Implications
Grain/starch digestion rate coefficients show an inverse square dependence on milled grain particle size, providing an opportunity for the pig feed industry to determine optimum particle size distributions for complete digestion in the small intestine to maximise feed utilisation. Mechanistic models based on physiological factors controlling digesta passage rate and feed intake provide a platform for researchers in human and pig nutrition to form testable hypotheses for increasing or decreasing intake by: (1) formulating diets based on grains of different digestibility; and (2) adding fibre of different fermentability at different concentrations.
Introduction
Cereal grains are usually the main source of dietary energy for monogastric production animals. Feed costs constitute 60% to 70% of total pork production costs. Digestive utilisation energy varies from 70% to 90% for most pig diets, and the rest (10% to 30%) is excreted in urine, faeces, lost as body heat and fermented in the gut and lost as gases (Noblet and Henry, Reference Noblet and Henry1993). Therefore, not all of the energy contained in feed grains can be used by animals, depending on feed grain characteristics and physiological limitations of the animal. Maximising energy utilisation for performance and growth is a major aim of the pig production industry. Animal growth and performance are directly dependent on nutrient and feed intake (FI) (Nyachoti et al., Reference Nyachoti, Zijlstra, De Lange and Patience2004; Black et al., Reference Black, Williams, Gidley, Torrallardona and Roura2009). Physiologically, FI is thought to be, in part, dependent on macronutrient (particularly starch) digestion rate and the consequent passage rate of digesta through the gastrointestinal tract (GIT).
The pig is also a reliable animal model for studying digestion in humans. There are extensive physiological similarities between pigs and humans in the major mechanisms involved in the regulation of feeding behaviour, FI and satiety (Roura et al., Reference Roura, Koopmans, Lallès, Le Huerou-Luron, De Jager, Schuurman and Val-Laillet2016). Although there is a parallel in human and pig digestive physiology and nutrition, in terms of desired outcomes they lie at the opposite end of the spectrum with targets of controlled BW and efficient weight gain, respectively. The aim of this review is to identify the factors affecting starch digestion rate in whole cereal grains, and how dietary fibres affect satiety, passage rate and FI. This understanding can serve as a basis for developing dietary regimes to control and achieve the desired opposite outcomes in humans and pigs (weight maintenance v. weight gain) by manipulating satiety and FI.
Role of starch in pig and human nutrition
Starch is the major macronutrient in whole-grain diets providing calories for normal energy metabolism in both humans and pigs. Starches from different grains are digested and absorbed at different rates and to different extents, depending on their physicochemical characteristics (Giuberti et al., Reference Giuberti, Gallo, Masoero, Ferraretto, Hoffman and Shaver2014; Taylor et al., Reference Taylor, Emmambux and Kruger2015). These physicochemical characteristics of starches can modulate postprandial metabolic response, which potentially affects FI, nutrient digestibility and hormone release associated with digestion. Starch from grains is digested in the small intestine (SI) to produce glucose. However, a fraction of this starch may escape digestion (resistant starch) and reach the large intestine (LI), where it is fermented, leading to the production of short-chain fatty acids (SCFA) (Lee et al., Reference Lee, Bello-Perez, Lin and Kim2013). The starch converted to glucose in the SI is more efficient for providing energy to pigs (and humans) compared to SCFA produced as a result of fermentation in the LI (Bosch et al., Reference Bosch, Borne and Gerrits2012). Therefore, improving and optimising starch digestion is an important focus of research in monogastric animal production for maximum feed efficiency and live weight gain in pigs.
Recent developments in starch digestion methods (in vitro)
The traditional method of classifying starches is based on the assignment of proportions of the starch that are rapidly digested (RDS), slowly digested (SDS) or resistant to digestion (RS) in vitro. This method has the advantage of being straightforward to carry out, but there are a number of drawbacks (Dhital et al., Reference Dhital, Warren, Butterworth, Ellis and Gidley2017). A recently developed method (Edwards et al., Reference Edwards, Grundy, Warren, Milligan, Butterworth and Ellis2014) was based on the realisation that the classification of starches as RDS, SDS and RS can be technically flawed as it does not take into account the digestion kinetics based on changes in the relative concentrations of amylase and starch as the digestion progresses. This method (Edwards et al., Reference Edwards, Grundy, Warren, Milligan, Butterworth and Ellis2014) suggests determining the starch digestion rate based on first-order kinetics behaviour of specific grain particle size fractions. However, this limits its usefulness to pig production systems because ground grains in pig (and human) nutrition, as a result of milling and/or mastication, contain a mixture of particle size fractions, and the residence time in the SI available for digestion varies depending on the transit or passage rate. A recent review (Dhital et al., Reference Dhital, Warren, Butterworth, Ellis and Gidley2017) of the mechanisms of starch digestion by alpha-amylase suggested that the classification of RS should be based on the interplay of enzyme digestion rate and small intestinal digesta passage rate. Therefore, a robust method is needed to determine the digestibility of ground grains irrespective of particle size, so that a link between in vitro and in vivo grain digestibility can be developed. Particle size is the main determinant of starch digestion rate in milled grains as the digestion by amylase is considered to be a surface-controlled enzyme diffusion process (Al-Rabadi et al., Reference Al-Rabadi, Gilbert and Gidley2009; Mahasukhonthachat et al., Reference Mahasukhonthachat, Sopade and Gidley2010). The apparent enzyme (amylase) diffusion rate, based on first-order kinetics analysis of starch digestion in milled grain size fractions, is proposed to be a useful approach to determine intrinsic grain digestibility as it is independent of particle size. Until recently, this had only been determined in a few grain samples (Al-Rabadi et al., Reference Al-Rabadi, Gilbert and Gidley2009; Mahasukhonthachat et al., Reference Mahasukhonthachat, Sopade and Gidley2010). However, a recent study (Ratanpaul et al., Reference Ratanpaul, Williams, Black and Gidley2018) showed that plotting the inverse of the first-order digestion rate coefficient against the square of particle size (Figure 1) gave linear plots for all of 16 diverse grain samples, consistent with a surface area-dependent rate-limiting step. The slope of this plot provides the diffusion coefficient for pure diffusion control, but as other surface area-dependent factors cannot be ruled out, we propose to designate this value as an apparent enzyme diffusion coefficient. This coefficient is a measure of the intrinsic digestibility of milled grains (i.e. independent of particle size), and can be used to investigate grain effects on amylase digestibility between species and as a function of growth conditions (Ratanpaul et al., Reference Ratanpaul, Williams, Black and Gidley2018).

