Implications
This study focuses on the role of animal production and on the main challenges related to the content of essential and non-essential heavy metals in animal feed and manure as a basis for developing effective approaches to the reduction of heavy metal pollution from livestock.
Introduction
Metals are natural constituents of the earth’s crust and through natural erosion due to water and wind, they are naturally spread into the environment as powders or leached into rivers. However, these natural processes emit fewer metals into the environment than anthropological activities. The spread of high amounts of these elements in the environment leads to their propagation in the food chain. Heavy metals (e.g. Fe, Co, Cu, Mn, Mo, Se, Zn, Cr and Cd, Hg, Pb, As) are metallic elements that have a high density compared with water and are present in various matrices in traces. Their heaviness and toxicity are interrelated, as heavy metals are able to induce toxicity at low doses (Bhargava et al., Reference Bhargava, Carmona, Bhargava and Srivastava2012; Govind and Madhuri, Reference Govind and Madhuri2014; Dai et al., Reference Dai, Jones, Lee, Li, Post and Herrman2016; Giromini et al., Reference Giromini, Rebucci, Fusi, Rossi, Saccone and Baldi2016).
Some metals are essential to maintain various biochemical and physiological functions in humans, animals and plants. The nutritional requirements of these trace elements, such as cobalt (Co), copper (Cu), chromium (Cr), iron (Fe), manganese (Mn), molybdenum (Mo), selenium (Se) and zinc (Zn) are generally low and they are called microelements. They are present in various matrices, although with a different bioavailability, in trace concentrations (ppb or ppm) (Hambidge, Reference Hambidge2003). Essential trace elements are usually added as nutritional additives in animal feed to promote health, and to optimize production (European Union (EU) Reg. 1881/2006). However, excessive exposure with higher concentration of these elements has been linked with cellular or systemic disorders and could represent a source of pollution (Rossi et al., Reference Rossi, Fusi, Boglioni, Giromini, Rebucci and Baldi2014b).
Other metals (e.g. As, Cd, Pb, Hg) have no established biological functions and are considered as contaminants and undesirable substances in animal feed (Reg. 2002/32/EC). Moreover, As, Cd, Cr, Pb and Hg which are a prior hazard to public health, present a high toxicity because they can induce organ damage, even at lower exposure levels (Table 1).
Table 1 Heavy metals in nutrition

According to Theron et al. (Reference Theron, Tintinger and Anderson2012) and Govind and Madhuri (Reference Govind and Madhuri2014).
*Additives authorized in animal nutrition according to EC N° 1831/2003.
**Undesirable elements according to 2002/32/EC.
The toxicity of heavy metals, whether essential or not, depends on several factors including the dose, route of exposure, and chemical species, as well as the age, gender, genetics and nutritional status of the exposed individuals (Tchounwou et al., Reference Tchounwou, Yedjou, Patlolla and Sutton2012). They have different effects in relation to the dose and the time of consumption: acute poisoning for high doses in a short period and chronic poisoning or bioaccumulation for reduced exposure over a long period.
In the long term, the accumulation of heavy metals in soil can lead to a deterioration of agricultural land, eutrophication and the absorption of toxic substances. This could have long-term implications for the quality of agricultural soils, including phytotoxicity at high concentrations, the maintenance of soil microbial processes, and the transfer of zootoxic elements to the human diet due to an increased crop uptake or soil ingestion by grazing livestock (Nicholson et al., Reference Nicholson, Smith, Allowayc, Carlton-Smithd and Chambersa2003).
In the last decade the EU has been promoting the reduction of nutrient and heavy metal pollution of water and soil given that such pollutants are the major cause of eutrophication. The contamination of food and feed with heavy metals has become a serious problem in intensive agriculture.
Moreover, with regard to farming livestock, a global nutritional ecology strategy is needed in order to guarantee both the health status of humans and animals and sustainable productions. The ecology of nutrition is a multidisciplinary approach, mainly focusing on living organisms, the environment and the nutritional basis of the cooperation between organisms (function, mechanism, development) and the environment (biotic and abiotic) (Raubenheimer et al., Reference Raubenheimer, Simpson and Mayntz2009). Considering the great variety of heavy metals in the environment, it is impossible to avoid the presence of heavy metals in the food chain, and in the environment.
This study focuses on the role of animal production and on the main challenges related to the content of essential and non-essential heavy metals in animal feed and manure as a basis for developing effective approaches to the reduction of heavy metal pollution from livestock.
Legislative context
The EU authorities have thus adopted various measures to control heavy metals presence in the environment, as a result of human activities such as farming, industry, and food processing and storage contamination. Therefore, reducing heavy metal inputs to the environment and the absolute decoupling are the main focus of EU environmental protection policies given that promote continued economic growth with a reduction in environmental impacts (Jarup, Reference Jarup2003). The EU has established comprehensive regulations on the maximum authorized admissible concentrations of essential (Fe, I, Co, Cu, Mn, Zn, Mo, Se) and undesirable substances (As, Cd, F, Pb, Hg) (Table 2). Moreover, different maximum inclusion levels of essential trace elements in additives for use in animal nutrition have been set (Table 3). Main aim of those regulations (EC Reg. No 1831/2003; Dir. 2002/32/EC) is to protect feed and food safety, and ultimately human health, and reducing environmental pollution (Fink-Gremmels, Reference Fink-Gremmels2012).
