Internal environment and homeostasis
Claude Bernard (Reference Bernard1865) introduced the idea of internal environment–internal milieu, as the fluid compartment within which cells of organs and tissues are bathed. This was a critical concept in physiology since it recognised the need of organisms to maintain an internal state protected from the variability and unpredictability of the external environment. The evolution of the ‘constancy of the internal milieu’ has been an essential factor that allowed animals to survive and thrive in changing environments.
The need to achieve a hospitable environment where cells and organs can perform in an optimal fashion was attributed to the continuous engagement at all levels – cells, organs, individuals – in active self-preservation. The concept of organisms adapting and coping with their environment was expanded and taken to a new dimension with the work of Cannon (Reference Cannon1929). He coined the term ‘homeostasis’ to refer to all the complex and coordinated physiological processes that maintain steady states in organisms. The term ‘homeostatic regulation’ was then created to define and describe all those physiological processes and mechanisms that keep the body ‘in balance’. Cannon argued that the body knows what is best – the wisdom of the body – and that organisms have ‘ancient biological knowledge’ embodied in their tissues (Schulkin, Reference Schulkin2001 and Reference Schulkin2005).
Behavioural homeostasis
Curt Richter extended Bernard’s ideas on the constancy of the internal milieu and Cannon’s views on homeostatic regulation by demonstrating the role of behaviour in the regulation of the internal environment (Schulkin, Reference Schulkin2005). Richter realised that the body adapts to external circumstances and internal needs not only by generating physiological responses that correct deviations from steady states, but also by generating behaviours. The central idea of behavioural homeostasis is that behaviours also evolved to serve physiological regulation, bringing the concept of homeostasis into the realm of behaviour and psychology (Schulkin, Reference Schulkin2005).
Richter’s research showed that adrenalectomised rats, experiencing severe depletions of Na, increased their intake and appetite for NaCl relative to control animals, behaviours that contributed substantially to their survival (Richter, Reference Richter1936). His groundbreaking findings on the relationships between behaviour and homeostasis led him to argue that specific appetites could be used as a measure of physiological deficiencies or nutritional needs in animals. In general, Richter assumed that the animals’ behavioural adjustments were innately programmed and guided by the sense of taste. In many aspects, he generalised his studies of sodium hunger to other specific hungers (Rozin, Reference Rozin1976).
The sodium model of nutritional wisdom was extrapolated to livestock by scientists attempting to explore ‘instinctive appetites’ for other nutrients. However, these efforts made researchers doubt that livestock possessed nutritional wisdom. Cattle and sheep did not instinctively ingest recommended levels of minerals, and many times animals’ under- or over-consumed mineral supplements relative to their requirements (Coppock, 1970; Coppock et al., Reference Coppock, Everett and Belyea1976; Pamp et al., Reference Pamp, Goodrich and Meiske1977).
The idea of animals instinctively searching for specific substances in the environment in order to rectify their needs has also influenced ecological theory where food selection has been explained through a ‘genetic programming of ingestive behaviour’ (Schmidt-Nielsen, Reference Schmidt-Nielsen1994). Evolution of foraging strategies that optimise nutrient intake (Belovsky, Reference Belovsky1978; Stephens and Krebs, Reference Stephens and Krebs1986) and fixed rules of thumb such as ‘eat tall green grass’ or ‘avoid bitter’ have also been proposed as mechanisms underlying diet selection in herbivores (Cassini, Reference Cassini1994). However, the ‘sodium model of nutritional wisdom’ is not a particularly good exemplar for energy, protein, minerals and vitamins because animals do not instinctively recognise through oduor/taste all of these nutrients, nor do they necessarily recognise all of the various configurations of any particular nutrient (Provenza and Balph, Reference Provenza and Balph1990; Provenza and Villalba, Reference Provenza and Villalba2006). Beyond the evolution of fixed codes or static rules of thumb, animals evolved learning mechanisms to cope with the frequent changes occurring in the internal and external environment. Learning is itself a genetically evolved mechanism, a kind of fixed plasticity that, like evolution, is adaptive (Skinner, Reference Skinner1984). Collectively, the information presented in this paper supports the notion that behavioural regulation is an important way individuals achieve homeostasis. However, needs are not fixed and neither are the behaviours underlying homeostatic regulation fixed or pre-programmed; they have a significant learned component. Behaviours are flexible and a function of their consequences (Skinner, Reference Skinner1981).
Feedback systems: a dynamic loop to learn based on consequences
Homeostatic regulation involves a mechanism of control with precise monitoring and effector systems such that the condition of the internal environment is regulated within rather narrow limits. Such a system of control can be understood as a feedback loop, or a circular arrangement of functionally connected links. These links interact until the last link in the loop feeds back to the first link in the cycle, resulting in self-regulation of the system. Feedback is a dynamic control system that influences behaviour through actual, rather than expected performance (Capra, Reference Capra1996). This monitoring-action loop is particularly relevant in living organisms because homeostasis is not static but inherently dynamic: set points, equilibrium states and biochemical processes change through time as organisms develop and preserve their integrity (Rose, Reference Rose1998). Thus, feedback cycles do more than simply maintain variables within normal limits; they increase the flexibility and adaptability of a system’s response (Provenza et al., Reference Provenza, Villalba, Cheney and Werner1998; Provenza and Villalba, Reference Provenza and Villalba2006).
The first link of a feedback system in the food selection process can be depicted as signals from nutrients and toxins that originate in the external environment and upon food ingestion impact cellular receptors in the animal (Figure 1). These signals are conducted through afferent nerves to a selector or decision-making organ – the central nervous system – where information is processed, integrated and where an output – a decision – is produced. The decision is conducted through efferent nerves to an effector organ, a muscle or group of muscles, which allows the animal to operate on the environment (Figure 1). As a function of the behaviour performed by the animal, a new signal will now interact with the receptor system. Thus, by the recursive application of these simple rules the system ‘rejuvenates’ itself and updates constantly, creating new decisions and fine-tuning new behaviours until homeostasis is achieved. This plasticity implies that as signals – in either the internal or external environment – change in the short (e.g. within a meal) or long (e.g. months) term, responses will track such changes, always with the same final goal: homeostatic regulation.
