Introduction
Since the discovery of antibiotics in the 1920s, they have played a substantial role in the advancement and prosperity of the poultry industry. Antibiotics have been supplemented in animal feed at sub-therapeutic doses to improve growth and feed conversion efficiency and to prevent infections for more than 60 years (Castanon, Reference Castanon2007). The effect of antibiotics on improving performance was first reported by Moore et al. (Reference Moore, Evenson, Luckey, McCoy, Elvehjem and Hart1946) when they observed that birds fed streptomycin exhibited increased growth responses. Many experiments conducted later in the early 1950s in chickens (Groschke and Evans, Reference Groschke and Evans1950; McGinnis, Reference McGinnis1950; Whitehill et al., Reference Whitehill, Oleson and Hutchings1950), pigs (Jukes et al., Reference Jukes, Stokstad, Taylor, Cunha, Edwards and Meadows1950; Luecke et al., Reference Luecke, McMillen and Thorp1950a, Reference Luecke, Newland, McMillen and Thorpb), and calves (Rusoff et al., Reference Rusoff, Davis and Alford1951) corroborated these results. In-feed antibiotic (IFA) use soon became a common and well-established practice in the animal industry and rose with the intensification of livestock production. In a review conducted by Rosen (Reference Rosen, Wallace and Chesson1995), it was concluded that inclusion of antibiotics in the diets gave a positive response 72% of the time. It was also proposed that the net effect of using IFA in the poultry industry was a 3–5% increase in growth and feed conversion efficiency (Choct, Reference Choct2001; Dahiya et al., Reference Dahiya, Wilkie, Van Kessel and Drew2006). Thus, it can be noted that IFA played a crucial role in contributing to the economic effectiveness of the livestock production (Wierup, Reference Wierup2000). Despite the well-demonstrated beneficial effects of IFA in improving the growth rate, reducing the mortality and increasing resistance to disease challenge, their use was also known to be associated with some disadvantages and challenges. Concerns exist that the use of IFA leads to development of antimicrobial resistance, posing a potential threat to human health (WHO, 2012). However, mixed opinions still exist on the transfer of antibiotic resistance genes from animal to human pathogens. Several studies showed that there might be a link between the practice of using sub-therapeutic antibiotics and the development of antimicrobial resistance among the microflora (Endtz et al., Reference Endtz, Mouton, van der Reyden, Ruijs, Biever and van Klingeren1990; Witte, Reference Witte1998; Wegener et al., Reference Wegener, Aarestrup, Jensen, Hammerum and Bager1999; Greko, Reference Greko, Piva, Bach Knudsen and Lindberg2001; M'ikanatha et al., Reference M'ikanatha, Sandt, Localio, Tewari, Rankin, Whichard, Altekruse, Lautenbach, Folster, Russo, Chiller, Reynolds and McDermott2010; Medeiros et al., Reference Medeiros, Oliveira, Rodrigues Ddos and Freitas2011; Cosby et al., Reference Cosby, Cox, Harrison, Wilson, Buhr and Fedorka-Cray2015).
Despite these debates on the role of IFA use in conferring antimicrobial resistance to human pathogens, the European Union issued a ban on the approval for antibiotics as growth promoters since 1 January 2006 on precautionary grounds (Dibner and Richards, Reference Dibner and Richards2005; Castanon, Reference Castanon2007). In the USA, antibiotic use in livestock and poultry feeds is under great scrutiny as a result of increasing consumer awareness and the demand for livestock products from antibiotic-free production systems. In 2013, the US Food and Drug Administration (FDA) called for major manufacturers of medically important animal drugs to voluntarily stop labeling them for growth promotion in animals and revise the labels such that veterinary supervision is required for therapeutic uses (GFI#213; FDA, 2013). FDA continued to strengthen its agenda on promoting judicious use of antimicrobials in food-producing animals and published its final rule of the VFD (Veterinary Feed Directive) in early 2015, bringing the use of medically important antimicrobials in feed under veterinary supervision, so that they are used only when necessary to ensure the health of the animals. In late 2015, the state of California passed a bill (Senate Bill 27) enforcing a strict ban on using medically important antimicrobials in animal feeds for both growth promotion and disease prevention.