Table 2 Main European Union (EU) regulations concerning essential and undesirable substances contamination

Table 3 Maximum levels of essential trace elements and undesirable substances in feeds according to different European Union legislation (EC N° 1831/2003; 2002/32/EC) and nutritional requirement for different species
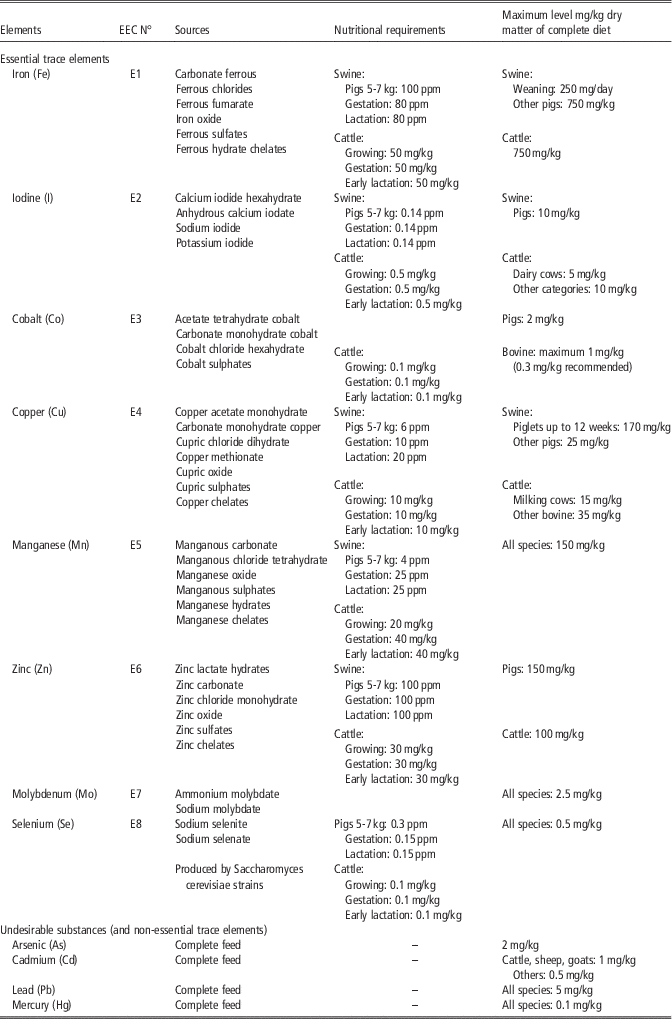
According to NRC for pigs (2012) and cattle (2000).
Considering the great variety of heavy metals in the environment and their concentration within different feed production chains, it is impossible to achieve lower levels of contamination than the detection limit for all elements in all products. It is therefore necessary to work at different levels (Aragay et al., Reference Aragay, Pons and Merkoçi2011). The EU is aware of all these problems and several laws have thus been enacted in order to control all heavy metal pollution, reduce the risk of human exposure in the food chain as well as setting up detection methods to control these contaminants in the food chain.
Heavy metals and human health
Humans and animals can be exposed to heavy metals and trace elements through different routes: the inhalation of air pollutants, consumption of contaminated drinking water, exposure to contaminated soils or industrial waste, and the ingestion of contaminated food, such as vegetables, grains, fruits, fish and shellfish and meat (Duruibe et al., Reference Duruibe, Ogwuegbu and Egwurugwu2007). The main source of exposure differs according to the elements. For example, Cd is present at low levels in most foods, such as whole grain cereals, fruit, root vegetables, meat and fish. The highest levels of Cd metals are found in the offal (kidney and liver) of mammals and in mussels, oysters and scallops. Heavy metals cause serious impacts on human and animal health including reduced growth and development, cancer, organ damage, nervous system damage, and in extreme cases, death (Table 4) (Thirulogachandar et al., Reference Thirulogachandar, Rajeswari and Ramya2014). The International Agency for Research on Cancer considered some heavy metals as carcinogenic to humans, based on evidence on human studies. Some metals are particularly toxic to the sensitive, rapidly developing systems of fetuses, infants and young children. Pb and Hg in particular, can easily cross the placenta and damage the fetal brain (Food Safety Authority of Ireland, 2009).
Table 4 Effects of different essential heavy metals on human health and their specific food sources

Childhood exposure to some metals can result in learning difficulties, memory impairment, damage to the nervous system, and behavioral problems such as aggressiveness and hyperactivity. At higher doses, heavy metals can cause irreversible brain damage (Jaishankar et al., Reference Jaishankar, Tseten, Anbalagan, Mathew and Beeregowda2014). It is also very important to consider that children receive higher doses of metals from food than adults, as they consume more food in relation to their BW (Thirulogachandar et al., Reference Thirulogachandar, Rajeswari and Ramya2014). The probability that a population will be exposed and harmed could be higher for a metal with a moderate toxicity but which is widespread and thus there is a higher risk of exposure. The environmental conditions, such as soil contamination, industrial processes and incorrect manufactory procedures, are responsible for high levels of Sn, Cu, Ni and Co.
Heavy metals in feed/food chain
Undesirable substances of heavy metals (Cd, Pb, Hg, As, Cr) as well as essential heavy metals (Fe, I, Co, Cu, Mn, Mo, Se) have potential adverse effects on livestock directly, but can also enter the food chain through animal consumption and thus represent a risk for humans. Concerning the evaluation of exposure levels to toxic metals or to toxic doses, it is important to consider that the dietary intake can be influenced by several factors: management, type and quality of raw materials, additives, soil ingestion and accidental contamination. Episodes of acute toxicity are uncommon, with the exception of accidental exposure. Heavy metals are potentially dangerous due to their toxicity, bioaccumulation and bio-magnification when found within living tissues, and are stored more quickly than they are excreted. Heavy metals are considered contaminants or undesirable substances (As, Cd, F, Pb, Hg) when they are not intentionally added to food, but may reach the feed and food chains throughout different sources (Jarup, Reference Jarup2003).
The carry-over of orally administered metals into animal-origin food (milk, eggs, meat) (Table 5) is related to the rate of absorption, bioaccumulation, metabolism and excretion (Cheli et al., Reference Cheli, Pinotti, Rossi and Dell’Orto2013). These biological phenomena largely vary depending on the chemical form, are dose- and/or time dependent (especially for some bio accumulative elements such as cadmium), and are influenced by other factors such as the interaction with other compounds (e.g. Cd greatly interferes with essential elements such as Cu and Zn) (Fink-Gremmels, Reference Fink-Gremmels2012).
Table 5 Effects of different essential and non-essential heavy metals as contaminants on human health, the specific carry-over effects and their sources

EHM=essential heavy metal; NHM=non-essential heavy metal.