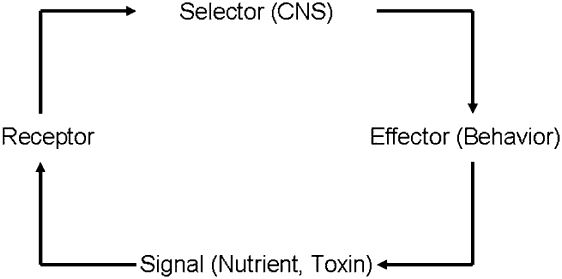
Figure 1 A feedback loop underlying behavioural homeostasis in herbivores. Feedback confers plasticity and adaptability to a system because control depends on actual conditions rather than on expected or predetermined performance. The recursive application of simple rules across each link of the loop rejuvenates and updates the system constantly, creating and fine-tuning behaviours until homeostasis is achieved.
Homeostatic behaviour and diet selection
Food preferences involve interactions between taste and postingestive feedback, which are determined by an animal’s physiological condition and the food’s chemical characteristics (Provenza, Reference Provenza1995 and Reference Provenza1996). The senses of smell and taste enable animals to discriminate among foods. Postingestive feedback calibrates sensory experiences of food – like or dislike – with its homeostatic utility. Thus, the receptor systems of the animal involves sensory receptors in the nose and mouth – that allow for food discrimination – and visceral receptors that respond to nutrients, toxins, osmosis and pressure, which inform the selector system of the animal (central nervous system) about the consequences of ingesting food (Figure 1) (Provenza, Reference Provenza1995 and Reference Provenza1996; Provenza and Villalba, Reference Provenza and Villalba2006)
Homeostatic behaviour can be observed in livestock fed diets that vary in nutrients. For instance, lambs and goats fed diets low in energy and protein prefer flavoured low-quality foods previously paired with intra-ruminal infusions of energy (starch, propionate, acetate) or nitrogen (urea, casein, gluten), respectively (Villalba and Provenza, Reference Villalba and Provenza1996, Reference Villalba and Provenza1997a, Reference Villalba and Provenzab and Reference Villalba and Provenzac; Arsenos et al., Reference Arsenos, Kyriazakis and Tolkamp2000; Duncan and Young, Reference Duncan and Young2002). Given pre-loads of energy or nitrogen, lambs prefer flavours previously paired with nitrogen or energy, respectively, during the ensuing meals (Villalba and Provenza, Reference Villalba and Provenza1999). Thus, animals maintain a balance of energy to protein that meets their nutritional needs, and in the process, they associate different internal states with the ingestion, or lack thereof, of different nutrients (Egan, Reference Egan1980; Kyriazakis and Oldham, Reference Kyriazakis and Oldham1993). Likewise, lambs deficient in phosphorus and calcium increase preferences for flavours and supplements that provide those minerals (Villalba et al., Reference Villalba, Provenza, Hall and Peterson2006a; J. Villalba, unpublished results).
Homeostatic behaviour can be also observed when animals are offered diets with deficits or excesses of nutrients or excesses of toxins that deviate cells and organs from well-being and self-preservation. In this case, behaviours are geared towards limiting food intake. For instance, sheep are reluctant to eat poorly nutritious foods such as straw as straw does not meet needs for energy or protein, but their intake and preference for straw increase when a nutritious food (Greenhalgh and Reid, Reference Greenhalgh and Reid1971) or starch (Villalba and Provenza, Reference Villalba and Provenza1997a and Reference Villalba and Provenza2000) is infused into the rumen immediately after a meal of straw. Ruminants also avoid flavours associated with excesses of nitrogen (Kyriazakis and Oldham, Reference Kyriazakis and Oldham1993; Villalba and Provenza, Reference Villalba and Provenza1997a) or excesses of volatile fatty acids (Villalba and Provenza, Reference Villalba and Provenza1997b) and limit intakes of otherwise nutritious foods that contain plant secondary metabolites (PSMs) (Provenza et al., Reference Provenza, Burritt, Clausen, Bryant, Reichardt and Distel1990; Provenza, Reference Provenza1996; Dearing et al., Reference Dearing, Foley and McLean2005).
Self-medication
If herbivores can evolve mechanisms to maintain homeostasis and contemporarily learn to avoid certain foods because they lower their fitness, or prefer flavours associated with nutrients because they increase their fitness, they may also learn to ingest other substances in the environment such as medicines as they also raise fitness (Janzen, Reference Janzen1978). Just as foraging behaviour is influenced by nutrients and PSMs, some responses may also be geared towards reducing disease (Lozano, Reference Lozano1998).
Our thesis is that food selection in herbivores can be interpreted as the constant quest for substances in the external environment that provide a homeostatic benefit to the internal environment (Provenza and Villalba, Reference Provenza and Villalba2006). Under this view, nutrients, PSMs, medicines are all ‘substances’ with the same final utility: improving the welfare of cells and cellular processes that enable life. Thus, self-medication – as ingestion of nutrients and avoidance of PSMs – is another dimension of homeostatic behaviour in animals. Ingesting nutrients and medicines are means to the same end – stay well (Engel, Reference Engel2002).
From prehistoric times, man has looked to the presumed self-medicative behaviour of animals for clues about remedies for ailments (Huffman, Reference Huffman2003). Implications of self-medication transcend animal species and range from providing shortcuts for discovering new medicines (Clayton and Wolfe, Reference Clayton and Wolfe1993) to managing the well-being of wild and domestic animals (Lozano, Reference Lozano1998) and improving human health (Engel, Reference Engel2002).
