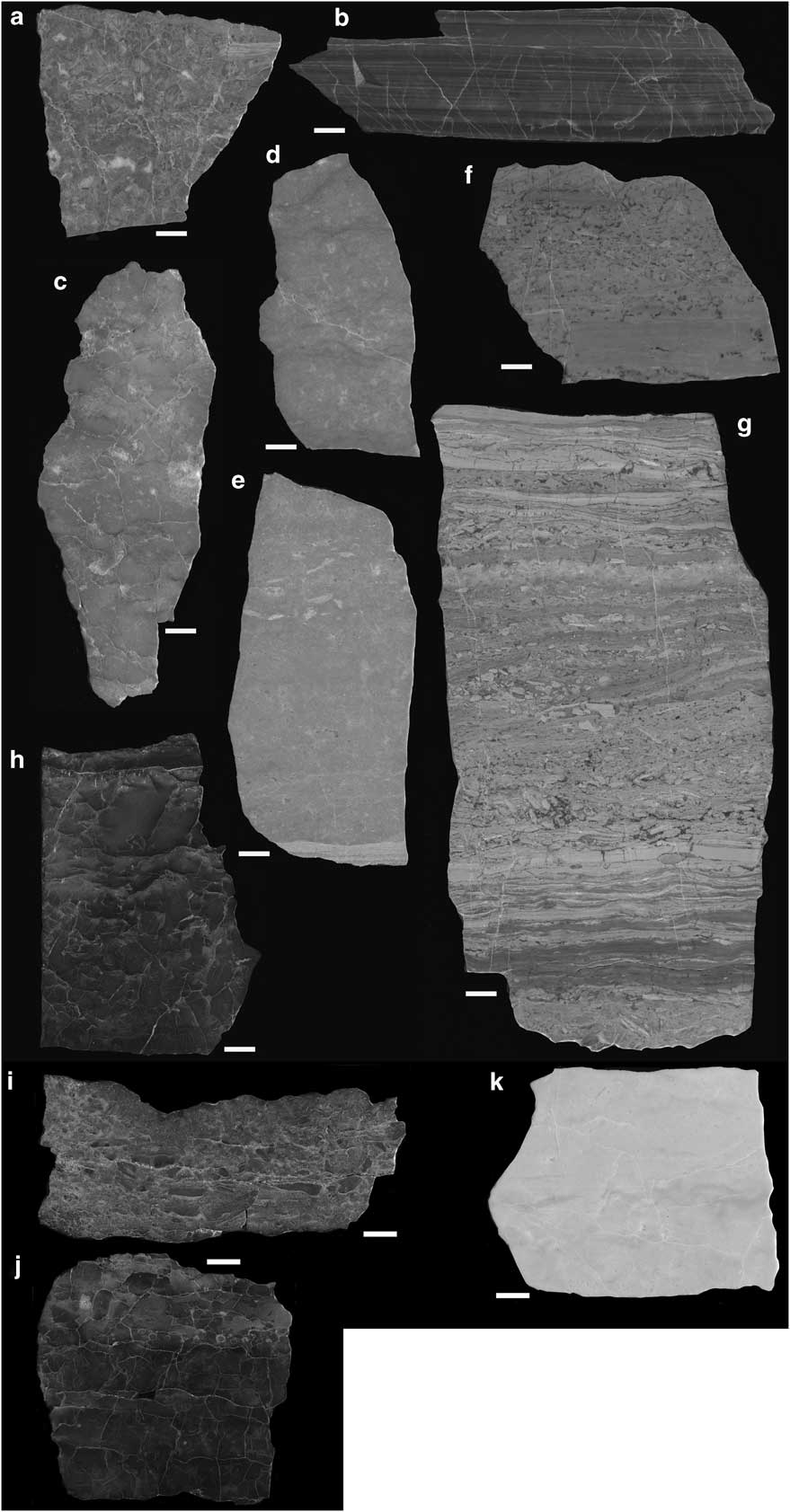
Fig. 7 Polished limestone slabs representative of lithofacies at Postel Nunatak (see Table I for detailed descriptions and values of stable isotopes). a. Intraclast grainstone to packstone (4.6 m), b. laminated lime mudstone (11.3 m), c. peloid grainstone (44.5 m), d. peloid grainstone (45.7 m), e. laminated peloid packstone to grainstone to fenestral peloid packstone (110.3 m), f. laminated lime mudstone to intraclast grainstone (243.8 m), g. laminated lime mudstone to intraclast grainstone (262.1 m), h. lime mudstone (318.5 m), i. intraclast grainstone with lime mudstone clasts (320.6 m), j. lime mudstone (321.9 m) and k. lime mudstone (356 m).