With regard to Cd, Pb, As and Hg different studies showed that the carry-over to milk, egg and muscle is generally low if animals are fed with a standard diet (with a concentration of heavy metals below the EU maximum permissible levels). Conversely, with a higher dietary toxic metal exposure, a general increase in residues in the specific accumulation organs (liver, kidney, bones) has been observed (Thirulogachandar et al., Reference Thirulogachandar, Rajeswari and Ramya2014). Thus, the control of the animal input could be an effective strategy to reduce human health risks related to the consumption of animal-origin products.
The accumulation of heavy metals varies significantly from one tissue to another within an animal, and between animals. Higher As concentrations have been detected in breast meat, the essential edible part of poultry; and kidney and gizzard showed the highest content of Cd and Cr, respectively (Mohammed et al., Reference Mohammed, Kolo and Geidam2013). A positive correlation between dietary intake and concentration in broiler organs (muscles, liver, and skin) has also been demonstrated for essential metals as Fe and Mn (Rehman et al., Reference Rehman, Andleeb, Mahmood, Bukhari, Naeem and Yousaf2012).
Among animal products, eggs are also a possible source of heavy metal contamination. Radu-Rusu et al. (Reference Radu-Rusu, Pop, Albu, Bologa and Radu-Rusu2013) compared the heavy metal transferability in improved cages and free-range reared hens, and discovered that free-range eggs had higher concentrations of heavy metals, compared with the conventionally-produced eggs, due to the intense soil contamination with these pollutants. The Cd level was 0.018 v. 0.023 ppm in the free-range group; 2.591 v. 2.734 ppm for essential Cu, and the essential Zn content was 5.386 ppm in improved cages v. 5.522 ppm.
Ruminants are less susceptible to As toxicosis and do not show any sign of toxicity unless the concentration is more than 200 to 300 mg of inorganic As/kg of feed (Kochare and Tamir, Reference Kochare and Tamir2015). Dairy cows are more susceptible to the accumulation of Cd and Pb than beef cattle, however both suffer from mercury toxicity. This heavy metal, especially in the form of methyl, is highly toxic and can lead to incoordination, decline in awareness, alopecia and visual and gastrointestinal disorders. García-Vaquero et al. (Reference García-Vaquero, Lopez-Alonso, Benedito, Hernandez, Gutiérrez and Miranda2011) investigated intensively farmed beef cattle and demonstrated that essential Cu accumulation in the liver had negative effects on animal performance, and found a reduced feed intake and average daily gain. This decreased growth performance may be due to the production of reactive oxygen species following excess supplementation with Cu.
Fish containing the valuable proteins and n-3 polyunsaturated fatty acids and could represent source of heavy metal exposure to humans (Nnaji et al., Reference Nnaji, Uzairu, Gimba and Kagbu2011). According to Qiu et al. (Reference Qiu, Lin, Liu and Zeng2011), heavy metal accumulation in fish tissues depends largely on their concentrations in water, in prey or commercial feed. Dangerous heavy metals of interest include Hg, As which are and carcinogenic. However, heavy metals tend to accumulate more in the visceral tissues of fish (liver, kidney, intestines, etc.), which are normally discarded during the manufacturing processes, than in the muscles (Nnaji et al., Reference Nnaji, Uzairu, Gimba and Kagbu2011). Qiu et al. (Reference Qiu, Lin, Liu and Zeng2011) found significant relationships between the concentrations of essential Cu and Zn and lipid contents in two farmed fish species (pompano and snapper). Their findings indicate that lipid content may be an important factor regulating the bioaccumulation of these metals. Cu is an essential metal for both fish and humans, however Cu poisoning induces gill, liver and kidney damage in fish, leading to mortalities.
Mineral nutrition in livestock
Livestock nutrition plays a pivotal role not only in guaranteeing the animal requirements and thus preventing nutritional deficiency, but also in improving animal health and welfare, productivity, food safety and also in controlling environmental pollution. All animals require mineral nutrition including some heavy metals that have been demonstrated to be essential nutrients. Minerals, such as Co, Cu, Fe, I, Mn, Mo, Se, and Zn, are part of the numerous enzymes that coordinate many biological processes, and consequently are essential to maintain animal health and productivity (Lopez-Alonso et al., Reference Lopez-Alonso2012a). Essential metals perform four important types of function: structural, physiological, catalytic and regulatory (Suttle, Reference Suttle2010).
Many essential trace elements used in intensive livestock are found in manure, in direct proportion to the quote supplied over the minimal requirements. From a mineral nutrition point of view, and in order to prevent mineral deficiencies that could compromise the production, commercial feeds are often supplemented with minerals in order to promote the optimum growth rate, functional bioactivity and antimicrobial properties. For example, Se is naturally present in many foods, such as yeast. It plays a critical role in reproduction, DNA synthesis, hormone metabolism and protects the body from infection, oxidative damage and has an important bioactive role related to a decrease in the susceptibility to carcinogens (Dai et al., Reference Dai, Jones, Lee, Li, Post and Herrman2016).
Farmers usually balance animal diets with minerals according to the maximum acceptable levels established by EU authorities. Nevertheless, the maximum permitted amount in feed is usually greater than the minimum requirement, resulting in the wide diffusion of minerals into the environment. For example, the minimum nutrient requirement for Zn (NRC, 2012) ranges between 50 and 100 ppm in different grow phases, however it is often used as an additive thus taking the level to 150 ppm, which is the maximum acceptable level established by the European authorities.
Although, the net requirements of essential metals are lower than the dietary needs, different aspects need to be considered in order to establish the optimal concentration in feed: genetic influences, dietary factors, interaction among nutrients, bioavailability and subclinical toxic effects. The optimal mineral supplementation, which is represented as a band between the adequate and inadequate toxic dietary concentration established by the dose/response, should also consider unnecessary levels excreted into the environment. The ban on antibiotic growth promoters (EU Reg. 1831/2003) in livestock led to the study of alternative compounds (Rossi et al., Reference Rossi, Di Giancamillo, Reggi, Domeneghini, Baldi, Sala, Dell’Orto, Coddens, Cox and Fogher2013 and Reference Rossi, Dell’Orto, Vagni, Sala, Reggi and Baldi2014a), however it increased the use of some minerals as growth stimulants and to prevent enteric diseases in pigs.