The study of self-medication in animals has led to the emergence of a new field, often referred to as ‘zoopharmacognosy’ to describe the process by which animals select and use PSMs or other non-nutritional substances for treating and preventing disease (Rodriguez and Wrangham, Reference Rodriguez and Wrangham1993). While little is known about the abilities of animals to self-medicate, and many of the observations are anecdotal and equivocal (Clayton and Wolfe, Reference Clayton and Wolfe1993; Lozano, Reference Lozano1998; Houston et al., Reference Houston, Gilardi and Hall2001), there is evidence animals self-medicate (Engel, Reference Engel2002). Researchers who contend that animals self-medicate under natural conditions do so based on observations that sick animals seek substances – not part of their normal diet and preferably with no nutritional benefit – that contain active ingredients capable of improving health (Ketch et al., Reference Ketch, Malloch, Mahaney and Huffman2001; Engel, Reference Engel2002; Huffman, Reference Huffman2003). Such correlative studies are consistent with the self-medication hypothesis, but they do not establish cause and effect (Lozano, Reference Lozano1998).
The amount of detailed information on self-medication in animals available thus far is greatest in primates, in particular the African great apes (Huffman, Reference Huffman2003). The hypothesis from research on these animals is that self-medication behaviour aids in the control of internal parasites and provides relief from gastro-intestinal upset (Huffman, Reference Huffman2003; Huffman et al., Reference Huffman, Gotoh, Izutso, Koshimizu and Kalunde1993). Studies of apes show correlations between the occurrence of parasite loads and ingestion of anti-parasitic plant secondary compounds or plant parts that enhance mechanical expulsion (Huffman, Reference Huffman2001; Huffman and Caton, Reference Huffman and Caton2001).
One of the best examples of self-medication comes from observations that wild chimpanzees consume the bitter pith of the plant Vernonia amygdalina when suffering from parasite-related diseases even when V. amygdalina is not part of the animals’ diet, despite its year-round availability. Parasite loads decrease dramatically after animals consume that plant, and overt signs of disease disappear (Huffman and Seifu, Reference Huffman and Seifu1989; Huffman et al., Reference Huffman, Gotoh, Izutso, Koshimizu and Kalunde1993). V. amygdalina contains sesquiterpene lactones and steroid glucosides with antiparasitic activity at the doses consumed by the animals (Koshimizu et al., Reference Koshimizu, Ohigashi and Huffman1994; Ohigashi et al., Reference Ohigashi, Huffman, Izutsu, Koshimizu, Kawanaka, Sugiyama, Kirby, Warhurst, Allen, Wright, Phillipson, Timmon-David, Delmas, Elias and Balansard1994). PSMs in V. amygdalina also have been effective at controlling nematodes that cause significant losses of livestock in the tropics (Plotkin, Reference Plotkin2000). Other plants selected by chimpanzees have medicinal effects in the amounts (doses) consumed: limonoids in Trichilia rubescens have antimalarial activity (Krief et al., Reference Krief, Martin, Grellier, Kasenene and Sevenet2004), and methoxypsoralen in Ficus exasperata is a strong antibiotic (Rodriguez and Wrangham, Reference Rodriguez and Wrangham1993).
Beyond the African great apes: self-medication in livestock and other animals
The most attractive and conspicuous animals, and those thought to be most similar to humans, have received the greatest attention regarding self-medication (e.g. African great apes; Huffman, Reference Huffman2003). However, evidence of self-medication in primates may well represent an artifact of collection in as much as intensive research in a reduced number of species may suggest other species are unable to self-medicate (Plotkin, Reference Plotkin2000). Nevertheless, there is evidence of self-medication in other animals including bears, geese, leopards and dogs (Huffman, Reference Huffman1997 and Reference Huffman2006). Asian two-horned rhinos consume large amounts of tannin-rich plants with antiparasitic properties (Janzen, Reference Janzen1978). Peruvian parrots prefer soils with much higher cation-exchange capacity than adjacent bands of rejected soils. Selected soils contain kaolin and mica, which bind PSMs such as alkaloids and tannic acid (Gilardi et al., Reference Gilardi, Duffey, Munn and Tell1999), allowing for increased diet breadth and digestibility. Likewise, some propose soil (35% kaolin) consumption by African elephants assists in the digestion of browse by detoxifying high concentrations of PSMs in tropical forest trees (Houston et al., Reference Houston, Gilardi and Hall2001).
Sheep learn to ingest medicines such as polyethylene glycol (PEG), a substance that attenuates the aversive effects of tannins, when they eat foods high in tannins, and they titrate the dose of PEG in accord with the amount of tannin in their diet (Provenza et al., Reference Provenza, Burritt, Perevolotsky and Silanikove2000). They discriminate the medicinal benefits of PEG from non-medicinal substances by selectively ingesting PEG after eating a meal high in tannins (Villalba and Provenza, Reference Villalba and Provenza2001). Sheep also choose to forage in locations where PEG is present when offered nutritious foods high in tannins in different locations. In contrast, time spent at locations with PEG declined when tannins were not present in their diets (Villalba and Provenza, Reference Villalba and Provenza2002). Sheep fed acid-producing substrates such as grains subsequently ingest foods and solutions that contain sodium bicarbonate, which attenuates acidosis (Phy and Provenza, Reference Phy and Provenza1998). In the most elaborate studies to date, sheep learned to selectively ingest three medicines – sodium bentonite, PEG, dicalcium phosphate – that lead to recovery from illness due to eating too high amounts of grain, tannins and oxalic acid, respectively (Villalba et al., Reference Villalba, Provenza and Shaw2006b). In contrast, control lambs that ate the same foods and medicines, but disassociated temporally so they did not recuperate from illness, never changed their pattern of use of the medicines, regardless of the food consumed before the choice of medicines. This study showed learning is a critical mechanism in self-medication and that sheep are able to form multiple malaise-medicine associations (Villalba et al., Reference Villalba, Provenza and Shaw2006b).