Figure 1 Starch digestion in vitro for a sorghum sample (Waxy Isoline) with SEM: (a) Digestograms as a function of particle size, (b) first-order kinetics fit – rate coefficient k (h–1) for each fraction is given by the slope, (c) relationship between square of particle size (mm2) and inverse of rate coefficient, 1/k (h) – the slope gives the ADC (Ratanpaul et al., Reference Ratanpaul, Williams, Black and Gidley2018). The fit in (c) shows that the rate constant for digestion (Ki) at a particle size of siavg2 is determined by the diffusion coefficient D. This is consistent with an ‘outside-in’ surface erosion method of starch digestion from grain particles; the light (yellow) region represents digested and the dark (blue) represents undigested regions of a schematic particle after partial digestion. ADC=apparent diffusion coefficient.
Milled grain particle size and digestion rate
Food structure and particle size have critical roles in determining the rate of small intestinal starch digestion and consequent postprandial metabolism. Characterisation by electron microscopy and solid-state 13C NMR of undigested grain-containing digesta recovered from the ileum of pigs showed that some milled grain particles can escape gastric and SI digestion, maintaining their intact particulate and semi-crystalline structure (Gidley et al., Reference Gidley, Flanagan, Sharpe and Sopade2011). In contrast, isolated maize starch in the feed results in essentially complete digestion by the midpoint of the SI in pigs (Hasjim et al., Reference Hasjim, Lavau, Gidley and Gilbert2010). Light microscopy of ileal effluents collected from human ileostomy subjects, 4 h after consuming cooked wheat porridge (2 mm particles), showed that some starch granules encapsulated in endosperm cell walls survived digestion in the SI (Edwards et al., Reference Edwards, Grundy, Grassby, Vasilopoulou, Frost, Butterworth, Berry, Sanderson and Ellis2015). Human ileostomy participants consuming coarse (2 mm particles) wheat porridge showed lower postprandial concentrations of blood glucose and insulin than those consuming smooth (<2 mm particles) porridge (Edwards et al., Reference Edwards, Grundy, Grassby, Vasilopoulou, Frost, Butterworth, Berry, Sanderson and Ellis2015). Smaller particles are digested faster in the SI than larger particles, providing more energy for normal body function and growth. Milled grain particle size has a major impact on starch digestion rate as found from in vitro studies (Al-Rabadi et al., Reference Al-Rabadi, Gilbert and Gidley2009; Mahasukhonthachat et al., Reference Mahasukhonthachat, Sopade and Gidley2010; Ratanpaul et al., Reference Ratanpaul, Williams, Black and Gidley2018). Smaller particles lead to a greater extent of growth and feed efficiency in pigs (De Jong et al., Reference De Jong, DeRouchey, Tokach, Dritz, Goodband, Paulk, Woodworth, Jones and Stark2016; Al-Rabadi et al., Reference Al-Rabadi, Hosking, Torley, Williams, Bryden, Nielsen, Black and Gidley2017). Despite the known effects of milled grain particle size on starch digestion, it is still unknown if the particle size has the same effect on starch digestion when the grains are processed (e.g. heat or enzyme treatment) prior to grinding/milling. Excess BW gain in humans can be a direct result of excess intake of highly digestible foods that provide fast glucose release during digestion (Anderson and Woodend, Reference Anderson and Woodend2003). Particle size also affects other physiological functions of the gastrointestinal tract such as secretory function (Saqui-Salces et al., Reference Saqui-Salces, Luo, Urriola, Kerr and Shurson2017).
Grain digestion and satiety
The digestibility of feed and its effects on short-term satiety and FI suggest that two key factors are important: a) the rate of macronutrient (e.g. starch) digestion, which determines the site of digestion in the GIT with slower-digesting nutrients reaching the distal SI or the LI; and b) feedback control of total FI through modification of satiety through intestinal brakes when digestion is slow. Hormonal and physiological mechanisms involved in this feedback are discussed later, but here we summarise dietary approaches to modifying FI via satiety effects.
The control of FI through modification of satiety is possible with the inclusion of soluble dietary fibre or proteins in the feed consumed (Fiszman and Varela, Reference Fiszman and Varela2013). In rats, the intake of soluble dietary fibres, such as pectin (Adam et al., Reference Adam, Williams, Dalby, Garden, Thomson, Richardson, Gratz and Ross2014) or beta-glucan (Adam et al., Reference Adam, Williams, Garden, Thomson and Ross2015), increased, in a dose-dependent manner, postprandial blood concentration of satiety hormones and LI fermentation activity, and decreased FI, weight gain and adiposity in rats. In humans, soluble dietary fibre is seen as desirable as it slows down the rate of nutrient digestion (Scazzina et al., Reference Scazzina, Siebenhandl-Ehn and Pellegrini2013). In commercial pig production, in terms of feed efficiency, cereal dietary fibres such as beta-glucans and arabinoxylans are considered undesirable due to their negative effects on nutrient digestibility and FI (De Lange, Reference De Lange, Moughan, Verstegen and Visser-Reyneveld2000).