High doses of Zn in the form of premix have thus been widely used in several EU countries. Zn is essential in the maintenance and restoration of barrier integrity, protection against pathogens and modulation of the immune system, promoting antibody production against intestinal pathogens (Rossi et al. Reference Rossi, Pinotti, Agazzi, Dell’Orto and Baldi2014c). In addition, Zn may reduce diarrhea and increase growth rates in weaning piglets (Sales, Reference Sales2013; Walk et al., Reference Walk, Wilcock and Magowan2015). Although the pharmacological use of Zn (2500 to 3000 mg of Zn/kg), available with a veterinary prescription, can reduce intestinal disorders after the weaning of pigs, from a nutritional and ecological point of view, a better strategy would be to identify and counter the main cause of diarrhea (Rossi et al. Reference Rossi, Pinotti, Agazzi, Dell’Orto and Baldi2014c).
Cu is another important mineral that is profoundly connected with livestock production. When this trace element is added to the diet of fattening pigs, it causes a faster growth and better feed-conversion ratio (Polen and Voia, Reference Polen and Voia2015). In pigs, dietary concentrations of 150 to 250 mg of Cu/kg can maximize growth performance without exposing animals to any risk of poisoning. Also in poultry production Cu, Zn and Mn prevents some diseases: Cu prevents anemia, whereas Zn and Mn act as catalysts in many enzymatic and hormonal reactions (Suleiman et al., Reference Suleiman, Ibitoye, Jimoh and Sani2015).
Although corn tissues cultivated in soil with high amounts of Cu do not accumulate it at toxic levels, Cu represents an environmental concern, and can enter into the human food chain by accidental soil ingestion, contamination of edible plants by soil, or by the consumption of contaminated products of an animal food origin (Alfthan et al., Reference Alfthan, Eurola, Ekholm, Venäläinen, Root, Korkalainen, Hartikainen, Salminen, Hietaniemi, Aspila and Aro2015).
In order to develop a sustainable nutritional strategy, the maximum permissible levels should not be considered as optimal, and mineral supplementation should be established in terms of the desirable limits, which should be lower than the legal ones (Eu et al., Reference Eu, Yang, He and Mahmood2007).
In conventional farms, minerals can be supplemented in different forms. Inorganic salts, such as sulphates, carbonates, chlorides and oxides, are the most common ones. When ingested, these salts are broken down in the digestive tract to form free ions which are then absorbed (Lopez-Alonso, Reference Lopez-Alonso, Garcia-Vaquero, Benedito, Castillo and Miranda2012a). In order to ensure the extranutritional effect of some elements on animals, the concentrations of these salts often exceed the physiological requirements, causing fecal excretion. Thus, there has been increasing interest in chelated compounds as hyperavailable mineral sources, thus reducing the dispersal of minerals in manure. Various studies have shown that chelated minerals can be included at much lower levels without compromising performance, minimizing nutrient excretion and the overall environmental impact (Lopez-Alonso, Reference Lopez-Alonso, Garcia-Vaquero, Benedito, Castillo and Miranda2012b). However more light needs to be showed on this matter, in fact fecal Zn excretion is related to the dietary Zn concentration rather than the source.
For an optimal mineral supplementation, the various interactions among minerals and diet components need to be considered. Positive and negative nutrient interactions, involve the impact of the nutrient on another nutrient’s bioavailability, including absorption and use. For example, Cu interacts mainly negatively with Mn, Zn and Fe (García-Vaquero et al., Reference García-Vaquero, Lopez-Alonso, Benedito, Hernandez, Gutiérrez and Miranda2011).
Various proteins bind and carry certain minerals including Fe, Cu and Ca and thus an inadequate protein intake may impair the function of these nutrients (Collins et al., Reference Collins, Prohaska and Knutson2010). The quality and quantity of dietary fiber can negatively influence the absorption of several minerals, including Ca and Fe. In monogastric animals, phytic acid, found in cereals and legumes, can bind minerals to insoluble complexes, thus decreasing the absorption of 2+ chemical configurations. The interaction of vitamins and minerals has also been described in several metabolic situations and is still under investigation. Many trace elements are recognized as oxidants, which may deteriorate animal feed, in particular during long-term storage at high temperatures (Medardus et al., Reference Medardus, Molla, Nicol, Morrow, Rajala-Schultz, Kazwala and Gebreyes2014).
A well-balanced diet is generally recommended in order to meet the requirements of all nutrients, considering possible interactions among nutrients, preventing deficiencies and chemical excesses or imbalances. For sustainable animal production and to develop effective approaches to preserving long-term soil and water quality strategies from heavy metal pollution, it is necessary to understand the nutritional basis of the interactions between organisms and the environment.
Heavy metals in feed: worldwide situation
Farming practices vary widely according to the global soil and climatic conditions, thus heavy metal contamination in feed can have divergent concentrations and strictly depends on the location and legal restrictions (Table 6).
Table 6 Comparison of content of heavy metals in feed in the USA, China and England and Wales (EW)
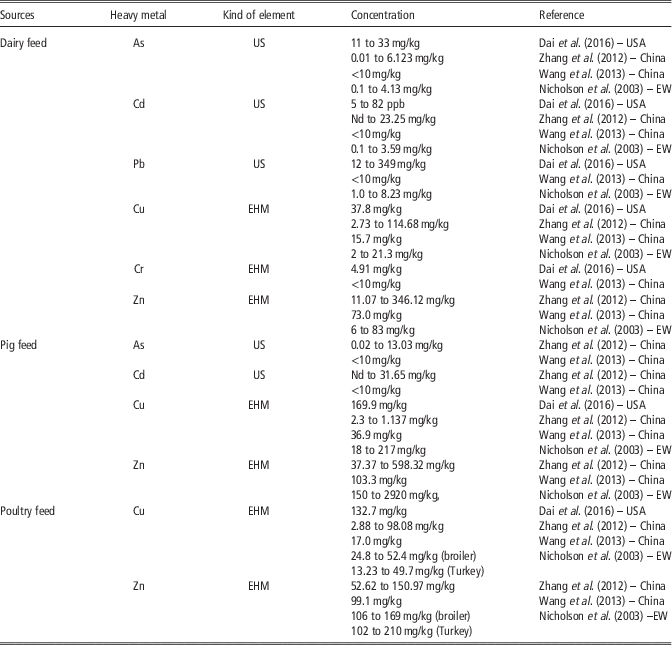
US=undesirable substances in animal feed; EHM=essential heavy metal; Nd=non-detectable.EW=England and Wales.