The decline in the use of antibiotic growth promoters (AGPs) in the future seems inevitable, and the practice of using antimicrobials may prove economically impractical because of market limitations and export restrictions (Dibner and Richards, Reference Dibner and Richards2005). In view of the increasing concerns over AGP use, the quest for novel alternate replacements to mitigate antibiotic use in animal agriculture has grown over the years. In the past two decades, a great deal of research has focused on the development of antibiotic alternatives to maintain or improve poultry health and performance. This review, therefore, is focused on current knowledge pertaining to several of the strategies that are being employed to improve poultry growth performance and provides a brief overview of such alternatives along with a description of their efficacy and modes of action.
Mechanism of action of AGPs
The successful development of antibiotic alternatives, at least to some extent, relies on understanding the mechanism of action of AGPs. Several ideas have been proposed to elucidate the rationale behind antibiotic-mediated growth enhancement, but to date there is no clear-cut explanation. Preliminary theories have linked their efficacy to their antibacterial action, which was thought to be mediated by a reduction in the overall numbers or diversity of the gut microbiota (Francois, Reference Francois1961; Visek, Reference Visek1978), resulting in decreased competition for nutrients and reduced microbial metabolites that affect growth (amino acid and bile catabolism) (Feighner and Dashkevicz, Reference Feighner and Dashkevicz1987; Gaskins et al., Reference Gaskins, Collier and Anderson2002; Knarreborg et al., Reference Knarreborg, Lauridsen, Engberg and Jensen2004). This theory was contradicted by Niewold (Reference Niewold2007), who proposed that the beneficial effects of antibiotics are due to their interaction with host immune cells rather than the growth inhibitory effects on microbiota. He hypothesized that antibiotics lower the inflammatory response and thus the production of proinflammatory cytokines, which reduce the appetite and promote muscle catabolism. The anti-inflammatory role of AGP reduces wasted energy and directs it toward production (Niewold, Reference Niewold2007).
Though a clear consensus on how AGP acts still does not exist in the scientific community, it is now clear – with the advent of novel molecular biology and bioinformatics techniques – that shifts in microbiota composition (structure and diversity) do occur when antibiotics are included in animal diets (Dumonceaux et al., Reference Dumonceaux, Hill, Hemmingsen and Van Kessel2006; Pedroso et al., Reference Pedroso, Menten, Lambais, Racanicci, Longo and Sorbara2006; Wise and Siragusa, Reference Wise and Siragusa2007; Lin et al., Reference Lin, Hunkapiller, Layton, Chang and Robbins2013). These shifts may ultimately result in an optimal and balanced microbiota that is less capable of evoking an inflammatory response, increases energy harvest from nutrients, and helps animals perform to their genetic potential (Huyghebaert et al., Reference Huyghebaert, Ducatelle and Van Immerseel2011; Lin, Reference Lin2011). However, it still remains challenging to definitively link-specific bacterial populations to enhanced growth and pinpoint ways/tools to modify microbiota to a desired one (Lin, Reference Lin2014). A few research trials were conducted to associate bacterial products or enzymes to enhanced performance, and have shown a decrease in bile salt hydrolase (BSH) enzyme activity in the gut. It was proposed that BSH produced by gut bacteria catalyzes deconjugation of bile acids and alters host lipid metabolism, and AGPs acts by reducing the number of bacteria that are producing BSH (Feighner and Dashkevicz, Reference Feighner and Dashkevicz1987; Knarreborg et al., Reference Knarreborg, Lauridsen, Engberg and Jensen2004; Guban et al., Reference Guban, Korver, Allison and Tannock2006; Lin, Reference Lin2014). Recent studies conducted in mice revealed that exposure to sub-therapeutic antibiotic levels not only altered the composition of gut microbiota, but also their metabolic capability by selecting for microbial species that were capable of extracting a high proportion of calories from complex carbohydrates (increase in copies of genes involved in metabolism of carbohydrates to short-chain fatty acids (SCFA)) (Cho et al., Reference Cho, Yamanishi, Cox, Methé, Zavadil, Li, Gao, Mahana, Raju, Teitler, Li, Alekseyenko and Blaser2012). The growth-promotion phenotype was shown to be transferrable to germ-free hosts by low-dose antibiotic-selected microbiota, indicating that the altered microbiota and not the antibiotics played a causal role (Cho et al., Reference Cho, Yamanishi, Cox, Methé, Zavadil, Li, Gao, Mahana, Raju, Teitler, Li, Alekseyenko and Blaser2012). It was also shown from the studies in mice that exposure to low-dose antibiotics early in life induces long-term host metabolic effects by accelerating normal age-related microbiota development and altering ileal expression of the genes involved in immunity (Cox et al., Reference Cox, Yamanishi, Sohn, Alekseyenko, Leung, Cho, Kim, Li, Gao, Mahana, Rodriguez, Rogers, Robine, Loke and Blaser2014). Though the effects observed in mice cannot be directly extrapolated to farm animals, they might provide an insight into a possible mechanism of action.