Consequences of incomplete starch digestion
Grains that are highly digestible lead to rapid increases in postprandial blood glucose concentrations and subsequently lead to increased insulin secretion compared to slower-digested foods (Scazzina et al., Reference Scazzina, Siebenhandl-Ehn and Pellegrini2013). Slow or incomplete digestion of starch, proteins and lipids has important consequences (Gidley, Reference Gidley2013) on:
1) insulin secretion, a component of carbohydrate metabolism and also involved in protein digestion, to regulate blood glucose levels. Insulin is the most potent anabolic hormone that facilitates the synthesis and storage of glycogen, lipids and proteins, and inhibits the breakdown and release of these stored macromolecules into the circulation. Insulin also enables the absorption of glucose, amino acids and fatty acids into cells (Saltiel and Kahn, Reference Saltiel and Kahn2001). The overstimulation of this glucose-regulating hormonal mechanism, over time, can lead to increased risk of type II diabetes;
2) ileal brake activation that takes place when digestion is slow and more nutrient uptake occurs towards the end of the SI, that is, the ileum (Maljaars et al., Reference Maljaars, Peters, Mela and Masclee2008); and
3) fermentation in the LI that occurs when starch and/or dietary fibre escapes gastric and SI digestion. Undigested and fermentable carbohydrates reaching the LI are fermented to produce SCFA. These SCFA are chemosensed by the FFAR2 receptor in the colon, leading to the secretion of appetite-suppressing gut peptide (PYY), reducing FI (Brooks et al., Reference Brooks, Viardot, Tsakmaki, Stolarczyk, Howard, Cani, Everard, Sleeth, Psichas, Anastasovskaj, Bell, Bell-Anderson, Mackay, Ghatei, Bloom, Frost and Bewick2017). PYY has been implicated in reducing the gastric emptying rate (GER) as shown by a comparative study (Nightingale et al., Reference Nightingale, Kamm, Van der Sijp, Ghatei, Bloom and Lennard-Jones1996) in human subjects with and without a colon. Human subjects with a colon had a higher colonic concentration of PYY and reduced GER after consuming pancakes and orange juice; and this effect was referred to as the ‘colonic brake’ (Nightingale et al., Reference Nightingale, Kamm, Van der Sijp, Ghatei, Bloom and Lennard-Jones1996). A recent study (Chambers et al., Reference Chambers, Viardot, Psichas, Morrison, Murphy, Zac-Varghese, MacDougall, Preston, Tedford, Finlayson, Blundell, Bell, Thomas, Mt-Isa, Ashby, Gibson, Kolida, Dhillo, Bloom, Morley, Clegg and Frost2015) involving the delivery of inulin-propionate ester to the colon in human subjects showed that propionic acid promoted postprandial secretion of PYY and GLP-1 and reduced energy intake. Of the various SCFA produced as a result of colonic fermentation of dietary fibre, propionic acid has the highest affinity for FFAR2 (Chambers et al., Reference Chambers, Viardot, Psichas, Morrison, Murphy, Zac-Varghese, MacDougall, Preston, Tedford, Finlayson, Blundell, Bell, Thomas, Mt-Isa, Ashby, Gibson, Kolida, Dhillo, Bloom, Morley, Clegg and Frost2015), a trigger for gut peptide secretion. In an experiment in pigs, a decreased insulin secretion after the consumption of rye bread was found to be associated with a higher absorption of butyrate generated by bacterial fermentation in the LI (Theil et al., Reference Theil, Jorgensen, Serena, Hendrickson and Knudsen2011). These findings highlight the importance of colonic fermentation and its end-products in influencing the effectiveness of satiety hormones. Both ileal and colonic brakes reduce the passage rate of digesta and FI (Black et al., Reference Black, Williams, Gidley, Torrallardona and Roura2009; Lee et al., Reference Lee, Bello-Perez, Lin and Kim2013).
Physiological factors affecting the rate of passage and feed intake
A wide array of factors affect FI in humans (Rolls, Reference Rolls2007) and pigs (Nyachoti et al., Reference Nyachoti, Zijlstra, De Lange and Patience2004; da Silva et al., Reference da Silva, van den Borne, Gerrits, Kemp and Bolhuis2012). In pigs, the main factors affecting FI are: (1) environmental factors: temperature, humidity and ventilation; (2) social factors: space allocation, feeder space, group size in pens, familiarity with other pigs and regrouping; (3) animal factors: health status, age, gender, physiological status and genetic effects; and (4) dietary factors: feed bulk and physical FI capacity, nutrient intake levels, dietary nutrient content and balance, feed additives, dietary contaminants, feed processing and ingredient type, feed form and presentation and availability of drinking water. In commercial pig production, all of these factors act together to affect FI. Although many of these factors have been studied individually, and their effect on FI quantified, the combined effect of all these factors is not well understood (Nyachoti et al., Reference Nyachoti, Zijlstra, De Lange and Patience2004). The focus here is to cover only those animal and dietary factors that physiologically affect the digestive processes and hence influence FI. Feed intake is likely to be controlled by two main physiological and biochemical processes: (1) rate of passage of digesta or transit time through the GIT (Black et al., Reference Black, Williams, Gidley, Torrallardona and Roura2009), and (2) activation of satiety and hunger centres in the brain as a result of meal consumption, absorption of digested nutrients and metabolite generation from subsequent biochemical processes (Nyachoti et al., Reference Nyachoti, Zijlstra, De Lange and Patience2004; Black et al., Reference Black, Williams, Gidley, Torrallardona and Roura2009).
Rate of passage of digesta as influenced by gastric emptying rate, and ileal and colonic brakes
Various studies of different monogastric animals, including pigs (Phillips et al., Reference Phillips, Phillips, Young and McQuitty1982), have suggested that FI is inversely related to transit time of digesta through the GIT (Black et al., Reference Black, Williams, Gidley, Torrallardona and Roura2009). However, the physiological mechanisms controlling FI through the rate of passage of digesta are not yet fully understood. A simplified schematic (Figure 2) of the monogastric GIT illustrates the effect of ileal and colonic brakes on the rate of digesta passage and FI.
