Introduction
In mammals, threonine is an essential amino acid (AA). In pigs, its supply by plant proteins is often below the requirements for maintenance and growth. Indeed, compared with the other essential amino acids, threonine requirements for maintenance are high (Fuller et al., Reference Fuller, McWilliam, Wang and Giles1989) and the intestine seems to contribute extensively to this requirement. In orally fed piglets, threonine requirement, evaluated by the indicator amino acid oxidation technique, is twice as much as that of parenterally fed piglets (Bertolo et al., Reference Bertolo, Chen, Law, Pencharz and Ball1998). Measurement of tissue balance in catheterised animals associated with tracer infusion confirms that dietary threonine is massively extracted by the portal-drained viscera (small and large intestines, pancreas, spleen and stomach). For instance, Stoll et al. (Reference Stoll, Henry, Reeds, Yu, Jahoor and Burrin1998) and Le Floc’h and Sève (Reference Le Floc’h and Sève2005) showed that less than 50% of labelled threonine newly infused in the lumen is recovered in the portal vein of continuously fed piglets. These data underline the importance of dietary threonine metabolism in the visceral tissues involved in its absorption, i.e. the small intestine. Until now, the metabolic fate of luminal threonine extracted by the intestine has remained unclear. In pigs, threonine oxidation mainly occurs through the l-threonine-3-deshydrogenase (TDG) pathway to give glycine (Ballevre et al., Reference Ballevre, Cadenhead, Calder, Rees, Lobley, Fuller and Garlick1990). Whereas TDG was present in liver and pancreas, no TDG enzymatic activity was detected in the small intestine, colon and associated lymphatic nodes (Le Floc’h et al., Reference Le Floc’h, Thibault and Sève1997). This result was confirmed using l-[1-13C] threonine continuous infusion (Le Floc’h and Sève, Reference Le Floc’h and Sève2005). Therefore, the high rate of threonine extraction by the intestine could not be explained by threonine oxidation through the TDG pathway. Otherwise threonine utilisation by the intestine is often associated with mucin synthesis (Faure et al., Reference Faure, Moënnoz, Montignon, Mettraux, Breuille and Ballèvre2005; Law et al., Reference Law, Bertolo, Adjiri-Awere, Pencharz and Ball2007). Indeed, threonine was found in high concentrations in the ileal endogenous protein losses (Hess et al., Reference Hess and Sève1999), particularly in mucins in which threonine represents 16% of total AAs (Lien et al., Reference Lien, Sauer, Mosenthin, Souffrant and Dugan1997).
The issue of threonine utilisation and metabolism in the gut is still open to discussion. We hypothesise that massive threonine extraction by the gut is essential for maintaining its structural and functional integrity. In this preliminary study, we compared intestinal development and growth performance of early-weaned piglets fed either a control well-balanced (C) or a low-threonine (LT) diet. At this age, small intestine is immature and grows rapidly. So effects of an LT diet on intestinal growth might be more important in early-weaned piglets than in older animals. We investigated descriptive parameters of intestine, both structural, such as weight parameters and morphology, and functional, such as mucosal enzyme activities. Modifications of these criteria were previously found to be associated with developmental alteration, for example, at weaning (Marion et al., Reference Marion, Biernat, Thomas, Savary, Lebreton, Zabielski, Le Huërou-Luron and Le Dividich2002; Montagne et al., Reference Montagne, Boudry, Favier, Le Huërou-Luron, Lallès and Sève2007) or in total parenteral feeding (Goldstein et al., Reference Goldstein, Hebiguchi, Luk, Taqi, Guilarte, Franklin, Niemec and Dudgeon1985; Adeola et al., Reference Adeola, Wykes, Ball and Pencharz1995).
Material and methods
Animals and feeding
The experiment was conducted with six pairs of littermate Piétrain × (Landrace × Large White) piglets from the experimental herd of INRA (Saint-Gilles). Piglets were weaned at 7 days of age, and within each litter, pairs were constituted on a body weight (BW; 2.5 ± 0.06 kg) basis. At weaning (day 0), piglets were fitted with a jugular catheter under general anaesthesia and then transferred into individual stainless-steel cage. Piglets were housed in a room maintained at 30°C.