Stable isotopes of carbon and oxygen
Hand samples were used to survey a gross-scale trend in the stable isotopic signature of the carbonate succession (Table I, Figs 7 & 8). For the 33 samples analysed, values for δ18O ranged from -11.63 to -6.38‰, and values for δ13C ranged from 3.63 to -0.72‰ (Table I). Low values for δ18O are expected for bulk carbonate analyses of early Palaeozoic limestone and suggest a diagenetic overprint at elevated temperatures during burial and lithification. The anomalously high value for δ13C was obtained from powder from an unusual dark lamina in the lower part of a laminated lime mudstone (Fig. 7b). In other samples, variation among the three positions drilled on each sample tend to be less than or equal to differences in values averaged for each sample. The lowest δ13C values were associated with the intraclast grainstone to packstone sample collected near the base of the section (Fig. 7a). Two additional samples were re-analysed and similar results were obtained from each. The δ13C and δ18O measurements are positively correlated (overall r 2=0.43 with the anomalous δ13C omitted) but the correlation seems to result from the values for samples with the lowest δ13C values (<0‰). In samples with δ13C values <0‰ there is a strong positive correlation between δ13C δ18O values (r 2=0.69) whereas there is no correlation between the two measures (r 2=0.02) for samples with δ13C values >0‰ (Fig. 8b). Positive correlation suggests alteration as do the low δ18O values. However, the bend in the isotopic cross-plot at ~δ13C=0‰ suggests that diagenetic carbon from a different source (perhaps remineralized organic matter) is a major confounding variable only in the most altered samples and less altered samples may have δ13C values close to original values consistent with the generality that δ13C is a more robust than δ18O values in carbonate-rich systems.

Fig. 8a δ13C and δ18O values (x-axes) plotted against stratigraphic position in the measured stratigraphic section (y-axes). b. Cross-plot of δ13C and δ18O, excluding subsamples with anomalously high values. Note the marked deviation, which is interpreted as a product of alteration in δ18O. Lines of correlation and Pearson’s products are shown for the two subpopulations. V-PDB=Vienna-PeeDee Belemnite.
Discussion
The analysis of sediments and trilobites recovered from an extensive 393 m thick succession in the Postel Nunatak provides new insights into the age relationships of this Cambrian deposit and suggests a close stratigraphic association with the Neptune Limestone in the Neptune Range. In particular, the fauna suggests the unit lies within Cambrian Series 3, and is either Drumian or Drumian plus Guzhangian in age. Additional analysis of the relationships of this unit to equivalent as well as overlying and underlying strata may ultimately provide further insight into the regional geology of Antarctica and the timing of the Ross Orogeny. Thus, some of these other deposits warrant mentioning. For instance, Series 2 and 3 (early and middle) Cambrian limestones or correlative siliciclastic strata are exposed across part of the Transantarctic Mountains to the Shackleton Range. Both Cambrian Series 2 and 3 faunas have been recovered from blocks in moraines near Mount Spann in the Panzarini Hills of the northern Argentina Range (Palmer & Gatehouse Reference Palmer and Gatehouse1972). Series 2 Cambrian limestone reefs are present in the Schneider Hills (Rowell et al. Reference Rowell, Rees and Evans1992). Cambrian Series 2 (and older) limestones are missing in the Neptune Range and presumably were removed by uplift and erosion during an early phase of the Ross Orogeny. Cambrian Series 2 (and older) strata equivalent to the Schneider Hills Limestone may be missing in the Patuxent Range, or they may be covered by snow and ice.
The interpretation of carbonate facies relies on modern analogues. Irregular laminae and thin beds of lime mudstone compare favourably with planar microbialites. Planar microbialites (including stromatolites) are common in Florida Bay tidal island interiors, where episodic wetting and drying of sediment has led to development of thick successions of irregularly laminated carbonate muds with desiccation cracks, interstratified with binding organic material (Enos & Perkins Reference Enos and Perkins1979). Lime mudstone intervals that contain regular laminae and thin beds often occur in bundles with marked thickening and thinning that would suggest episodic slack-water deposition. These features are comparable to laminae that have been influenced by tides and lunar cycles and are interpreted as tidal laminites (Ginsburg Reference Ginsburg1975). Both regular and irregular varieties of laminated mudstone accumulated in shallow water ranging from upper intertidal to supratidal settings (Wilson Reference Wilson1975). Intraclast conglomerates are commonly associated with both regular and irregular laminated mudstone facies and tend to have preserved shelter pores. Such lithologies are interpreted as storm deposits (Ager Reference Ager1974).