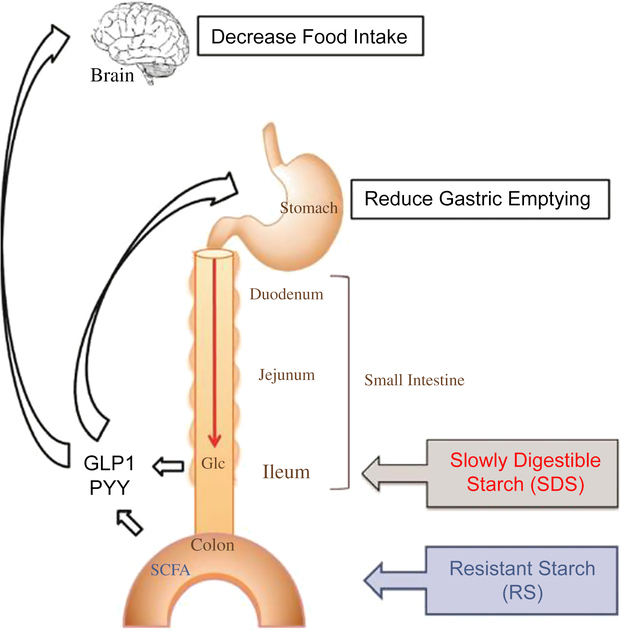
Figure 2 A schematic to illustrate the effect of ileal and colonic brakes caused by ileal Glc release and SCFA production from colonic fermentation on the rate of passage of digesta and food intake in monogastric animals. Glucagon-like peptide 1 and PYY are released from the ileum and colon, respectively, which control the gastric emptying rate and food intake. Glc=glucose; SCFA=short-chain fatty acids; GLP1=Glucagon-like peptide 1; PYY=peptide YY. Reproduced with permission from Lee et al. (Reference Lee, Bello-Perez, Lin and Kim2013).
Gastric distension and emptying rate
The stomach acts as a ‘holding tank’ for ingested feed and controls the flow of digesta through the pylorus to the duodenum. Therefore, gastric distension and emptying rate influence the rate of passage or transit time of digesta through the upper GIT. The presence of feed in the stomach causes initial distal gastric contractions and gastric emptying through the pyloric valve, which depends on the volume and subsequent gastric distension. Hence, an initial increase in the rate of passage is caused by volume effects rather than by nutrients (Black et al., Reference Black, Williams, Gidley, Torrallardona and Roura2009). Gastric distension caused by an increasing volume of food has the effect of sending satiety signals via the vagal nerve to the brain with a consequent reduced FI (Maljaars et al., Reference Maljaars, Peters and Masclee2007). However, the effects of gastric volume on satiety are only short-term and meal-related. While gastric satiety is largely volumetric, intestinal (duodenal) satiety is nutritional. Nutritional satiety signals act in synergy with gastric volumetric signals in controlling the GER (Powley and Phillips, Reference Powley and Phillips2004; Cummings and Overduin, Reference Cummings and Overduin2007).
Role of starch digestion rate, grain particle size and intact tissue structure
The presence of intact cellular structures from cereal-based foods inaccessible to mammalian amylases decreases the starch digestion rate (Scazzina et al., Reference Scazzina, Siebenhandl-Ehn and Pellegrini2013). Proteins can delay gastric emptying, though results have not always been consistent. Large grain particles delay gastric emptying in pigs as shown by barley ground through a 4.68-mm screen compared with a 1.56-mm one (Potkins et al., Reference Potkins, Lawrence and Thomlinson1991). Retropulsion and forceful grinding of large particles in the gastric antrum (Brown et al., Reference Brown, Schulze-Delrieu, Schrier and Abu-Yousef1993; Schulze, Reference Schulze2006) reduces the particle size before passage through the pylorus is possible.
The activation of ileal brake, as a result of undigested nutrients in the ileum, through mediators such as PYY, GLP-1 and vagal nerve stimulation (Maljaars et al., Reference Maljaars, Peters, Mela and Masclee2008) leads to a reduction in hunger and FI. The authors (Maljaars et al., Reference Maljaars, Peters, Mela and Masclee2008) argue that after ileal brake activation, enhanced gastric distension from delayed gastric emptying is most likely the cause of stimulation of satiety centres in the brain through hormonal and neural signals, which in turn lead to a reduction in hunger and FI. Several studies have indicated that SCFA in the ileum inhibit gastric motility and subsequently slow gastric emptying (Cherbut et al., Reference Cherbut, Aube, Blottiere and Galmiche1997). Short-chain fatty acids reaching the ileum from the colon as a result of colo-ileal reflux also inhibit gastric motility in pigs by a humoral pathway in which PYY is released (Cuche et al., Reference Cuche, Cuber and Malbert2000). Apart from lipids, ileal infusion of sucrose and casein also reduces FI in humans; and a reduction in GER and intestinal transit rate was observed concomitant with an increased concentration of cholecystokinin and PYY in the ileum, indicating the activation of the ileal brake (van Avesaat et al., Reference van Avesaat, Troost, Ripken, Hendriks and Masclee2015). There is no available data other than ileal infusion of isolated nutrients (Maljaars et al., Reference Maljaars, Peters and Masclee2007; Maljaars et al., Reference Maljaars, Peters, Mela and Masclee2008; van Avesaat et al., Reference van Avesaat, Troost, Ripken, Hendriks and Masclee2015) on the effects of ileal brake activation in humans.