Within each pair, one piglet received a C diet (C group) and the other one an LT diet (LT group) for 2 weeks. The composition of the experimental diets is presented in Table 1. Protein was supplied by skimmed-milk powder and a soluble fish protein concentrate. Those raw materials set the basal threonine level in both diets. A free AA mixture was added to match the ideal protein pattern as proposed by Chung and Baker (Reference Chung and Baker1992) for weaning piglets. Free threonine was added only in the C diet. The crude protein content of the LT diet was adjusted by addition of aspartic acid. Diets provided 250 g of protein (N × 6.25) and 15 MJ of digestible energy (DE) per kg. Dietary AA concentration was measured by high-performance liquid chromatography (HPLC Alliance System 2696, Waters Corporation, Milford, MA, USA) after chloridric acid hydrolysis of protein. Threonine content was 9.3 g/kg in the C diet and 6.5 g/kg in the LT diet.
Table 1 Ingredients and nutritional values of the experimental diets

†Providing the following amount of free amino acids (g/kg diet): l-lysine HCl, 3.53; l-tryptophane, 0.85; l-leucine, 1.86; l-isoleucine, 1.35; l-valine, 1.39; l-phénylalanine, 1.42; l-glutamate monoNa/A. glutamique (50/50), 35.3; glycine, 9.2.
‡Providing the following amount of vitamins and minerals (per kg diet): Ca, 1.82 g; Fe, 200 mg; Cu, 40 mg; Zn, 200 mg; Mn, 80 mg; Co, 4 mg; Se, 0.6 mg; I, 2 mg; vitamin A, 30 000 IU; vitamin D3, 6000 IU; vitamin E, 80 IU; vitamin B1, 4 mg; vitamin B2, 20 mg; panthotenic acid, 30 mg; vitamin B6, 20 mg; vitamin B12, 0.1 mg; vitamin PP, 60 mg; folic acid, 4 mg; vitamin K3, 4 mg; biotin, 0.4 mg; choline, 1600 mg; vitamin C, 200 mg.
The meals were offered in liquid form by mixing the air-dried diet with warm water (1 : 2). During the first 3 days of the experiment, the daily feed allowance was gradually increased and was delivered in five meals (0700 h, 1100 h, 1400 h, 1700 h and 2100 h). From day 3, C piglets were pair-fed the intake of the LT piglets. From this time, piglets received their daily allowance (mean value: 58 ± 1.6 g/kg of metabolic weight per day) in four equal portions, provided at 0700, 1100, 1600 and 2100 h.
Measurements and blood sampling
At days 4, 6, 8, 11 and 13, piglets were weighed and 5 ml of blood was sampled from the jugular catheter 3 h after the first morning meal. Blood samples were collected in heparinised tubes, chilled on ice and centrifuged at 3000 × g for 15 min at 4°C. Plasma aliquots were stored at −20°C until analysis.
For determination of plasma AA concentrations, plasma samples were deproteinised in a sulphosalicylic acid solution (60 g/l) containing norvaline as an internal standard. Separation of AAs was performed by ion exchange liquid chromatography, using the Biotronik LC 5001 analyser (Biotronik, Pusheim Bahnhof, Germany) according to the method of Moore and Stein (Reference Moore and Stein1954).
Plasma haptoglobin, a major acute phase protein in pig, was used as an indicator of the piglet inflammatory response (Eckersall et al., Reference Eckersall, Saini and McComb1996) and measured by a colorimetric assay (Phase Haptoglobin Assay, Tridelta Development Ltd., Ireland).