The lime mudstone to peloid grainstone facies is interpreted as having accumulated in a shallow water lagoonal setting. Fenestral pores indicate wetting and drying of the sediment. Rare to common burrows indicate marine deposition in a well-oxygenated, normal to somewhat restricted salinity setting. Burrows in these massively bedded intervals were commonly filled with sparry calcite, which indicates relatively rapid cementation early in the diagenetic history of this succession. Equant calcite spar is consistent with meteoric diagenesis where sediments may have been exposed to freshwater dissolution and subsequent precipitation of sparry carbonate cement. Faint horizontal laminae within these successions would suggest this facies was closely linked and perhaps juxtaposed with the supratidal laminite and microbialite facies.
Burrow-mottled carbonate muds generally accumulate below normal fair weather wave base, but water depth may vary and can be fairly shallow in protected areas that are sheltered by barriers associated with supratidal carbonate banks. The presence of trilobites would indicate at least episodic normal salinity and shallow marine conditions, but the depauperate nature of the collections suggest a restricted setting. The sole fossiliferous interval from 318–322 m is relatively dark, suggestive of a higher content of organic carbon.
In the entire succession, depositional environments ranged from shallow subtidal to supratidal settings. Repeated shallowing-upward trends are recognized within the succession (Fig. 6), but it is difficult to determine from available information if these are related to an extrinsic forcing mechanism (e.g. Milankovitch cyclicity) or episodic allocyclic changes among facies within a broad, shallow depositional setting. A few smaller scale packages of cyclic carbonate laminae indicate tidal influence. From the unit thickness and trends in depositional environments, it would seem possible to correlate intervals of dominantly supratidal and subtidal lithofacies with three depositional cycles recognized in exposures of the Nelson Limestone in the Neptune Range (Evans et al. Reference Evans, Rowell and Rees1995), except for the fact that the base and top of the section at Postel Nunatak are not exposed. The marine interval present in the type area near Nelson Peak, above the mostly non-marine valley-fill sequence, include: i) an initial transgressive sequence dominated by muddy carbonates, ii) a carbonate shoal and iii) a deepening–shallowing succession that was truncated below volcanics of the Gambacorta Formation. Three distinct depositional sequences of strata are present at Postel Nunatak. All are shallow water successions, but the middle, dominantly lagoonal interval compares favourably with the middle carbonate shoal interval in the Neptune Range. In both the Neptune and Patuxent ranges, depositional environments abruptly deepen in the overlying upper sequence, where mud-dominated subtidal successions have yielded a trilobite fauna. The upper sequence has yielded the Amphoton oatesi Palmer & Gatehouse zone fauna in both areas (Lieberman Reference Lieberman2004). Regardless of the plausible intraformational correlations, the shallow water succession at Postel Nunatak is clearly a more restricted setting, and one can argue from distribution of the Nelson Limestone that the Neptune Range was located in a basinward setting, which is consistent with deepening toward the north illustrated in a cross-section of Nelson Limestone in the Neptune Range (Evans et al. Reference Evans, Rowell and Rees1995, Fig. 5).
The carbon isotope trend at Postel Nunatak is intriguing and surprisingly coherent given that we were working from a limited number of samples collected to document lithology. Further, screening for strong diagenetic overprints using correlation with δ18O values, suggests δ13C values may approximate depositional values in many samples. It seems higher resolution sampling would have good potential for documenting patterns of secular variation that reflect regional and global changes to carbon cycling and can be useful in chemostratigraphic correlations.
With the caveat explicitly stated that our sample spacing is too coarse to provide meaningful independent constraints on interpretations, we suggest the δ13C data do support a working hypothesis concerning the chronostratigraphic position of the Nelson Limestone when combined with stratigraphic and palaeontological observations. Specifically, the pattern of variation within the Nelson Limestone compares favourably with the Drumian carbon isotope excursion (DICE). The DICE event in Cambrian Series 3 is a significant shift in the chemostratigraphic record found a little above the first appearance datum of Ptychagnostus atavus (Tullberg Reference Tullberg1880), and it has been recognized in many parts of the world (Zhu et al. Reference Zhu, Babcock and Peng2006, Babcock et al. Reference Babcock, Robison, Rees, Peng and Saltzman2007, Howley & Jiang Reference Howley and Jiang2010).