Self-medication and plant secondary metabolites
We have learned much during the past 30 years about the importance of PSMs in the health of plants, including functions as diverse as attracting pollinators and seed dispersers, helping plants recover from injury, protecting plants from ultraviolet radiation and defending plants against diseases, pathogens and herbivores (Rosenthal and Janzen, Reference Rosenthal and Janzen1979; Palo and Robbins, Reference Palo and Robbins1991). PSMs cause postingestive consequences in herbivores typically through their negative actions on several cellular and metabolic processes, promoting reduced intake, weight loss and even death (Cheeke and Scull, Reference Cheeke and Shull1985; Cheeke, Reference Cheeke1998). Consequently, PSMs can exert some of those negative actions across several trophic levels, including herbivores and the bacteria, parasites and fungi that inhabit herbivores’ bodies and cause decreases in health (Lozano, Reference Lozano1998). What has not been seriously considered in the ecological literature is that the difference between a toxin and a medicine can be very small, merely a matter of dosage (Plotkin, Reference Plotkin2000).
Plant-derived alkaloids, terpenes and phenolics have antiparasitic properties (Kayser et al., Reference Kayser, Kiderlen and Croft2003; Hocquemiller et al., Reference Hocquemiller, Cortes, Arango, Myint, Cave, Angelo, Munoz and Fournet1991) and sesquiterpene lactones have anti-tumorigenic, anti-amoebic, anti-bacterial, anti-fungal and cardiotonic properties (Picman, Reference Picman1986; Robles et al., Reference Robles, Arguellin, West and Rodriguez1995; Huffman et al., Reference Huffman, Ohigashi, Kawanaka, Page, Kirby, Gasquet, Murakami and Koshimizu1998). Thus, PSMs at appropriate doses have the potential to benefit herbivores in ways that may be more consequential than their negative effects. Nevertheless, the notion of herbivores using PSMs as medicines has been overshadowed by the inherent negative effects of PSMs at high doses on herbivores. Another constraint on using PSMs as medicines is the potential large quantities of a single PSM such as tannins required to achieve meaningful doses in the herbivore in order to combat disease (Waghorn and McNabb, Reference Waghorn and McNabb2003).
Self-medication and internal parasites
Internal parasites are one of the greatest disease problems in grazing livestock worldwide (Min and Hart, Reference Min and Hart2003; Waller, Reference Waller2006). Parasitism is often the most pervasive challenge to host survival and reproduction (Hutchings et al., Reference Hutchings, Athanasiadou, Kyriazakis and Gordon2003) and failure to control gastro-intestinal nematodes results in poor growth rates, ill thrift and death (Min et al., Reference Min, Pomroy, Hart and Sahlu2004). Nevertheless, control of internal parasites is not easy, particularly in recent times due to the rise of drug-resistant organisms (Plotkin, Reference Plotkin2000; Jackson and Miller, Reference Jackson and Miller2006). Drugs used to control gastro-intestinal parasites are failing due to the increased prevalence of parasite resistance to anthelmintics (Pomroy et al., Reference Pomroy, Hart and Min2002; Min et al., Reference Min, Pomroy, Hart and Sahlu2004). Drug resistance is testimony to the remarkable biological plasticity of nematode parasites in response to a sustained and almost exclusive reliance on specific chemicals for disease control (Waller, Reference Waller2006). More effective and sustainable programmes for combating parasites may be possible if more choices and the greater variety of controls, including anthelmintics, are used in combinations, along with grazing management designed to minimise parasite infections (Waller, Reference Waller2006). Avoidance of dung patches and rotation of animals in landscapes probably occurred naturally, prior to the use of fencing in grazing management. Clearly, programmes that integrate grazing management and biological control, in addition to anthelmintics, are best for reducing parasites and minimising harmful effects of drugs on the functioning of soils (Strong, Reference Strong1993; Floate et al., Reference Floate, Colwell and Fox2002).
Considerable attention has been given recently to bioactive plants that affect internal parasite populations (Jackson and Miller, Reference Jackson and Miller2006). Several in vitro and in vivo studies suggest condensed tannins have anthelmintic effects against ruminant nematode parasites. Livestock feeding on plants with tannins such as sulla (Hedysarum corarium) and sericea lespedeza (Lespedeza cuneata) have lower nematode burdens and lower faecal egg counts than those eating plants of similar quality without tannins (Niezen et al., Reference Niezen, Robertson, Waghorn and Charleston1998; Coop and Kyriazakis, Reference Coop and Kyriazakis2001; Min and Hart, Reference Min and Hart2003; Min et al., Reference Min, Pomroy, Hart and Sahlu2004). Chicory (Cichorium intybus) contains an array of condensed tannins and other phenolic compounds including sesquiterpene lactones, coumarin and caffeic acid derivatives that reduce the need for commercial anthelmintics in young farmed deer (Hoskin et al., Reference Hoskin, Barry, Wilson, Charleston and Hodgson1999).
The effectiveness of certain PSMs at combating disease has enhanced the interest in continuing the search for plants with therapeutic properties. The quest for bioactive PSMs in nature i.e. bioprospecting is the means that leads to two different ends: (1) the industrial approach, where the quest for bioactives has the main objective of creating new drugs and additives to combat disease and (2) the holistic and more sustainable approach where a variety of plants rich in PSMs or other bioactives are considered and incorporated into grazing systems for disease control (see also Athanasiadou and Kyriazakis, Reference Athanasiadou and Kyriazakis2004). We believe that self-medication has its highest potential for improving animal health and welfare under the sustainable approach.