Slaughter procedure
After 2 weeks of treatment (day 14) and 3 h after the morning meal, piglets were electrically stunned and immediately sacrificed by exsanguination. The small intestine, from the ligament of Treitz to the ileocoecal junction, and the large intestine were quickly removed, weighed and measured. The small intestine was divided into three parts of equal length corresponding to the proximal and the distal jejunum and the ileum. The large intestine was divided into two equal parts and identified as proximal and distal colon. Each part of the small intestine and the colon were emptied, rinsed with water and weighed. A segment of about 70 cm long was taken in the middle of each segment. Mucosa/muscularis weight ratio was measured after mucosa scraping of a 50-cm segment. Additional mucosa from a 10-cm-long segment was scraped and frozen in liquid nitrogen for enzymatic analysis. Other 3-cm-long segments were sampled and immediately fixed in phosphate-buffered formalin (10%, pH 7.6) for histological measurements and morphometry. The weights of stomach, spleen and pancreas were also recorded.
Small intestine morphology
After fixation in phosphate-buffered formalin during 24 h at 4°C, samples were rinsed with ethanol : water (3 : 1, vol/vol). Then they were stained with Schiff’reagent after dehydratation according to the technique of Goodlad et al. (Reference Goodlad, Levi, Lee, Mandir, Hodgson and Wright1991). Bands of villus–crypt units were microdissected and mounted on a glass slide in acetic acid (45%). Villus height and crypt depth were measured under blind conditions using an optical microscope (Eclipse E400, Nikon, France), a camera (digital camera DXM1200, Nikon, France) and an image analyser (Lucia software, Laboratory Imaging, Prague, Czech Republic). Means values were determined on at least 30 villi and 20 crypts for each preparation.
Intestinal goblet cell histochemistry
Total numbers of mucin-containing goblet cells were counted in the ileum and the proximal colon. Segments from the ileum and from the proximal colon were kept in formalin for 48 h at 4°C. Then, they were dehydrated and embedded in paraffin. For all measurements, serial sections of 5 μm thickness were deparaffined and rehydrated just before use. Slides were stained with Alcian Blue 8 GX at pH 2.5 and periodic acid Schiff (AB/PAS) for total mucin. Coloration was performed in one batch in order to minimise differences in technical manipulation. Mucin-containing cells were counted in 20 crypts and a minimum of 40 villi per sample using an optical microscope (Eclipse E400, Nikon, France), a camera (digital camera DXM1200, Nikon, France) and an analyser (ImageJ).
Intestinal enzymes analysis
The mucosa from each segment was homogenised in water according to a 1 : 5 ratio (g/ml). The homogenate was centrifuged at 3000 r.p.m., at 4°C for 5 min. Protein content and enzyme activities were analysed on the supernatant. Protein content was measured by the method of Lowry et al. (Reference Lowry, Rosebrough, Farr and Randall1951) using BSA as a standard. Lactase, maltase and aminopeptidase N were measured according to the methods described by Marion et al. (Reference Marion, Petersen, Romé, Thomas, Sangild, Le Dividich and Le Huërou-Luron2005). Enzyme activities were expressed as the amount of substrate hydrolysed in nmol/min and per mg of protein.
Statistical analysis
Analysis of variance was performed using general linear model procedure of Statistical Analysis Systems Institute (2000). For growth, average daily feed intake, morphometry, histology and enzyme activities, the effect of pair and dietary threonine supply was tested using the residual variation between piglets as the error term. For plasma AA concentrations, feed intake was used as a covariate but it was not significant and was removed from the analysis. Then, the statistical model included the effect of pair, dietary treatment and day of sampling. The effects of pair and dietary threonine supply were tested using the residual variation between piglets as the error. At each day, dietary threonine supply effect was evaluated by pre-planned contrasts; at day 4, 6, 8, 11 and 13, the difference in AA concentrations between C and LT groups was compared with the difference calculated at day 0. The results are presented as least-square means (lsmeans) ± standard error (s.e.). Contrasts and differences between lsmeans were declared significant at P < 0.05.