Other implications from this project have bearing on the status of what has been termed the Patuxent Formation in Antarctica (Fig. 9). Much of what had been originally mapped as Patuxent Formation in the Neptune Range was initially treated as Precambrian (Schmidt et al. Reference Schmidt, Williams and Nelson1978). Rowell et al. (Reference Rowell, van Schmus, Storey, Fetter and Evans2001) reported that deformed rocks cut by angular unconformity below the Nelson Limestone in the eastern Neptune Range have yielded detrital zircons that are Neoproterozoic in age although some strata may be partly correlative with the early Cambrian (Terreneuvian Series) in age. In contrast, rocks mapped as Patuxent Formation in the Schmidt Hills, Williams Hills, Rambo Nunataks and one sample from the Patuxent Range have yielded post-late middle Cambrian isotopic age dates (Van Schmus et al. Reference Van Schmus, McKenna, Gonzalez, Fetter and Rowell1997, Rowell et al. Reference Rowell, van Schmus, Storey, Fetter and Evans2001). Rowell et al. (Reference Rowell, van Schmus, Storey, Fetter and Evans2001) referred to the latter as Patuxent Formation in a restricted sense and proposed the name Hannah Ridge Formation for siliciclastic rocks that are demonstrably truncated below the Nelson Limestone in the eastern Neptune Range. These stratigraphic relationships have led some investigators (e.g. Curtis et al. Reference Curtis, Millar, Storey and Fanning2004) to suggest that Patuxent Formation in the Patuxent Range may be entirely post-middle Cambrian (Furongian Series) in age. This assignment could be problematic as it suggests that deep water siliciclastic deposition of several thousand metres of turbiditic sandstone and quartzite (see Schmidt & Ford Reference Schmidt and Ford1969) would have post-dated deposition of the Nelson Limestone, and that the entirety of the Patuxent Formation would be correlative with volcanic rocks of the Gambacorta Formation and overlying Weins Formation in the Neptune Range. At present, there is no tectonic mechanism that could explain this association.

Fig. 9 Correlation chart of stratigraphic units in the Pensacola Mountains and adjacent mountain ranges.
Instead, the distribution, depositional settings and level of deformation of the Nelson Limestone, especially in the Patuxent Range, would suggest that at least part of what was originally mapped as the Patuxent Formation by Schmidt & Ford (Reference Schmidt and Ford1969) may be older than the Nelson Limestone and correlative with the Hannah Ridge Formation of the eastern Neptune Range (Fig. 9). This uncertainty in the stratigraphic position of the Patuxent Formation and its relationship to the Hannah Ridge Formation has implications regionally and is not independent from our understanding of the relationship between the Hannah Ridge Formation and the lower Cambrian Schneider Hills Limestone in the Argentina Range (e.g. Faure & Mensing Reference Faure and Mensing2010, p. 246).
Conclusions
We confirm that limestone exposures at Postel Nunatak are correlated with the late middle Cambrian Nelson Limestone of the Neptune Range. Trilobites from the upper part of these exposures help to establish a Drumian or earliest Guzhangian age (Cambrian Series 3) for the uppermost strata. Carbonate lithofacies are interpreted as shallow subtidal marine and peritidal deposits. The regional distribution of the Nelson Limestone indicates an overall basinward direction and relative deepening to the north. Stable isotopic analyses are consistent with these findings and suggest potential exists for chemostratigraphic study including the possibility of documenting the position of the middle Cambrian DICE event within the section, but further study at higher resolution is needed to realize this goal.
Acknowledgements
This work was made possible through grants from the National Science Foundation Office of Polar Programs, numbers DPP 9117444 and OPP-9909302 to the University of Kansas. We are grateful to the staff and contractors serving the US Antarctic Program McMurdo Station for their mission support. We also want to thank our pilot Harry Hanlan and his flight crew from Kenn Borek Air for their support in the deep field. We thank reviewers and editors of the manuscript for their thoughtful comments, which greatly improved this paper. We are grateful to Julien Kimmig for facilitating the loan of specimens and also assistance with specimen photography, and also to the Departments of Ecology & Evolutionary Biology and Geology for providing resources. Evans was supported for writing and further investigation of the material presented here during sabbatical leave from Missouri State University; he is grateful for assistance with arranging the sabbatical from the Graduate College, College of Natural and Applied Sciences, and Department of Geography, Geology, and Planning. He also thanks Sara Ratcliffe for assistance scanning black and white film, along with various colleagues at the University of Swansea for resource support during the course of his sabbatical.
Author contributions
The efforts of each author are 20%. Evans oversaw the project, wrote part of the manuscript and participated in the collection of field data. McKenna wrote part of the manuscript and participated in the collection of field data. Lieberman wrote part of the manuscript, prepared and identified the trilobites, and continues to manage the lithologic sample and faunal collections. Weichert wrote a portion of the manuscript and oversaw the sample preparations for examining stable isotopes. MacLeod wrote part of the manuscript, ran isotopic sample analyses and interpreted the data. In addition to individual contributions, each author collectively contributed to the overall editing and organization of the manuscript.