To be able to predict the risk of exposure to toxic doses of metals, it is important to consider the production system. Extensive ruminant farms, both beef cattle and dairy, need land to produce forage (hay, straw and silage) as energy sources for the ruminal symbiotic microflora (Zaninelli et al., Reference Zaninelli, Rossi, Costa, Tangorra, Agazzi and Savoini2015). Naturally, all soils contain different concentrations of heavy metals. Furthermore, such components are also added to agricultural soils through the application of mineral and organic fertilizers, direct defecation and urination by animals, and pesticides (Kochare and Tamir Reference Kochare and Tamir2015). In these cases, exposure in animals may vary in relation to the quality of the soil, the use of inorganic fertilizers and anthropogenic activities. Animals in intensive systems usually receive concentrate feed, with raw materials from a global market supplemented by mineral additives. By accurate monitoring in intensive systems, the control of the input of heavy metals is easier than in extensive systems.
Feed additives are widely applied in animal production in all described locations and Cu and Zn are present in feeds largely. The content of heavy metals, especially Cu and Zn in swine feed has been found to be higher than in poultry feed or in cattle feed. According to Dai et al. (Reference Dai, Jones, Lee, Li, Post and Herrman2016) more than half of the Wisconsin (USA) dairy farms used feed rations containing Cu above the recommended levels. Zhang et al. (Reference Zhang, Li, Yang and Li2012) reported that in north-east China Cu, Zn and As were found in all feed samples. According to Wang et al. (Reference Wang, Dong, Yang, Toor and Zhang2013) in China (Jiangsu Province), median concentrations of Zn were the highest heavy metals found, followed by Cu. The median concentrations of undesirable substances such as Hg, As, Pb, Cd and Cr in all feeds were below 10 mg/kg (Table 6). This is probably related to the different management of raw materials and feed, animal species as well as different legal limits.
The concentration of toxic undesirable metals, such as Cd and Pb, was higher in forage than in concentrate feed materials and in particular in herbage cultivated near industrial areas. This is probably related to the contamination of forage with soil and not to the plant uptake. Instead, the main sources of As and Hg in feeds are represented by non-plant materials such as products of marine origin.
Heavy metals in livestock manure
Heavy metals can be introduced into agricultural soil through manure. The input of organic waste to agricultural soil increases organic matter, introduces nutrients, improves soil structure and increases nutrient absorption by plants, which improves soil fertility and quality (Gul et al., Reference Gul, Naz, Fareed, Khan and Irshad2015). Despite, the considerable fertilizer value of slurry, it may be abundant in Zn, Cu and other heavy metals derived from animal intake (Jakubus et al., Reference Jakubus, Dach and Starmans2013). The heavy metal composition of composts varies widely depending on the geographical location, sources and composting process (Faridullah et al., Reference Faridullah, Pervaiz, Irshad, Alam, Mahmood and Ashraf2014).
Ingelmo et al. (Reference Ingelmo, Molina, Desamparados Soriano, Gallardo and Lapeña2012) reported on digested sewage sludge (collected from Spanish farms), during the compost process. They found that the heavy metal content varies and total Zn, Pb, Cu and Ni content increased during the composting process. On the final composting day, the content was ranked Zn>Pb>Cu>Ni>Cd (Table 7). Thus, the total content of heavy metals depends on the organic matter transformation, which may influence the bioavailability of metals and in the end render the metals in more available forms.
Table 7 Essential and non-essential heavy metal content (mg/kg) in sludge-based compost on different days of composting

EHM=essential heavy metal; NHM=non-essential heavy metal.
Ingelmo et al. (Reference Ingelmo, Molina, Desamparados Soriano, Gallardo and Lapeña2012).
Faridullah et al. (Reference Faridullah, Pervaiz, Irshad, Alam, Mahmood and Ashraf2014) have shown that acid-extracted metals were also higher in composted manure than fresh manure. The authors collected animal-composted waste samples (Abbottabad District, Pakistan). They were ranked Fe>Hg>Mn>Zn>Ni. In addition, Fe, Mn and Hg concentrations were higher in the composted manures, whereas Ni and Zn showed their maximum concentrations in fresh manures. The maximum Fe concentration was detected in composted buffalo manure. Faridullah et al. (Reference Faridullah, Pervaiz, Irshad, Alam, Mahmood and Ashraf2014) observed a different trend in metal extraction. The high content of Fe was related to the different feed composition or products of fish origin.
According to Jakubus et al. (Reference Jakubus, Dach and Starmans2013) tested slurries (collected from Dutch farms) containing Cu and Zn were from 1.5 to 3.0 times higher in swine slurry than cattle slurry. Nicholson et al. (Reference Nicholson, Smith, Allowayc, Carlton-Smithd and Chambersa2003) showed that in England and Wales, the highest metal concentrations for swine and cattle and poultry livestock manures were Zn and Cu. In sewage sludge the highest concentrations of heavy metals were also Zn, Cu and also Pb, Cr.
In China, the highest concentrations for swine livestock manures were: Zn, Cu, Cr and Pb; and for cattle manures: Zn, Cu, Pb and Cr. The contents of trace elements in animal manures increased over a decade, with the use of feed additives (Luo et al., Reference Luo, Maa, Zhang, Wei and Zhu2009). Zhang et al. (Reference Zhang, Li, Yang and Li2012) showed that in north-east China, the contents of Cu and Zn in manures of different size farms (small, medium and large farms) were significantly higher than other metals detected. In cattle and chicken manure, there was no significant difference in the content of heavy metals from farms of different sizes (Table 8). In China, in the last decade an increase in the content of Zn in manure has been found. This is related to the high content of Zn in animal additives, which have usually resulted in a higher concentration in the manure.