Results
Food intake and growth performance
To minimise the stress of weaning, the diets were formulated to be highly digestible and were delivered every 4 days in liquid form. Piglets did not present any signs of clinical diseases and no diarrhoea was observed during the experimental period. Plasma haptoglobin did not differ between the two groups (1.37 v. 1.19 ± 0.38 mg/ml for C and LT piglets, respectively). The very first days after weaning, piglets readily consumed their daily feed allowance. However, by the end of the 1st week (data not showed), we observed feed refusals in C piglets fed at the intake level of LT piglets. Therefore, rationing of LT piglets was adjusted to feed consumption of C piglets on the days before. Finally, the average feed and net energy intakes were not significantly different between LT and C piglets (Table 2). However, because of the slight difference in feed intake between groups, dietary threonine intake was reduced by 25% in LT piglets compared with C piglets instead of the expected 30%: LT piglets consumed 0.39 g/kg BW0.75 per day and the C piglets 0.52 g/kg BW0.75 per day (P < 0.001).
Table 2 Growth performance and feed intake in early-weaned piglets pair-fed either a control well-balanced threonine diet or a low-threonine diet for 2 weeks (least-square means and standard error; n = 6 piglets per treatment)

†C = control diet (9.3 g threonine/kg of diet); LT = low-threonine diet (6.5 g threonine/kg of diet).
***P < 0.001.
Body weights at weaning and slaughter, weight gain and feed conversion ratio (Table 2) were not significantly different between treatments.
Plasma free AA
Diet × time interaction was significant for threonine (P < 0.0001), glutamine (P < 0.001), citrulline (P < 0.01) and ornithine (P < 0.05). As illustrated by Figure 1, plasma threonine concentration decreased from day 4 (89 ± 30 nmol/ml) to the end of the trial (38 ± 29 nmol/ml) in LT piglets (P < 0.0001) whereas it remained constant in C piglets during the 2 weeks (356 ± 12 nmol/ml; P > 0.05).

Figure 1 Evolution of plasma free threonine (nmol/ml) in early-weaned piglets pair-fed either a control well-balanced threonine diet (C: 9.3 g threonine/kg of diet) or a low-threonine diet (LT: 6.5 g threonine/kg of diet) for 2 weeks.
Plasma free AAs are presented in Table 3. The effect of time was significant for most AAs except for glutamic acid. The time response of plasma free AAs was characterised by two phases: firstly, plasma AA concentrations varied during the 1st week, then remained constant during the 2nd week. This was probably due to the transition between sow milk and the experimental weaning diets. On the overall experimental period, the effect of dietary threonine was significant on plasma concentrations of leucine, isoleucine (P < 0.05), lysine, methionine and phenylalanine (P < 0.01) for essential AAs and of serine, cysteine (P < 0.05), alanine, glutamine, asparagine, tyrosine and citrulline (P < 0.01) for non-essential AAs. For these AAs, plasma concentrations were significantly lower in LT compared with C piglets. At each day, the difference in AA concentrations between C and LT groups was compared with the difference calculated at day 0. On day 4, for methionine, serine (P < 0.05), ornithine, citrulline (P < 0.01), glutamine and threonine (P < 0.001), differences to day 0 were significantly higher in LT piglets than in C piglets. The same effect was noticed on day 6 for aspartic acid (P < 0.05) and threonine (P < 0.001). On day 8, 11 and 13, threonine (Figure 1) was the only AA for which the effect of diet on this difference (P < 0.001) remained significant.