If parasitised herbivores learn to self-medicate, given a variety of plant species with a variety of PSMs, producers may not need to give fixed or average dosages of chemicals to all the animals of a herd, likely with different parasite burdens, and it may not be necessary to force parasited animals to graze monocultures of PSM-rich pastures, which may lead to overconsumption of PSMs and to negative impacts on health and welfare. On the contrary, forage mixes could be sown to enhance the antiparasitic and nutritional characteristics of the forage on offer. If parasited animals learn to self-medicate on PSM-containing plants, this could aid in developing management programmes geared to seeding and distributing ‘medicinal’ plant species strategically in the environment, allowing herbivores to combat disease by themselves.
Use of anthelmintics as a function of need via self-medication is a targeted treatment that reduces usage and helps maintain populations in refugia, which in turn aids in conserving the genes for susceptibility within parasite populations (Jackson and Miller, Reference Jackson and Miller2006). In fact, minimising treatment to periods of peak infection, rotating or combining different chemotherapies and proper dosage are fundamental principles to control for parasite chemoresistance in livestock and human treatment programmes (Geertz et al., Reference Geertz, Coles and Gryseels1997). Self-medicative behaviour in the African great apes appears to mirror these principles and may represent a stable evolutionary strategy for parasite control (Huffman et al., Reference Huffman, Ohigashi, Kawanaka, Page, Kirby, Gasquet, Murakami and Koshimizu1998). Thus, research on self-medication may provide novel insights into strategies for countering the increased prevalence of parasite resistance to anthelmintics (Huffman et al., Reference Huffman, Ohigashi, Kawanaka, Page, Kirby, Gasquet, Murakami and Koshimizu1998). Moreover, self-medication could be used in combination with other sustainable disease prevention and treatment practices such as nutritional and biological control and grazing management (Waller, Reference Waller2006), and when necessary, with the conventional approach of drug application.
Even when self-medication may represent an effective and alternative tool for disease control, there is no evidence that livestock self-medicate to control infectious diseases (e.g. helminthoses) and information on livestock self-medicating in general is limited. Thus, innovative and multidisciplinary research is needed to further explore the limits and variables of this behaviour in domestic animal species. This will help strengthen the current correlational evidence on self-medication and infectious diseases, providing a starting point for developing parasite control strategies in livestock systems.
The fundamental dichotomy in self-medicating with PSMs: benefits v. costs
Overcoming the negative impacts of PSMs to experience their benefits
The anti-parasitic, anti-fungal and anti-bacterial characteristics of PSMs come with a cost. This is because some of the negative impacts of PSMs on the cells and physiological processes of the disease-inducing agents also can adversely affect the host. Thus, the potential benefits associated with consuming PSMs by sick animals must be traded-off against the potentially negative consequences of PSMs on cells and physiological processes of the host (Hutchings et al., Reference Hutchings, Athanasiadou, Kyriazakis and Gordon2003).
The positive (medicine) and negative (toxic) effects of PSMs must be evaluated under the context of a common measure to determine the net positive or negative outcome of PSMs on herbivores. Animal performance emerges as an interesting possibility in this regard as both disease and PSM consumption affect performance (Athanasiadou and Kyriazakis, Reference Athanasiadou and Kyriazakis2004). Athanasiadou and Kyriazakis (Reference Athanasiadou and Kyriazakis2004) propose three scenarios regarding the costs on herbivore performance from ingesting PSMs: (1) the negative impacts of PSMs overshadow their medicinal benefits; (2) the benefits of PSMs overshadow their anti-nutritional properties; and (3) the negative effects of PSMs offset their potential medicinal benefits. Beyond production costs and benefits, the crucial question for understanding self-medication with PSMs is: can sick herbivores associate a potential medicinal effect with PSMs they might otherwise avoid (Engel, Reference Engel2002; Hutchings et al., Reference Hutchings, Athanasiadou, Kyriazakis and Gordon2003) and under what circumstances will herbivores ingest PSMs to experience the benefits?
Typically, PSMs decrease preference for food, a mechanism that prevents herbivores from exceeding their capacity to biotransform and eliminate these compounds, which in turn prevents toxicosis (Provenza, Reference Provenza1996). Aversions result from the stimulation of the emetic system of the mid-brain and brain stem (Mitchelson, Reference Mitchelson1992). Stimulation of this system induces malaise, and subsequently causes the animal to decrease intake of food (Provenza, Reference Provenza1996). Based on this mechanism we can depict a cost–benefit scenario within the realm of self-medication (Figure 2).
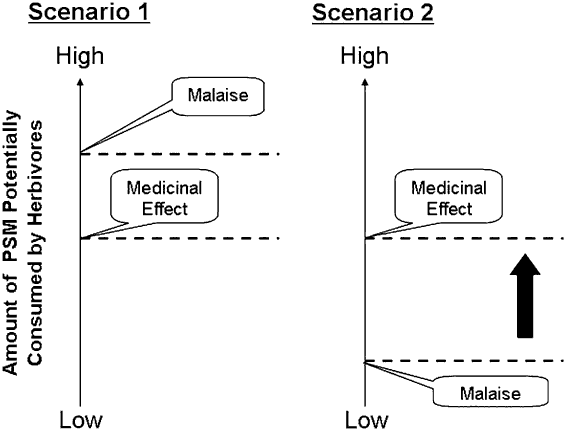
Figure 2 Two potential scenarios affecting the likelihood of learning about the beneficial effects of plant secondary metabolites (PSMs). In the first scenario, the dose needed for PSMs to have a medicinal effect requires that the animal ingest amounts of PSMs that are below those that cause malaise and stimulation of the emetic centre. In this scenario, the likelihood of animals being willing to consume therapeutical amounts of PSMs will be high. In contrast, in the second scenario the dose needed for PSMs to have a medicinal effect requires that the animal eats amounts of PSMs which are above those that cause malaise and stimulation of the emetic centre. This hierarchy of malaise and medicinal effects may prevent herbivores from learning about the beneficial effects of PSMs.