Table 8 Concentration of different essential and non-essential heavy metals in selected livestock manures in different countries

CM=composted manure; FM=fresh manure; S=small; animal population (head): cattle <100, chicken <2000, swine <200; M=middle; animal population (head): cattle 100 to 300, chicken >2000, swine 200 to 800; L=large; animal population (head) : cattle >300, chicken >20 000, swine >800; EHM=essential heavy metal; NHM=non-essential heav metals; d.m.=dry matter.
The heavy metal contents of animal manures are largely a reflection of their content in the feed, which poses a high pollution risk to farmlands. Considering the different kinds of farms and different species, swine and poultry represent the most important sources of Zn and Cu pollution. This is also linked to the additives used in animal feed (Nicholson et al., Reference Nicholson, Smith, Allowayc, Carlton-Smithd and Chambersa2003; Luo et al., Reference Luo, Maa, Zhang, Wei and Zhu2009; Zhang et al., Reference Zhang, Li, Yang and Li2012, Jakubus et al., Reference Jakubus, Dach and Starmans2013). Consequently, swine and poultry farms may have the highest risk for agricultural lands. In terms of environmental protection, animal feed additives should be monitored based on the legal limits in each country (Zhang et al., Reference Zhang, Li, Yang and Li2012). Different approaches are also needed for reducing heavy metal inputs to agricultural land and to target policies for preserving long-term soil quality (Nicholson et al., Reference Nicholson, Smith, Allowayc, Carlton-Smithd and Chambersa2003).
Heavy metal content in agricultural soil
The soil represents an important risk in terms of the livestock exposure to heavy metals due to accidental ingestion, contamination of forage, and absorption by edible plants. In most EU member states, but also in the rest of the world, complete inventories of soil are lacking. Quantifying the full extent of local soil pollution is therefore difficult, although this is an important further objective of the EU in the proposed Soil Framework Directive. According to the European Environment Agency (2006) measurements, there were a total of three million potentially contaminated locations in the EU, of which 250 thousand were actually contaminated (Dir. 2004/35/EC). Therefore, reducing heavy metal contamination in the soil is a strategic target for EU soil protection policies (Nicholson et al., Reference Nicholson, Smith, Allowayc, Carlton-Smithd and Chambersa2003).
In various parts of the world widely different levels of trace elements in the agricultural industry have been observed. Zn pollution has become a general global problem. Zn contamination has reported in all described locations (Nicholson et al., Reference Nicholson, Smith, Allowayc, Carlton-Smithd and Chambersa2003; Luo et al., Reference Luo, Maa, Zhang, Wei and Zhu2009; Belon et al., Reference Belon, Boisson, Deportes, Eglin, Feix, Bispo, Galsomies, Leblond and Guellier2012) (Table 9). In fact, Zn is monitored by authorities as it is responsible for eutrophication and water pollution. Depending on the country, there are main sources of Zn pollution: atmospheric deposition and livestock manure. The livestock industry contributes to Zn pollution as it is widely used in animal feed as additives. Authorities should thus monitor the pollution and create new strategies for the improved management of animal nutrition. This would help to prevent soil contamination and to build an approach based on ecological nutrition, which could remain sustainable development between economic development, social development and environmental protection. The pressure on agricultural land in China is almost nine times higher for Zn (187 742 g/ha per year) and more than 14 times higher for Cu (71 824 g/ha per year) compared with Germany (Zn – 21 237 g/ha per year) and France (Cu – 4 869 g/ha per year) which have the highest annual input of Zn and Cu in the total area of agricultural land, in all described countries in Europe. These differences between China and Europe are probably related to different legal restrictions.
Table 9 Comparison of annual input of essential and non-essential heavy metals in soil for one million of ha (mln ha) yearly in various countries
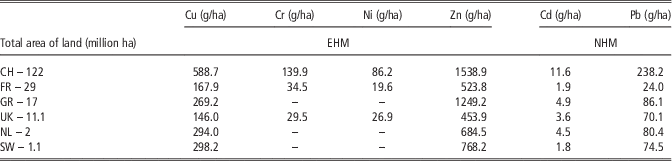
EHM=essential heavy metal; NHM=non-essential heavy metal; CH=China; FR=France; GR=Germany; NL=the Netherlands; SW=Switzerland.
Adapted from Nicholson et al. (2003); Luo et al. (2009); Belon et al. (2012).
In the EU in terms of the entire agricultural land area, atmospheric deposition has been reported to be the main source of most metals, ranging from 25% to 85% of total inputs (Nicholson et al., Reference Nicholson, Smith, Allowayc, Carlton-Smithd and Chambersa2003). According to Luo et al. (Reference Luo, Maa, Zhang, Wei and Zhu2009), in China atmospheric deposition and livestock manures were also the predominant sources of trace elements in agricultural land. In agricultural soils in China, atmospheric deposition may be responsible for 43% to 85% of the total As, Cr, Hg, Ni and Pb inputs. The average atmospheric deposition flux of As in China is about 100 times higher than that in Europe. These sources are related to agricultural and industrial activities (Belon et al., Reference Belon, Boisson, Deportes, Eglin, Feix, Bispo, Galsomies, Leblond and Guellier2012) (Table 10).
Table 10 Essential and non-essential heavy metals in atmospheric deposition rate yearly in the UK (Nicholson et al., Reference Nicholson, Smith, Allowayc, Carlton-Smithd and Chambersa2003), France (FR) (Belon et al., Reference Belon, Boisson, Deportes, Eglin, Feix, Bispo, Galsomies, Leblond and Guellier2012) and China (CH) (Luo et al., Reference Luo, Maa, Zhang, Wei and Zhu2009)

EHM=essential heavy metal; NHM=non-essential heavy metal.
In England, livestock manure and sewage sludge are also important sources, responsible for an estimated 37% to 40% and 8% to 17% of total Zn and Cu inputs. According to Belon et al. (Reference Belon, Boisson, Deportes, Eglin, Feix, Bispo, Galsomies, Leblond and Guellier2012) in France animal manure, mineral fertilizers and pesticides are the predominant sources of heavy metals. Livestock manure was the predominant (>50%) source of Zn, Cu, Mo Ni, As, and Hg.