Table 3 Plasma free amino acids (nmol/ml) in early-weaned piglets pair-fed either a control well-balanced threonine diet or a low-threonine diet for 2 weeks (least-square means and standard error; n = 6 piglets per treatment)
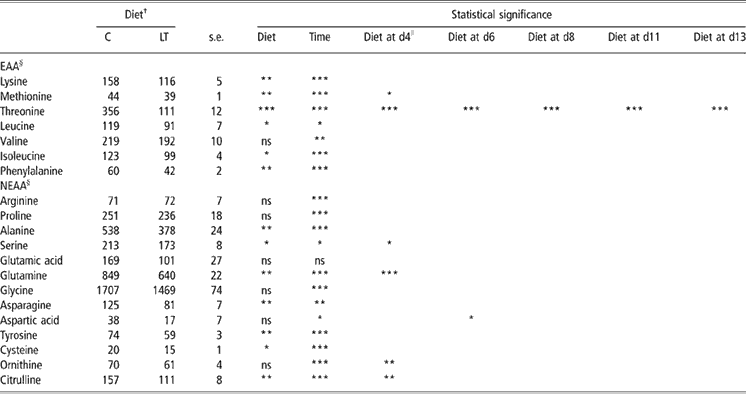
†C = control diet (9.3 g threonine/kg of diet); LT = low-threonine diet (6.5 g threonine/kg of diet).
*P < 0.05; **P < 0.01; ***P < 0.001.
§Data are least-square means of all the time points for C and LT ± s.e. (standard error).
∥Dietary threonine supply effect at different time was evaluated by pre-planned contrasts involving comparisons of differences between weaning (day 0) and the following days (day 4, 6, 8, 11 and 13).
Digestive organ development and structure
Dietary threonine supply significantly modified neither small and large intestine total weights and lengths, nor the weights and the mucosa/muscularis weight ratios of the different intestinal parts. The weights of stomach, spleen and pancreas were not altered by the dietary threonine supply (Table 4).
Table 4 Small and large intestine parameters in early-weaned piglets pair-fed either a control well-balanced threonine diet or a low-threonine diet for 2 weeks (least-square means and standard error; n = 6 piglets per treatment)

†C = control diet (9.3 g threonine/kg of diet); LT = low-threonine diet (6.5 g threonine/kg of diet).
*P < 0.05; **P < 0.01; ***P < 0.001.
Low threonine supply did not alter significantly crypt and villous architecture in the proximal and distal jejunum (Table 5). In the ileum, villi heights were 37% lower in the LT piglets than in the C piglets (P < 0.05; Figure 2) but villi width did not differ significantly between the two groups. Similarly, ileum crypt widths were lower in ileum of LT than in C piglets (P < 0.05) and the same trend was noticed for depths (P < 0.10). Consequently, these alterations of ileum structure led to a decrease in the villus height/crypt depth ratio (P < 0.05). In the proximal colon, none of these parameters was altered significantly (Table 5).
Table 5 Intestinal morphometry in early-weaned piglets pair-fed either a control well-balanced threonine diet or a low-threonine diet for 2 weeks (least-square means and standard error; n = 6 piglets per treatment)

†C = control diet (9.3 g threonine/kg of diet); LT = low-threonine diet (6.5 g threonine/kg of diet).
+ = Approaching significance, P < 0.1; *P < 0.05.

Figure 2 Comparison of number of mucin-containing goblets cells in the ileum of early-weaned piglets pair-fed either a control well-balanced threonine diet (C: 9.3 g threonine/kg of diet) or a low-threonine diet (LT: 6.5 g threonine/kg of diet) for 2 weeks. Slides were purple stained with Alcian Blue 8 GX at pH 2.5 and periodic acid Schiff (AB/PAS) for total mucin. Representative photomicrographs are shown for ileal tissues obtained from C piglets (a) and LT piglets (b). Original magnification 150×.
Digestive enzyme activities
We found no significant difference between C and LT groups for maltase- and lactase-specific activities measured in the different sites of the small intestine (Table 6). Low dietary threonine supply did not affect the jejunal aminopeptidase nitrogen (N)-specific activity. However, aminopeptidase N-specific activity was reduced by 28% in the ileum of the piglets fed the LT compared with the C diet (P < 0.05). Contrary to lactase and maltase, this activity is usually higher in the distal part of the intestine (Fan et al., Reference Fan, Stoll, Burrin and Jiang2001).