If the amount of PSM required to induce a therapeutic effect in herbivores is below the threshold amount that causes malaise and stimulates the emetic system, then the likelihood of animals consuming such amounts to self-medicate and combat disease will increase. In turn, ingestion of PSMs will give sick herbivores the opportunity to experience the therapeutic effects of PSMs, which may lead to a stronger preference for such compounds. In contrast, if the doses of PSM required to promote a medicinal effect are equal or above the threshold amount that stimulates the emetic system, then the likelihood of animals crossing such a threshold will diminish (Figure 2). Low or no therapeutic consumption of PSMs will deprive animals from experiencing the potential medicinal benefits of PSMs. However, if animals are forced to cross the ‘malaise threshold’ and experience the benefits of consuming PSMs, will they subsequently select PSMs to combat disease when they are not forced to do so? Will they subsequently cross the ‘malaise threshold’ by themselves?
Previous research suggests they might. Lambs in a group (experienced) were forced to consume foods with tannins, terpenes and oxalates. These foods are complementary as the combined intake of the three foods is comparable with the intake of food without PSMs (Villalba et al., Reference Villalba, Provenza and Han2004). A second group of lambs (naïve) was not forced to consume the PSM-containing foods. Subsequently, when these foods were offered in a choice, lambs with experience ate significantly more of the foods containing the PSMs, up to 40% of their diet, even when safe and nutritious foods (alfalfa, barley) were available ad libitum. These differences in food preferences and intake persisted during trials a year later (Villalba et al., Reference Villalba, Provenza and Han2004).
Up to this point, we have considered animals ingesting large doses of PSMs to obtain medicinal benefits, but animals may not need to ingest PSMs in amounts that stimulate the emetic system. Consuming small amounts of a variety of PSMs, below therapeutic doses but on a daily basis, may have a cumulative effect that eventually will negatively impact disease-inducing organisms such as parasites (F. Jackson, personal communication). Preliminary results in our laboratory suggest low amounts of quebracho tannin consumed by lambs on a daily basis have negative impacts on nematode faecal egg counts (L. Lisonbee et al., unpublished results).
Some propose herbivores have evolved specific changes in diet selection in response to parasitism (Kyriazakis et al., Reference Kyriazakis, Tolkamp and Hutchings1998), which may facilitate exposure and familiarity with PSMs in parasitised animals. Behaviour is a function of need (Provenza et al., Reference Provenza, Villalba, Dziba, Atwood and Banner2003) and by sampling a variety of bitter substances an animal may increase the probability of finding an active compound that supplies a medicinal benefit (Freeland and Janzen, Reference Freeland and Janzen1974; Glendinning, Reference Glendinning1994). Given the chemoprophylactic effects of bitter-tasting substances such as tannins and sesquiterpene lactones discussed above, it is conceivable that herbivores benefit from consuming low doses of such compounds when sick. In support of this, parasitised sheep begin to sample novel low-quality foods with quebracho tannin more readily than non-parasitised animals (L. Lisonbee et al., unpublished results). Repeated sampling of a bitter-tasting antimalarial agent (chloroquine) by malaria-infected mice significantly reduced percentages of parasitemia and the risk of mortality (Vitazkova et al., Reference Vitazkova, Long, Paul and Glendinning2001). More recently, a mechanism for increasing preference for PSMs when organisms are under parasitic burdens has been described. Infection by parasites alters the taste response for specific PSMs (pyrrolizidine alkaloids) in caterpillars, and in so doing, encourages PSM ingestion, providing a biochemical defence (Bernays and Singer, Reference Bernays and Singer2005). Finally, social models are likely to be another way herbivores are enticed to cross the ‘malaise threshold’ and become exposed to the beneficial effects of PSMs (see below).
Self-medicating in chemically diverse environments: an alternative for reducing costs and enhancing benefits
In studies where production in parasitised animals has been recorded, performance remained the same or was impaired by the addition of PSMs to supplements of feeds. No net benefit in terms of production has been found (Athanasiadou and Kyriazakis, Reference Athanasiadou and Kyriazakis2004). However, the majority of such studies have forced animals to consume PSMs and only a single PSM has been offered, much as conventional approaches use anthelmintic drugs.
Historically, we have emphasised monocultures of plants on pastures and even on rangelands (Provenza et al., 2007). We are just beginning to appreciate the importance of PSMs and biodiversity in the foraging behaviour of herbivores (Provenza and Villalba, Reference Provenza and Villalba2006; Provenza et al., 2007). We believe creating mixtures of plants whose PSM profiles complement one another and allowing animals to self-medicate using a diverse array of PSMs is the way to reduce the negative impacts of PSMs on animal production and to enhance animal health through the medicinal effects of PSMs.
All plants contain PSMs that limit the amount of a particular plant a herbivore can eat. Herbivores are able to meet their needs for nutrients by ingesting a variety of plants with different PSMs that complement one another (Freeland and Janzen, Reference Freeland and Janzen1974; Provenza et al., Reference Provenza, Villalba, Dziba, Atwood and Banner2003). Large doses of one PSM overload specific detoxification mechanisms in herbivores. Thus, PSMs that affect different organs or detoxification pathways are likely to be less toxic as a diluted mixture than as a larger dose of one PSM (Freeland and Janzen, Reference Freeland and Janzen1974). Indeed, sheep eat more when offered choices of foods with various PSMs that affect different detoxification mechanisms, and thus are complementary (Burritt and Provenza, Reference Burritt and Provenza2000; Villalba et al., Reference Villalba, Provenza and Han2004).