Although the toxic metal concentration in feedstuff from unpolluted soil has been found to be in line with the safety limits established by the EU, a renewed inventory of metal inputs into agricultural soils is of immense importance in order to assess the environmental risks posed by contaminated agricultural soils (Luo et al., Reference Luo, Maa, Zhang, Wei and Zhu2009).
Strategies to control heavy metal pollution
Controlling environmental losses and the spread of contaminants from livestock manure is essential in balanced production systems and in order to achieve the homeostasis of agriculture with natural habitats. Although, as in most European countries, spreading manure near to surface water and on frozen soil is illegal, the accidental release of farm waste to water has resulted in outbreaks of serious illnesses. There is thus is a need for technologies and strategies to control these environmental problems. Efforts are also needed to close nutrient cycles on farms by recycling nutrients in livestock manure which will reduce pollution problems and limit heavy metals in soil (Petersen et al., Reference Petersen, Sommer, Béline, Burton, Dach, Dourmad, Leip, Misselbrook, Nicholson, Poulsen, Provolo, Sørensen, Vinnerås, Weiske, Bernal, Böhm, Juhász and Mihelic2007; Gerber et al., Reference Gerber, Uwizeye, Schulte, Opio and Boer de2014). Therefore, many studies have described strategies to control the content of heavy metals in livestock manure and in soil. Effective strategies should focus on a reduction in the heavy metal input/output ratio in livestock. Thus, different multidisciplinary approaches should be considered to reduce the animal intake, but also the excretion in feces and the concentration in manure.
The manipulation of the diet could be a useful way to control the amount of manure produced together with its composition, because nutrients found in manure or in compounds derive from the fraction of the feed that is not absorbed by the animals. A formulated diet is needed that increases the efficiency of nutrient retention by animals, decreasing their excretion in feces and urine and reduce the import of nutrients in feed and mineral mixtures from outside the farm (Petersen et al., Reference Petersen, Sommer, Béline, Burton, Dach, Dourmad, Leip, Misselbrook, Nicholson, Poulsen, Provolo, Sørensen, Vinnerås, Weiske, Bernal, Böhm, Juhász and Mihelic2007). For instance, in pigs and poultry, the use of industrial amino acids is a very efficient way to reduce nitrogen excretion. At the farm level this thus leads to a significant reduction in the import of protein rich feedstuffs, such as soybean meal. The inclusion of enzymes in the feed which improves the biological availability of some specific nutrients has been shown to be efficient in many species. Animal feeding plays an important role in the control of nutrient flows on livestock farms (Petersen et al., Reference Petersen, Sommer, Béline, Burton, Dach, Dourmad, Leip, Misselbrook, Nicholson, Poulsen, Provolo, Sørensen, Vinnerås, Weiske, Bernal, Böhm, Juhász and Mihelic2007). Using mineral supplements of trace minerals could help prevent the ‘waste’. The maximum permitted level should not be considered as the ideal level for animal requirement and alternative innovative compounds to antibiotics but also to Zn and Cu should be used to control enteric diseases (Rossi et al., Reference Rossi, Fusi, Boglioni, Giromini, Rebucci and Baldi2014b).
An excessive heavy metal output still can penetrate the soil and water from manures, thus there is a need for different technologies to remove the content of heavy metals from contaminated soil and water in agricultural land (He et al., Reference He, Yanga and Stoffell2005). To reduce the heavy metal output from livestock, different approaches to treat the manure have been studied and can be applied in the field.
Electroremediation which passes an electric current through liquid manure and metal ions are precipitated on an electrode, can reduce metal concentrations. However, at present the technology is unproven at the farm-scale and is unlikely to be cost-effective. From a whole-farm perspective, the recycling loop of manure back into food production should be as short as possible in order to minimize the environmental impact and ensure a high nutrient efficiency (Petersen et al., Reference Petersen, Sommer, Béline, Burton, Dach, Dourmad, Leip, Misselbrook, Nicholson, Poulsen, Provolo, Sørensen, Vinnerås, Weiske, Bernal, Böhm, Juhász and Mihelic2007).
According to Bhargava et al. (Reference Bhargava, Carmona, Bhargava and Srivastava2012) phytoremediation is simple cleanup technology which has promising possibilities to eliminate metals from agricultural land, through the use of plants that accumulate large amounts of heavy metal contaminants. This technology was developed a few decades ago from the recognition that plants were capable of metabolizing toxic pesticides (Van Aken, Reference Van Aken2009). It is perceived as an acceptable, cost-effective and efficient, novel technology with acceptability among the communities. Phytoremediation comprises several techniques that use plants and associated microbes to remediate contaminated matrices, which are removed through transfer, containment, accumulation or dissipation. The fact that phytoremediation is usually carried out in situ contributes to its cost-effectiveness and may reduce the exposure of the polluted substrate to humans, wildlife and the environment (Pilon-Smits, Reference Pilon-Smits2005). Depending on the conditions, the level of clean-up required, the plants used and the contaminants, phytoremediation can be divided into four types: phytoextraction, phytofiltration, phytostabilization and phytovolatilization (Thangavel and Subbhuraam, Reference Thangavel and Subbhuraam2004; Ali et al., Reference Ali, Khan and Anwar Sajad2013).