Table 6 Aminopeptidase nitrogen (N)-, lactase- and maltase-specific activities in different parts of the small intestine in early-weaned piglets pair-fed either a control well-balanced threonine or a low-threonine diet for 2 weeks (least-square means and standard error; n = 6 piglets per treatment)

†C = control diet (9.3 g threonine/kg of diet); LT = low-threonine diet (6.5 g threonine/kg of diet).
*P < 0.05.
Goblet cell histochemistry
The total numbers of goblet cells per villus and per crypt in the ileum (Figure 2) and per crypt in the proximal colon were not significantly affected by dietary threonine intake (Table 7) . Moreover, piglets fed the LT diet did not show any modifications in the distribution of mucin-containing goblet cells along the crypt–villus axis. Respectively 42%, 31% and 27% of mucin-containing goblet cells are present in the first, second and last third along the crypt–villus axis.
Table 7 Number of total mucin-containing goblet cells per villous and crypt in the ileum and in the proximal large intestine of early-weaned piglets pair-fed either a control well-balanced threonine diet or a low-threonine diet for 2 weeks (least-square means and standard error; n = 6 piglets per treatment)

†C = control diet (9.3 g threonine/kg of diet); LT = low-threonine diet (6.5 g threonine/kg of diet).
There were no significant differences (P > 0.05).
Discussion
Usually, ingestion of a diet lacking or deficient in an essential AA induces a decrease in feed intake (Peng et al., Reference Peng, Tews and Harper1972). For this reason, pair-feeding was first adjusted on the basis of feed consumed by LT piglets. However, LT piglets did not show feed refusals and their feed intake tended to be higher than that of C piglets (see Results section). This could indicate that piglets consuming a diet slightly deficient in threonine increase their feed intake in order to meet more closely their daily requirement. This kind of response was already noticed and discussed by Henry (Reference Henry1985) in growing pigs. In the present study, the level of dietary threonine supply modified neither daily weight gain nor feed efficiency. This lack of effect of threonine deficiency on growth was also observed in early-weaned piglets fed a diet supplying only 17% of threonine requirements for a short period of time (Law et al., Reference Law, Bertolo, Adjiri-Awere, Pencharz and Ball2007). This result was quite unexpected since several studies showed that low dietary threonine supply decreases growth of weaning piglets (Sewell et al., Reference Sewell, Loosli, Maynard, Williams and Sheffy1953; Adeola et al., Reference Adeola, Lawrence and Cline1994) and growing pigs (Le Floc’h et al., Reference Le Floc’h, Sève and Henry1994; Schutte et al., Reference Schutte, De Jong, Smink and Koch1997). A period of 2 weeks may not be long enough to induce differences in growth rate. However, modifications of body composition, such as an alteration of the AA composition of the protein deposited, might have occurred before any alteration in weight gain (A. Hamard et al., unpublished data).
Plasma free threonine is responsive to dietary threonine supply (Le Floc’h et al., Reference Le Floc’h, Obled and Sève1995). As expected, plasma free threonine was 80% lower in LT than in C piglets. During the 1st week, plasma concentrations of AA were lower in LT compared with C piglets. This could be ascribed to an enhanced AA utilisation by the tissues of the LT piglets. Indeed, previous results showed that in weaned piglets, threonine deficiency increased fractional protein synthesis rate in the muscle and in the intestine (Sève and Ponter, Reference Sève and Ponter1997). Concerning non-essential AA, decrease in plasma glutamine and some of their end products (alanine, citrulline and ornithine) in LT piglets at day 4 could be in relation to the high energy cost of an increased protein turnover in the intestine since glutamine is used as a major energy fuel by the gut. In the 2nd week, threonine was the only essential AA for which plasma concentrations remained at a lower level in the LT compared with the C piglets, confirming that it was the most limiting dietary AA. Other differences in plasma free AA disappeared during the 2nd week suggesting that piglets progressively adapted their protein metabolism to a low threonine supply.