If animals can consume a higher total amount of PSMs that are detoxified by different detoxification pathways, then it is likely organisms inhabiting a host will receive a greater total dose of PSMs, making the effectiveness of the treatment greater. Thus, by providing a variety of plants with different PSM profiles, herbivores may be able to meet needs for nutrients and combat disease simultaneously.
Both bacterial resistance to drugs and the developing anthelmintic resistance to drugs show how strains of bacteria and parasites can quickly evolve to survive any one type of selection pressure (e.g. specific antibiotics and anthelmintics). Thus, they are also perfectly capable of doing the same with other chemicals (Waller, Reference Waller2006), just as treatment with a single PSM such as tannins. A diversity of PSMs might provide the variability needed to prevent or diminish the development of resistance.
Eating nutrients or PSMs?
Given the negative impacts of PSMs and the positive effects of nutrients on a herbivore’s physiology, a superficial analysis of the rules that guide foraging behaviour may lead to the conclusion that animals will strongly avoid PSMs and select nutrient-rich forages. However, not only do herbivores manifest partial preferences (Westoby, Reference Westoby1978), they also ingest substantial amounts of PSMs (Provenza, Reference Provenza1995 and Reference Provenza1996; Villalba et al., Reference Villalba, Provenza and Han2004), likely due in part to their health and medicinal effects. If we view nutrients from another angle, they can also enhance resilience and resistance of the host to disease (Coop and Kyriazakis, Reference Coop and Kyriazakis1999 and Reference Coop and Kyriazakis2001). For instance, protein intake increases the resilience of livestock to parasitic infection by increasing host productivity, and when protein bound to tannins bypasses the rumen and is subsequently digested in the small intestine, that protein enhances immunity to nematode infections (Coop and Kyriazakis, Reference Coop and Kyriazakis2001; Ketzis et al., Reference Ketzis, Vercruysse, Stromberg, Larsen, Athanasiadoum and Houdijk2006). Thus, both nutrients and PSMs can supply ‘health and medicinal’ benefits to herbivores. The question is how grazing animals in their homeostatic quest for substances in their external environment can best harvest both the benefits of nutrients and PSMs, such that they achieve optimal nutrition and enhanced protection against disease.
A useful approach to dealing with the complexity of interactions among nutrients and PSMs in food selection is the ‘geometric framework’ or the state-space graphical representation of the animal within its chemical environment (Simpson and Raubenheimer, Reference Simpson and Raubenheimer1999; Raubenheimer and Simpson, Reference Raubenheimer and Simpson1997). A Cartesian coordinate system defines a ‘chemical space’ where each coordinate represents a specific chemical, such as a nutrient or a PSM. In this synthesis, there is a point in chemical space or a ‘target’ that represents the optimal intake of the chemicals in question relative to the animal’s physiological state. The rationale of this system is rooted in behavioural homeostasis: When herbivores are offered choices among foods with different concentrations of nutrients and PSMs with medicinal properties, their selection should reflect the outcome of homeostatic regulation for the chemicals in question.
The trade-off between toxicity-nutrition-medicinal effects can be depicted in a two-dimensional space, where one dimension is a nutrient (‘nutrient axis’) and the other a PSM that provides medicinal effects (‘PSM axis’). Each food available for consumption is represented in that space by a line or ‘rail’ as it contains a specific proportion of nutrients and PSMs. For instance, in Figure 3 the OA line represents a food high in PSMs and low in nutrients (e.g. medicinal food), whereas the OB line represents a food low in PSMs and high in nutrients (e.g. nutritive food). A point in chemical space represents the hypothetical choice made by animals when offered these two foods. The point closer to the nutritive food (‘c’; Figure 3) depicts a choice where the animal is not willing to consume high amounts of PSMs and thus prefers the nutritive food. On the other extreme, the point closer to the medicinal food (‘d’; Figure 3) shows a choice where the animal consumes higher amounts of PSMs (i.e., is willing to ‘take its medicine’) to the detriment of its nutrition. Animals performing the former choice will consume their required nutrients but not enough PSMs, which may not be optimal if the animal is sick and the medicine is highly effective at combating disease. In contrast, the latter choice will provide doses for medicinal effects but not enough nutrients.
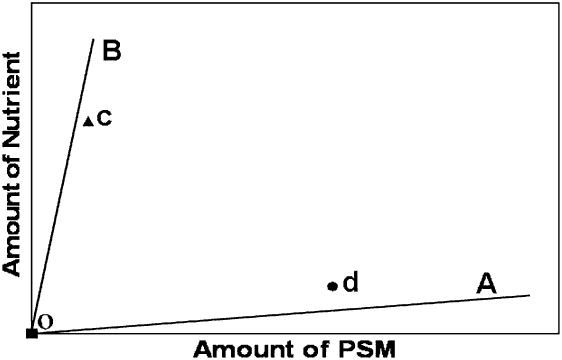
Figure 3 Schematic illustration of nutrient and plant secondary metabolite (PSM) regulation in a two-dimensional space. The line OA represents a food high in PSMs and low in nutrients (medicinal food), whereas the line OB represents a food low in PSMs and high in nutrients (nutritive food). The depicted points in space represent two possible outcomes of a selection made by a hypothetical animal when the two foods are available for consumption. The point ‘c’ represents a decision where the animal is not willing to consume high amounts of PSMs and thus selects almost exclusively the food high in nutrients. In contrast, the point ‘d’ represents a decision where the animal consumes substantial amounts of PSMs but low amounts of nutrients.