The manipulation of the diet could be a useful way to control the amount of manure produced together with its composition, because nutrients found in manure or in compounds derive from the fraction of the feed that is not absorbed by the animals. A formulated diet is needed that reduces the efficiency of nutrient retention by animals, increases their excretion in feces and urine and decreases the import of nutrients in feed and mineral mixtures from outside the farm (Petersen et al., Reference Petersen, Sommer, Béline, Burton, Dach, Dourmad, Leip, Misselbrook, Nicholson, Poulsen, Provolo, Sørensen, Vinnerås, Weiske, Bernal, Böhm, Juhász and Mihelic2007). For instance, in pigs and poultry, the use of industrial amino acids is a very efficient way to reduce nitrogen excretion. At the farm level this thus leads to a significant reduction in the import of protein rich feedstuffs, such as soybean meal. The inclusion of enzymes in the feed which improves the biological availability of some specific nutrients has been shown to be efficient in many species. Animal feeding plays an important role in the control of nutrient flows on livestock farms (Petersen et al., Reference Petersen, Sommer, Béline, Burton, Dach, Dourmad, Leip, Misselbrook, Nicholson, Poulsen, Provolo, Sørensen, Vinnerås, Weiske, Bernal, Böhm, Juhász and Mihelic2007). Using mineral supplements of trace minerals could help prevent the ‘waste’. The maximum permitted level should not be considered as the ideal level for animal requirement and alternative innovative compounds to antibiotics but also to Zn and Cu should be used to control enteric diseases (Rossi et al., Reference Rossi, Fusi, Boglioni, Giromini, Rebucci and Baldi2014b).
An excessive heavy metal output still can penetrate the soil and water from manures, thus there is a need for different technologies to remove the content of heavy metals from contaminated soil and water in agricultural land. To reduce the heavy metal output from livestock, different approaches to treat the manure have been studied and can be applied in the field.
Electroremediation which passes an electric current through liquid manure and metal ions are precipitated on an electrode, can reduce metal concentrations. However, at present the technology is unproven at the farm-scale and is unlikely to be cost-effective. From a whole-farm perspective, the recycling loop of manure back into food production should be as short as possible in order to minimize the environmental impact and ensure a high nutrient efficiency.
According to Bhargava et al. (Reference Bhargava, Carmona, Bhargava and Srivastava2012) phytoremediation is simple cleanup technology which has promising possibilities to eliminate metals from agricultural land, through the use of plants that accumulate large amounts of heavy metal contaminants. This technology was developed a few decades ago from the recognition that plants were capable of metabolizing toxic pesticides (Van Aken, Reference Van Aken2009). It is perceived as an acceptable, cost-effective and efficient, novel technology with acceptability among the communities. The proper plants for removal heavy metals should have the following components: (i) high growth rate, (ii) highly branched and widely distributed root system, (iii) good adaptation to prevailing environmental and climatic conditions, (iv) easy cultivation and harvest, (v) production of more above-ground biomass, (vi) resistance to pathogens and pests, (vii) more accumulation of the target heavy metals from soil, (viii) translocation of the accumulated heavy metals from roots to shoots and (ix) tolerance to the toxic effects of the target heavy metals (Sakakibara et al., Reference Sakakibara, Ohmori, Ha, Sano and Sera2011; Shabani and Sayadi, Reference Shabani and Sayadi2012; Ali et al., Reference Ali, Khan and Anwar Sajad2013; Maric et al., Reference Maric, Antonijevic and Alagic2013). Phytoremediation comprises several techniques that use plants and associated microbes to remediate contaminated matrices, which are removed through transfer, containment, accumulation or dissipation. The fact that phytoremediation is usually carried out in situ contributes to its cost-effectiveness and may reduce the exposure of the polluted substrate to humans, wildlife and the environment (Pilon-Smits, Reference Pilon-Smits2005). Depending on the conditions, the level of clean-up required, the plants used and the contaminants, phytoremediation can be divided into four types: phytoextraction, phytofiltration, phytostabilization and phytovolatilization (Thangavel and Subbhuraam, Reference Thangavel and Subbhuraam2004; Ali et al., Reference Ali, Khan and Anwar Sajad2013).
Phytoextraction is the uptake of contaminants from soil or water by plant roots and their translocation to and accumulation in above-ground biomass (Rafati et al., Reference Rafati, Khorasani, Moattar, Shirvany, Moraghebi and Hosseinzadeh2011). Phytofiltration is the removal of pollutants from contaminated surface waters or wastewater (Mukhopadhyay and Maiti, Reference Mukhopadhyay and Maiti2010). Removal reach by plants roots (rhizofiltration) or seedlings (blastrofiltration). Seeding roots or plants roots rose in aerated water absorb, precipitate and concentrate heavy metals (Thangavel and Subbhuraam, Reference Thangavel and Subbhuraam2004). Phytostabilization is used to reduce the mobility and bioavailability of pollutants in the environment, thus preventing their migration to groundwater or their entry into the food chain (Erakhrumen, Reference Erakhrumen2007). Phytovolatilization is the uptake of pollutants from soil by plants, their conversion to volatile form and subsequent release into the atmosphere. Among these techniques phytoextraction is the main and most useful technique for removal of heavy metals and metalloids from polluted soils or water (Ali et al., Reference Ali, Khan and Anwar Sajad2013).
The effectiveness of phytoremediation is highly influenced by the bioavailability of metals in soil that depends on several factors: chemical composition, pH, geochemical characteristic of metals, environmental variables and agricultural soil management (Thangavel and Subbhuraam, Reference Thangavel and Subbhuraam2004). Bioavailability can be increased by lowering pH of soil, using fertilizers, soil microorganisms, root exudates and adding chelating agents (Lone et al., Reference Lone, He, Stoffella and Yang2008).
Conclusions
In the commercial agricultural industry, heavy metals are represented as both mineral nutrients and contaminants/undesirable substances. Although EU has established a comprehensive regulation to control their pollution, their spread at different level does not allow avoiding the presence of heavy metals in the food chain, and in the environment. The control of the animal input could be an effective strategy to reduce human health risks related to the consumption of animal-origin products and the environmental pollution by manure. The diets of livestock can be manipulated in order to reduce the quote of non-absorbed minerals and nutrients that can be present in the manure.
To set up effective strategies against heavy metals the complex interrelationships in rural processes, the widely variability of farming practices, the soil and climatic conditions must be considered. Using the additives with more precision should be suggested in order to avoid spreading the contaminations to the environment.
Innovative and sustainable approaches have discussed for the heavy metal nutrition ecology to control the environmental pollution from livestock-related activities.
Acknowledgments
This work was supported by the Ministry of Agriculture Food and Forestry Policies under the Grant MIPAAF 2015.