In the piglet, rapid growth of the intestine is critical to the expression of body growth potential and this implies high AA requirements to support gut protein synthesis. The high rate of threonine extraction by the intestine has been mainly associated with protein synthesis in the gut (Schaart et al., Reference Schaart, Schierbeek, Van der Schoor, Stoll, Burrin, Reeds and Van Goudoever2005; Le Floc’h et al., Reference Le Floc’h and Sève2005). In the present study, intestinal growth estimated from weight and length parameters as well as from mucosa/musculosa ratio was not altered by threonine supply. Moreover, the architecture of the proximal intestine was not altered in the piglets consuming the LT diet. This result supports the hypothesis that intestine growth was preserved in LT piglets as observed under other non-optimal nutritional conditions. Indeed, protein deposition seems to be only marginally decreased in the intestine compared with the carcass, for example, in situations of inadequate protein supply (Ebner et al., Reference Ebner, Schoknecht, Reeds and Burrin1994), tryptophan imbalance (Ponter et al., Reference Ponter, Cortamira, Seve, Salter and Morgan1994), fasting (McNurlan et al., Reference McNurlan, Tomkins and Garlick1979) and undernutrition associated with weaning (Sève et al., Reference Sève, Reeds, Fuller, Cadenhead and Hay1986). This could be associated with a stimulation of the intestinal fractional protein synthesis rate (Sève et al., Reference Sève, Reeds, Fuller, Cadenhead and Hay1986; Ponter et al., Reference Ponter, Cortamira, Seve, Salter and Morgan1994). In the same way, Sève and Ponter (Reference Sève and Ponter1997) reported that an inadequate dietary threonine supply in early-weaned piglets induced an increased fractional protein synthesis rate in the duodenum probably associated with an acceleration of protein turnover.
By contrast, decrease in dietary threonine supply induced villus atrophy in the ileum of the LT piglets, indicating a decrease in absorptive surface area. Moreover, crypt depth was reduced in the ileum of the LT piglets as were crypt width and villous height-to-crypt depth ratio. Villous atrophy is associated to gut disorders and malnutrition such as occurring at weaning, in parenterally fed animals or in animals suffering from infectious diseases. In these different situations, it is frequently associated with functional disturbances such as alteration of absorption capacity (Pluske et al., Reference Pluske, Williams and Aherne1995; Marion et al., Reference Marion, Romé, Savary, Thomas, Le Dividich and Le Huërou-Luron2003) and permeability (McCracken et al., Reference McCracken, Spurlock, Roos, Zuckermann and Gaskins1999; Kansagra et al., Reference Kansagra, Stoll, Rognerud, Niinikoski, Ou, Harvey and Burrin2003; Boudry et al., Reference Boudry, Péron, Le Huërou-Luron, Lallès and Sève2004). In the present study, villous atrophy was associated with a reduction in aminopeptidase N-specific activity in the ileum. Because brush-border enzyme activities sharply decline along the villus–crypt axis towards the crypt (Fan et al., Reference Fan, Stoll, Burrin and Jiang2001), the reduction in aminopeptidase N activity in the ileum was consistent with villi hypotrophy, both leading to altered digestive capacities in the ileum of the LT piglets. Despite this, we did not observe signs of intestinal clinical disease, diarrhoea and growth performance deterioration. This could be explained by the good sanitary conditions in which piglets were housed associated with the utilisation of a highly digestible diet. Nevertheless, such alterations might imply greater gut sensitivity, and thus may compromise the transition to a new diet or resistance to infection. Moreover, the response of the piglets to a longer period of treatment remains questionable.