A review (Black et al., Reference Black, Williams, Gidley, Torrallardona and Roura2009) explained that colonic fermentation of undigested carbohydrates and subsequent SCFA production induces a colonic brake, which reduces the passage rate of digesta, increases oesophageal sphincter tension, lowers gastric tone, decreases GER, reduces gastric and pancreatic secretions and reduces intestinal motility. The result of these effects of colonic brake is a slower passage of digesta through the GIT and reduced FI. The presence of nutrients in the distal ileum and colon, rather than in the proximal regions, has greater effects in decreasing GER and transit time through the SI, which consequently reduces FI.
It is apparent that there is more direct evidence of the colonic brake (Nightingale et al., Reference Nightingale, Kamm, Van der Sijp, Ghatei, Bloom and Lennard-Jones1996) than the ileal brake. Ideally, a direct measure of gastric and SI passage rates based on grains with widely varying digestibility would verify whether the undigested nutrients indeed reduce passage rate by triggering ileal and colonic brakes, without ileal or colonic infusion of nutrients or SCFA.
Hormonal control of feed intake
There are more than 20 different regulatory peptide hormones secreted by the GIT (Figure 3), the largest endocrine organ in the body. These hormones are released in response to the presence of nutrients in the gut and carry signals to the brain regarding the current energy balance. Most of these hormones are sensitive to the availability of nutrients in the GIT, and short-term feelings of hunger and satiety are consequences of their changing circulating levels (Murphy and Bloom, Reference Murphy and Bloom2006). Gut hormones and the adipose hormone leptin reduce FI, whereas ghrelin is the only peptide hormone secreted in the stomach, and increases appetite and induces feelings of hunger. Circulating ghrelin levels fall after a meal and increase on fasting (Murphy and Bloom, Reference Murphy and Bloom2006). Long-term energy homeostasis is regulated by circulating levels of insulin and leptin in proportion to recent nutrient intake and body adiposity. The short- and long-term energy-maintaining mechanisms work collaboratively such that insulin and leptin seem to determine the brain sensitivity to satiety, inducing effects of short-term peptide signals from the GIT (Havel, Reference Havel2001).
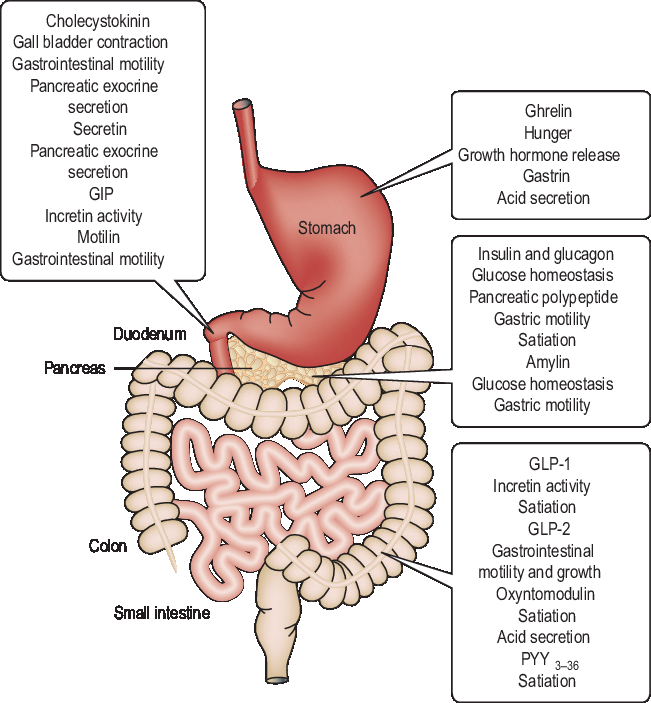
Figure 3 A schematic of the gastrointestinal tract in monogastric animals illustrating the sites where gut hormones are released and their major putative functions. GIT=gastrointestinal tract; GIP=gastric inhibitory polypeptide; GLP=glucagon-like peptide; PYY=peptide YY. Reproduced with permission from Murphy and Bloom (Reference Murphy and Bloom2006).
In humans, low GI foods reduce postprandial blood glucose and plasma insulin levels, stimulate gastric inhibitory polypeptide (GIP) secretions and exert a second meal effect of low postprandial glucose upon consumption of high GI foods (Jenkins et al., Reference Jenkins, Wolever, Taylor, Griffiths, Krzeminska, Lawrie, Bennett, Goff, Sarson and Bloom1982). The second meal effect is the blood glucose-lowering effect that a first meal has on the blood glucose level after consumption of a subsequent meal (Jenkins et al., Reference Jenkins, Wolever, Taylor, Griffiths, Krzeminska, Lawrie, Bennett, Goff, Sarson and Bloom1982). Carbohydrates in the upper GIT stimulate GIP release, and a reduction in GIP response indicates a decreased concentration of sugars available for absorption (Jenkins et al., Reference Jenkins, Wolever, Taylor, Griffiths, Krzeminska, Lawrie, Bennett, Goff, Sarson and Bloom1982). Slower gastric emptying (Lee et al., Reference Lee, Bello-Perez, Lin and Kim2013) after consumption of the second meal suggests that SCFA are involved in gastric motility. Slower gastric emptying directly reduces FI.
Interactions between starch digestion rate and feed intake
The interactions between macronutrient (particularly starch) digestion rate and FI in monogastric animals may offer opportunities to improve growth performance and economic benefits for the animal industry by manipulating satiety and FI for efficient feed utilisation and growth. This scenario runs in parallel for human nutrition, but in the opposite direction, where satiety can be manipulated by meal composition to regulate healthy BW and prevent or alleviate obesity.