The challenge is for the animal to determine – once animals are trained to experience the beneficial effects of PSMs and nutrients from the foods on offer – the optimal point in chemical space to maintain homeostasis by meeting needs for nutrients and PSMs. For instance, animals with a parasitic burden may be willing to consume more pastures with bioactive PSMs that enhance health than non-parasitised animals. Parasitised animals may increase their grazing time to include medicinal plants in their diets. This analysis, beyond the theoretical implications for the interactions of nutrient-medicine, may guide the design of grazing systems where nutritive and medicinal plants are sown to deliver optimal proportions of nutrients and bioactive PSMs for animal nutrition and health.
Trans-generational transmission of self-medication
Social organisation creates culture, the knowledge and habits acquired by ancestors and passed from one generation to the next about how to survive in an environment (De Waal, Reference De Waal2001). Cultures develop when learned practices contribute to the group’s success in solving problems. Cultures evolve as individuals in groups discover new ways of behaving, as with finding new foods or habitats and better ways to use foods and habitats (Skinner, Reference Skinner1981). The transition from the unfamiliar to the familiar begins at conception and in utero with mother and continues after birth with mother and peers. Lessons learned early in life from a mother create a dichotomy between the familiar and the unfamiliar (novel), which is essential for survival (Provenza, Reference Provenza1995; Provenza et al., Reference Provenza, Villalba, Cheney and Werner1998 and Reference Provenza, Villalba, Dziba, Atwood and Banner2003). Animals prefer familiar to novel foods and environments and they prefer to be with companions as opposed to strangers (Provenza et al., Reference Provenza, Villalba, Cheney and Werner1998). Critically, animals are at the same time cautious and curious about the unfamiliar. Maintaining the appropriate balance between the two is critical for survival.
Socialising enhances learning efficiency because each animal no longer has to discover everything through trial and error. It is difficult for animals to learn through trial and error about the medicinal effects of substances, especially if behaviour and consequences (flavour-feedback) are not contingent (paired consistently) and contiguous (paired closely in time). Naïve animals familiar with only a limited number of foods in a landscape, or familiar with many foods eaten in the wrong sequence, may be less likely to learn about the potential medicinal values of foods available in their environment. Thus, ‘pioneering’ animals in a social group – those that learn exclusively based on consequences – must be positioned in the ‘right place and at the right time’. Once an individual ingests the ‘right foods at the right time’ the beneficial effects of the new behaviour may then spread through the group, becoming part of the foraging behaviour of females (Huffman, Reference Huffman2001; Huffman and Hirata, Reference Huffman and Hirata2004), who can then transmit those behaviours to their offspring.
Such transmission of information across generations occurs in livestock. For instance, when offered a choice, lambs avoid or prefer foods as a function of their mothers’ avoidances or preferences (Mirza and Provenza, Reference Mirza and Provenza1990 and Reference Mirza and Provenza1992). When a mother’s food selection behaviour (eat or avoid) is subsequently reinforced by postingestive feedback from nutrients or toxins (positive or negative), her offsprings respond strongly (eat or avoid) to a food (Provenza et al., Reference Provenza, Lynch and Nolan1993). Such knowledge then becomes a part of the culture, wherein young animals learn from their ancestors through their mothers. As mentioned above, mother and peers may facilitate exposure to PSMs in naïve animals, increasing the likelihood of experiencing the beneficial effects of PSMs, even when they have to be ingested at doses that cause stimulation of the emetic system.
Conclusions and implications
There is an increasing need to create a sustainable agriculture, with less dependence on external finite resources, such as fossil fuels and their environmentally detrimental derivatives. From the consumer perspective, there is also an increasing demand for products that are both ‘clean’ and ‘green’ (Waller, Reference Waller2006).
Natural landscapes with diverse mixtures of plant species are literally nutrition centres and pharmacies with vast arrays of primary (nutrient) and secondary (pharmaceutical) compounds vital in the nutrition and health of plants and herbivores. Regrettably, the simplification of agricultural systems to accommodate inexpensive, rapid livestock production, coupled with a view of secondary compounds as toxins, has resulted in selecting for a biochemical balance in forages favouring primary (mainly energy) and nearly eliminating secondary compounds. Ironically, while we were minimising secondary compounds to maximise yields of crop and pasture plants over the past 30 years, we were learning of their value in plant resistance to environmental stressors, and we are now beginning to appreciate their nutritional and pharmaceutical values for herbivores and people. In their stead, we resorted to fertilisers, herbicides and insecticides to grow and protect plants in monocultures, and antibiotics and anthelmintics to maintain the health of herbivores.
Most contemporary agricultural systems have also viewed animals as ‘machines’ whose fuel – needed nutrients and medicines – had to be provided at prescribed times and known amounts. While more research is required, evidence presented in this paper suggests herbivores can meet their needs for nutrients and ‘write their own prescriptions’. If so, self-medication by herbivores can contribute to sustainable grazing systems with benefits to the environment and to the animal’s health and welfare.
Viewing foraging behaviour as the quest for substances in the external environment that provide homeostatic utility to the ‘internal milieu’ leads us to the conclusion that self-medication is the consequence of the same mechanism that allows animals to form preferences for nutrients (Villalba and Provenza, Reference Villalba and Provenza1996, Reference Villalba and Provenza1997a, Reference Villalba and Provenzab, and Reference Villalba and Provenzac, Reference Villalba and Provenza1999) and develop aversions to toxins (Provenza et al., Reference Provenza, Burritt, Clausen, Bryant, Reichardt and Distel1990): Behaviour by consequences. Thus, while nutrients, PSMs and medicines are labels scientists use to better comprehend foraging complexity, for the animal in a dynamic homeostatic quest, ingesting nutrients, PSMs and medicines are means to the same end – staying well.
Acknowledgements
This effort was supported by grants from the Utah Agricultural Experiment Station and the Initiative for the Future of Agriculture and Food Systems, USDA. This paper is published with the approval of the Director, Utah Agricultural Experiment Station, and Utah State University, as journal paper number 7852. We thank A. Duncan and two anonymous reviewers for valuable comments to improve the manuscript.