An interesting observation is that villi atrophy induced by threonine deficiency was noticed only in the ileum. Law et al. (Reference Law, Bertolo, Adjiri-Awere, Pencharz and Ball2007) reported villi atrophy in the mid jejunum and the ileum of piglets receiving a diet strongly deficient in threonine (piglets received 17% of the requirements recommended by National Research Council (1998)) for 1 week. In our study, we may assume that enough dietary threonine was available for the proximal but not for the distal part of the intestine. Most luminal nutrients are absorbed in the proximal part of the intestine. This was confirmed by Schaart et al. (Reference Schaart, Schierbeek, Van der Schoor, Stoll, Burrin, Reeds and Van Goudoever2005) who showed that most of the dietary free threonine was taken up and incorporated into the proteins of the proximal part of the small intestine. By contrast, the distal part of the small intestine probably relies more on arterial AAs for protein synthesis. Stoll et al. (Reference Stoll, Chang, Fan, Reeds and Burrin2000) showed that in parenterally fed piglets the distal ileum maintained a positive protein balance whereas net protein loss occurred in the jejunum and in the proximal ileum. These data support the hypothesis that villi atrophy we observed in the ileum resulted from a decrease in systemic threonine availability. Villous atrophy was not observed in the proximal part of the small intestine at day 14, but we noted numerically higher villous height and villous/crypt ratio in the distal jejunum of the LT compared with the C piglets at day 14. So, we did not exclude that structural alterations might have occurred earlier. Indeed, Marion et al., (Reference Marion, Biernat, Thomas, Savary, Lebreton, Zabielski, Le Huërou-Luron and Le Dividich2002) showed that after weaning villous atrophy was first noticed in the proximal part of the small intestine. The restoration of the upper part of intestinal mucosa architecture was then noticed at the same time that villous atrophy concerns the distal part.
Very few data about the relationship between threonine intestinal metabolism and mucins synthesis are available. This can be related to the complexity in analysing mucin biology and characteristics. Total crude mucin content is reduced in the colon but not in the ileum of early-weaned piglets receiving 17% of threonine requirements for 1 week (Law et al., Reference Law, Bertolo, Adjiri-Awere, Pencharz and Ball2007). In adult rats fed 30% of threonine requirements for growth (Faure et al., Reference Faure, Moënnoz, Montignon, Mettraux, Breuille and Ballèvre2005), mucins synthesis is impaired in the ileum and the colon. Interestingly, Faure et al. (Reference Faure, Moënnoz, Montignon, Mettraux, Breuille and Ballèvre2005) noticed that rats receiving 60% of threonine requirements maintained mucins synthesis at a normal level. In the present study, threonine dietary supply did not significantly affect the total number of mucin-containing goblet cells in the villi of the ileum and in the crypts of the proximal large intestine. Although not significant, the numerical increase in mucin-containing goblet cells (18%) associated with a reduction of crypt surface would imply a higher mucin-containing goblet cells density in the ileal crypt of the LT piglets (Figure 2). As suggested by Conour et al. (Reference Conour, Ganessunker, Tappenden, Donovan and Gaskinsm2002), goblet cells expansion may represent a compensatory mechanism for the impairment of the barrier function by villus hypotrophy. However, this indicator is not fully representative of mucin synthesis capacity and did not take into account the different types of mucins. Indeed, Law et al. (Reference Law, Bertolo, Adjiri-Awere, Pencharz and Ball2007) noticed reduction in the number of ileal goblet cells producing acidic mucins, which are more resistant to bacterial mucolytic activities and protect against microbial penetration and translocation.
Conclusion
This study is the first one providing information on the impact of a 30% decrease in dietary threonine supply on intestine structure and growth of piglets. Our data indicate that a low dietary threonine supply did not alter intestine growth and architecture of the proximal small and large intestine. By contrast, villous hypotrophy observed in the ileum of piglets fed an LT diet for 2 weeks demonstrates that threonine supply is critical for maintaining the ileal structure. Additional experiments will be necessary to elucidate the impact of villi hypotrophy on gut functionality (permeability, sugar absorption, mucins biology) and its origin (protein metabolism).
Acknowledgements
We would like to thank Y. Lebreton, F. Legouevec and V. Piedvache for surgical procedure and animal care. We also thank Y. Colleaux for amino acid analysis, M. Formal for histochemistry and N. Mezière, P. Ganier, J.N. Nouchet and J.N. Thibault for their help during slaughter and tissue sampling.