Therapeutic drugs to control obesity do not target natural physiological satiety mechanisms and can have side effects. Mimicking natural satiety mechanisms by administering a combination of gut hormones may provide effective anti-obesity treatment (Wren and Bloom, Reference Wren and Bloom2007). The challenge is that the GIT is a complex organ with many hormones secreted from different locations in response to the presence of nutrients in a precise manner, which is difficult to mimic. The ability to control or manipulate the rate of passage of digesta may provide a natural way to induce and control satiation and satiety without having to administer gut hormones exogenously. Signals of satiation (the feeling of fullness) and satiety (the postprandial prevention of hunger onset) originate primarily from gastric distension and nutrient sensing in the intestine, respectively, activating neural and humoral pathways (Powley and Phillips, Reference Powley and Phillips2004; Cummings and Overduin, Reference Cummings and Overduin2007). Prolonged ileal brake activation, as demonstrated by ileal intubation and surgical studies, can lead to major weight loss in humans by reduction in hunger and FI impacting BW regulation (Maljaars et al., Reference Maljaars, Peters and Masclee2007). In contrast, ileal brake activation is expected to have negative effects on animal performance as it reduces FI, but is a relatively unexplored territory in monogastric animal nutrition. Finding ways to avoid ileal brake activation from intake of feed ingredients with defined digestibility is, therefore, an attractive target in order to maximise growth performance in pigs.
Ileal infusion of glucose in human subjects has shown enhancement of satiety and reduced energy intake (Shin et al., Reference Shin, Poppitt, McGill, Lo and Ingram2013). As both ileal and colonic brakes induce satiety and reduce FI (Shin et al., Reference Shin, Poppitt, McGill, Lo and Ingram2013), in order to alleviate obesity in humans, one approach is to structure starchy foods made from cereals so they can reach the ileum and colon undigested (Taylor et al., Reference Taylor, Emmambux and Kruger2015). In humans, insoluble fibre, from a source such as wheat bran, has been used to treat constipation (Muir et al., Reference Muir, Yeow, Keogh, Pizzey, Bird, Sharpe, O’Dea and Macrae2004), but has not been given much consideration as a tool for manipulating satiety in combination with other nutrients by virtue of its ability to stimulate the passage rate.
Physiological effects of fibre in the gastrointestinal tract in relation to starch digestion rate and feed intake
A number of studies (Livesey and Tagami, Reference Livesey and Tagami2009; Scazzina et al., Reference Scazzina, Siebenhandl-Ehn and Pellegrini2013) have supported the concept that soluble fibre that increases viscosity of digesta results in a low glycaemic response upon consumption of foods containing starch, due to slow GER and absorption of nutrients in the SI. The underlying mechanism is proposed to be inhibition of enzyme access to digesta in the stomach and SI. From a meta-analysis (Livesey and Tagami, Reference Livesey and Tagami2009), it has been proposed that soluble fibres which are non-viscous, such as resistant maltodextrin, can also attenuate glycaemic response when added to drinks and consumed with starchy foods. Some of the mechanisms suggested are slower GER, quicker transit of digesta to the distal SI where absorption may be less rapid and inhibition of digestive enzymes. The mixing of digesta in the stomach and SI increases the probability of enzyme–substrate binding, whereas viscosity of the fluid without any mixing hinders the enzyme–substrate complex formation required for digestion (Dhital et al., Reference Dhital, Dolan, Stokes and Gidley2014), as is the case for grain particle size effects (Figure 1).
The effects of dietary fibre on GER and rate of passage in the GIT are related to the volume and physical properties of gastric and intestinal contents. The GER is also controlled by biological feedback mechanisms operating in the SI through receptors sensitive to osmolality, acidity and particle size of the digesta (Potkins et al., Reference Potkins, Lawrence and Thomlinson1991). Wheat bran included in diets of humans (Lewis and Heaton, Reference Lewis and Heaton1997) or pigs (Wilfart et al., Reference Wilfart, Montagne, Simmins, Noblet and Milgen2007) reduced whole gut transit time. In a study with different soluble and insoluble fibres added to diets offered to human subjects, wheat bran showed the most potent effect of increasing the rate of passage, while soluble fibres showed the opposite effect (Jenkins et al., Reference Jenkins, Wolever, Leeds, Gassull, Haisman, Dilawari, Goff, Metz and Alberti1978). Pigs fed diets containing neutral detergent fibre (NDF) from different sources showed increased rate of passage through the GIT with increasing NDF levels (Stanogias and Pearcet, Reference Stanogias and Pearcet1985).
In pigs fed ad libitum, FI increases sufficiently with decreasing digestible energy content from addition of fibre, to maintain normal metabolism and homeostasis. This increase in FI occurs with increasing GIT capacity and changes the transit time of digesta. Eventually, a threshold point is reached beyond which the FI cannot be increased any further by additional fibre to compensate for the declining energy density. At that point, the energy intake required for normal metabolism falls (Black, Reference Black, Moughan, Verstegen and Visser-Reyneveld2000). At high fibre concentrations, the hydration and swelling capacity of pig feeds increases. The reduction in energy density and increase in feed bulk and digesta swelling in the stomach causes gastric distension and reduces FI in pigs (Kyriazakis and Emmans, Reference Kyriazakis and Emmans1995; Brachet et al., Reference Brachet, Arroyo, Bannelier, Cazals and Fortun-Lamothe2015). In humans with a different eating behaviour to pigs, postprandial gastric distension contributes to satiation in synergy with pre- and post-gastric nutrient stimuli as the volume of meals is not sufficient to cause excessive gastric distension (Ritter, Reference Ritter2004; Cummings and Overduin, Reference Cummings and Overduin2007).
A recent review (Capuano, Reference Capuano2017) on the physiological effects of dietary fibre in the human GIT highlights that there is much information on the physiological effects of soluble and fermentable fibre in the mouth, stomach, SI and LI. Both soluble and insoluble fibre can increase transit rate through the SI and LI to variable extents because of the enhanced effect of peristalsis on digesta with increased bulk and swelling through water absorption. The effects of fibre (soluble or insoluble) on the transit rate are well studied for the LI, whereas limited information is available for the effects of fibre on the transit rate in the SI (Wilfart et al., Reference Wilfart, Montagne, Simmins, Noblet and Milgen2007; Capuano, Reference Capuano2017), where digestion of most macronutrients takes place. Passage rate in the SI determines the extent and site of digestion (Lee et al., Reference Lee, Bello-Perez, Lin and Kim2013), and therefore the interplay of digestion rate and passage rate in the SI as influenced by fibre needs to be explored.
The rate of passage of digesta is a critical physiological factor that determines FI through the GIT and rate of digestion. During the postprandial period, peptides released from the stomach and duodenum, and mild distension of the GIT, stimulate the rate of passage (Black et al., Reference Black, Williams, Gidley, Torrallardona and Roura2009). As undigested nutrients trigger ileal and colonic brakes (Black et al., Reference Black, Williams, Gidley, Torrallardona and Roura2009; Lee et al., Reference Lee, Bello-Perez, Lin and Kim2013), a decline in passage rate increases the extent of digestion by increasing the time available for enzymatic digestion in the SI, but also reduces FI through delayed gastric emptying. The negative effect of ileal brake on FI may be compensated for by inclusion of higher amounts of indigestible fibre in the diet to increase FI by stimulating the passage rate (Campbell, Reference Campbell1988; Black et al., Reference Black, Williams, Gidley, Torrallardona and Roura2009).
An evaluation (Vicente et al., Reference Vicente, Valencia, Pérez-Serrano, Lázaro and Mateos2008) of the effects of feeding cooked corn and cooked rice to pigs showed that the pigs (from 25 until 53 days of age) fed cooked rice had 23% higher FI, 29% faster growth rate and 5% more efficient feed conversion ratio than pigs fed cooked corn only. A similar experiment (Mateos et al., Reference Mateos, Martin, Latorre, Vicente and Lazaro2006) investigated the effects of cooked maize and cooked rice with addition of cooked and expanded oat hulls at levels of 20 and 40 g/kg in the diet on productive performance of piglets weaned at 21 days till 54 days of age. Addition of oat hulls reduced FI and average daily weight gain in pigs fed diets based on maize, but increased both FI and average daily weight gain for diets based on rice. This may be due to higher indigestible fibre levels increasing the rate of digesta passage, leading to more undigested maize starch ending up in the distal intestine and activating the ileal brake. On the other hand, more highly digestible rice starch would have little chance to escape digestion in the SI and reach the distal intestine even with an increased passage rate, and therefore would not be able to activate the ileal brake (Black et al., Reference Black, Williams, Gidley, Torrallardona and Roura2009). Another study (Gerritsen et al., Reference Gerritsen, van der Aar and Molist2012) on 28-day-old piglets found that the addition of 15% indigestible fibre consisting of oat hulls and wheat straw to a normal cereal diet (25% barley, 20% wheat and 20% corn) increased FI and weight gain in piglets during the first 14-day post-weaning period.
A critical evaluation of the findings of the aforementioned studies implies that indigestible fibre can: (1) increase the negative effect of slowly digestible cereal grain fractions on FI, by activating the ileal brake sooner, and (2) enhance FI of highly digestible cereal grain fractions by stimulating the passage rate of digesta and maximising digestion before the ileal brake has a chance to act, since most nutrients are digested before reaching the ileum.
The effects of fibre on the GIT passage rate in pigs have been investigated (Jorgensen et al., Reference Jorgensen, Lindberg and Andersson1997; Wilfart et al., Reference Wilfart, Montagne, Simmins, Noblet and Milgen2007; da Silva et al., Reference da Silva, van den Borne, Gerrits, Kemp and Bolhuis2012). However, it is difficult to compare the findings, as these were based on diets containing different grains and fibres. In addition, the indigestible markers used to determine passage rates were also mostly different. It becomes complicated to interpret results across different studies when different experimental strategies and feeding regimes are used. Moreover, no attempts seem to have been made to investigate whether macronutrient digestion rates influence passage rate.
Fibre, both soluble and insoluble, increases passage rate through the SI and LI. A higher (268 g/kg) proportion of dietary fibre (pea fibre and pectin) fed to pigs increased the passage rate, measured at the end of ileum, by five to six times compared with low-fibre diets (59 g/kg), and this was attributed to the water-holding capacity of the dietary fibre used in the diets (Jorgensen et al., Reference Jorgensen, Lindberg and Andersson1997). An important study (Wilfart et al., Reference Wilfart, Montagne, Simmins, Noblet and Milgen2007) with double proximal duodenal and distal ileal cannulation of pigs investigated the effects of insoluble fibre from wheat bran (0%, 20% and 40%) on mean retention time in different segments of the GIT of pigs (~33 kg) and reported that mean retention time in the stomach, SI and LI was 1, 4, and 38 h, respectively. Higher insoluble fibre content reduced mean retention time of both solid and liquid phases in both the SI and LI, but had no effect on the GER. However, MRT of the liquid phase in the LI was 4 to 8 h less than that of the solid phase. A major limitation of the study was that pigs were fed six equal rations at 4-h intervals on the day of digesta collection, and individual meal size might not have been sufficient to fill the stomach and cause any gastric distension. This could explain their finding that despite the high level of wheat bran up to 40% in the diet, there was no effect on GER.
Transit time also depends on the length of the intestinal tract, which increases with age. Mean retention time through the GIT, nutrient and fibre digestibility all increase with the age of pigs (Le Goff et al., Reference Le Goff, Van Milgen and Noblet2002; Kim et al., Reference Kim, Lindemann, Cromwell, Balfagon and Agudelo2007), as longer retention time improves nutrient digestibility. A study (Solà-Oriol et al., Reference Solà-Oriol, Torrallardona and Gasa2010) investigating the effect of wheat bran (13%) offered to pigs with white rice or oats found a faster passage rate for oats. The authors concluded that cereal with high fibre content, such as oats, increased passage rate. However, the authors did not characterise grain digestibility or measure ileal digestibility of nutrients. The diets (Solà-Oriol et al., Reference Solà-Oriol, Torrallardona and Gasa2010) contained 60% grains (rice or oats), wheat bran (13%) and soybean meal (20%). Grains are (partially) digested in the SI depending on the particle size and intrinsic grain digestibility. Undigested grains reaching the LI may ferment and reduce the passage rate by triggering colonic brake, as discussed earlier. Wheat bran and soybean meal are also at least partially fermentable. It is important to consider individual ingredient fermentability before formulating diets intended for passage rate studies.
Another challenge is that passage rate studies (Albin et al., Reference Albin, Wubben, Smiricky and Gabert2001; Solà-Oriol et al., Reference Solà-Oriol, Torrallardona and Gasa2010) are usually based on rationed rather than ad libitum feeding. This approach may not allow true manifestation of physiological mechanisms controlling passage rate and FI, as FI also depends on satiety signals arising from the stomach (Powley and Phillips, Reference Powley and Phillips2004; Cummings and Overduin, Reference Cummings and Overduin2007). Ad libitum feeding eliminates external controls that stop pigs from eating while pigs may be hungry between rationed meals.
In an ad libitum study (Adam et al., Reference Adam, Williams, Dalby, Garden, Thomson, Richardson, Gratz and Ross2014), rats were fed 5% cellulose and 10% of either cellulose, fructo-oligosaccharides, oat beta-glucan or apple pectin added to their normal diets. It was found that rats on a diet with 10% cellulose had the highest cumulative FI followed closely by the 5% cellulose diet. FI and weight gain was significantly higher in rats on cellulose diets than on soluble fibre diets. Insoluble fibres from different sources have different functional effects in the gut. Wheat bran increased the rate of passage of digesta in pigs (Stanogias and Pearcet, Reference Stanogias and Pearcet1985; Wilfart et al., Reference Wilfart, Montagne, Simmins, Noblet and Milgen2007), and can also shift the site of fermentation of indigestible carbohydrates from caecum and proximal to distal colon (Govers et al., Reference Govers, Gannon, Dunshea, Gibson and Muir1999). In an investigation in humans it was found that wheat bran increased digesta transit rate, and coarse bran was more effective at increasing transit rate than finely ground bran (Heller et al., Reference Heller, Hackler, Rivers, Van Soest, Roe, Lewis and Robertson1980). Two investigations (Mateos et al., Reference Mateos, Martin, Latorre, Vicente and Lazaro2006; Gerritsen et al., Reference Gerritsen, van der Aar and Molist2012) have implicated oat hulls as a promising source of indigestible fibre, which perhaps could be used to increase passage rate and FI to derive maximum performance in pigs. Due to the positive effects, though slight, of pelleting high-fibre diets on nutrient digestibility, the dietary fibre content in pig diets is expected to increase in the near future (Le Gall et al., Reference Le Gall, Warpechowski, Jaguelin-Peyraud and Noblet2009). Therefore, the effects of high-fibre diets on FI and growth performance deserve new studies with different fibre sources.
Conclusions and gaps in current knowledge
• Macronutrient (e.g. starch) digestion rate determines the site of digestion throughout the GIT, together with the digesta passage rate. Starch is the major nutrient that provides energy for metabolism, energy homeostasis and growth. The enzyme diffusion rate of milled grain particles is the main determinant of the digestion rate of starch and, in part, determines the site of digestion in the GIT. To understand the mechanistic cause-and-effect relationships between digestion behaviour and these benefits over time, there is a need to understand diffusion behaviour of amylolytic enzymes, digestion kinetics of starch and release of glucose from whole grains. Knowing the proportion of starch fermented in the LI allows calculation of the proportion of starch energy not available for metabolism because of heat of fermentation, methane, lower use of SCFA than glucose and microbes/microbial products excreted in faeces.
• Dietary fibre, both soluble and insoluble, affects the digestion of macronutrients (starch, proteins and lipids). Both soluble and insoluble fibres increase passage rate through the SI and LI. However, there is less information on the rate of passage in the SI compared with the LI. Partially or undigested nutrients reaching the distal SI trigger ileal and colonic brakes, and are expected to induce satiety by neural and humoral mechanisms leading to reduced FI. The effects of the interplay of macronutrient digestion rate and passage rate in the SI have not been investigated in relation to the effects on FI. Fibre has the potential to influence the site of digestion of macronutrients in the SI, by virtue of its effects on passage rate. The prevalence of cereal grains in human and pig nutrition, and a need for increased use of fibre in their diets, provides a compelling reason to investigate and understand the interactions of grain digestion rate and fibre, and their effects on passage rate in relation to FI.
• Many studies on passage rate in pigs are based on rationed feeding. In order to eliminate the external control of FI, it is important to investigate FI in relation to passage rate based on ad libitum feeding. This will allow assessment of satiety and under which conditions FI may be reduced.
• There is limited information on insoluble and non-fermentable fibres originating from sources such as cereal hulls, in relation to GIT passage rate. Evidence of the effects of (partly fermentable) wheat bran on passage rate supports the idea that insoluble fibres can influence passage rate. A next step is to investigate if largely insoluble and non-fermentable fibre can be added to grain-based diets to achieve a desired change in the passage rate to manipulate satiety.
Acknowledgements
Funding was provided by the Pork Co-operative Research Centre and the Australian Research Council Centre of Excellence in Plant Cell Walls (CE110001007).
Michael Gidley, 0000-0002-8372-4527
Declaration of interest
None.
Ethics statement
No approval by an ethics committee was required for this review.
Software and data repository resources
None of the data were deposited in an official repository.