Classes of alternatives
An ideal alternative should have the same beneficial effects of AGP, ensure optimum animal performance, and increase nutrient availability (Huyghebaert et al., Reference Huyghebaert, Ducatelle and Van Immerseel2011). Considering the proposed mechanism of action of AGPs (microbiome and immune-modulating activities), a practical alternative should possess both of these properties in addition to having a positive impact on feed conversion and/or growth (Huyghebaert et al., Reference Huyghebaert, Ducatelle and Van Immerseel2011; Seal et al., Reference Seal, Lillehoj, Donovan and Gay2013). Several classes of alternatives have been proposed and tested in poultry production, including probiotics, prebiotics, synbiotics, organic acids, enzymes, phytogenics and metals. Novel alternatives such as hyperimmune egg yolk IgY, antimicrobial peptides (AMP), bacteriophages, and clay have come into existence in recent years.
Probiotics
Probiotics, sometimes used interchangeably with the term direct fed microbials (DFMs), are gaining acceptance as potential alternatives to antibiotics to improve production efficiency (Lee et al., Reference Lee, Lillehoj and Siragusa2010c). They are defined as “live microbial feed supplements which beneficially affect the host animal by improving its intestinal microbial balance” (Fuller, Reference Fuller1989). A recent definition adopted by FAO/WHO (2001) states that “Probiotics are mono or mixed cultures of live organisms which when administered in adequate amounts confer a health benefit to the host.” Probiotics may contain one or more strains of microorganisms and may be given either alone or in combination with other additives in feed or water (Thomke and Elwinger, Reference Thomke and Elwinger1998). Novel application strategies such as spraying on chicks or embryonated eggs are also practiced and potential methods such as in-ovo application are being explored (Wolfenden et al., Reference Wolfenden, Pixley, Higgins, Higgins, Vicente, Torres-Rodriguez, Hargis and Tellez2007; Cox and Dalloul, Reference Cox and Dalloul2015).
A variety of bacteria (Bacillus, Bifidobacterium, Enterococcus, Lactobacillus, Streptococcus, and Lactococcus spp.) and in some cases yeast (Saccharomyces spp.) have been tested as probiotics in poultry (Simon et al., Reference Simon, Jadamus and Vahjen2001; Patterson and Burkholder, Reference Patterson and Burkholder2003; Griggs and Jacob, Reference Griggs and Jacob2005; Kabir, Reference Kabir2009). The majority of the conducted research was specifically aimed at investigating the effects of probiotics in reducing the numbers of pathogenic microorganisms in the gastrointestinal tract. However, a considerable amount of research also examined the effects of probiotics on improving growth and performance in poultry without apparent disease. Supplementation of diets with a single strain of Lactobacillus sp. (L. casei, L. fermentum, L. bulgaricus, L. reuteri) was shown to improve the body weight and feed efficiency in broilers (Yeo and Kim, Reference Yeo and Kim1997; Khan et al., Reference Khan, Raoult, Richet, Lepidi and La Scola2007; Apata, Reference Apata2008; Nakphaichit et al., Reference Nakphaichit, Thanomwongwattana, Phraephaisarn, Sakamoto, Keawsompong, Nakayama and Nitisinprasert2011; Salim et al., Reference Salim, Kang, Akter, Kim, Kim, Kim, Na, Jong, Choi, Suh and Kim2013). Similar results were shown when broilers were given multiple strains of Lactobacillus sp. (Jin et al., Reference Jin, Ho, Abdullah and Jalaludiin1998; Kalavathy et al., Reference Kalavathy, Abdullah, Jalaludin and Ho2003; Mookiah et al., Reference Mookiah, Sieo, Ramasamy, Abdullah and Ho2014). Bacillus sp.-based probiotics (B. coagulans, B. subtilis, B. licheniformis, and B. amyloliquefaciens) were also successfully employed in poultry diets and were shown to have growth-promoting effects (Cavazzoni et al., Reference Cavazzoni, Adami and Castrovilli1998; Lee et al., Reference Lee, Lee, Lillehoj, Li, Jang, Babu, Park, Kim, Lillehoj, Neumann, Rehberger and Siragusa2010a, Reference Lee, Li, Lillehoj, Lee, Jang, Babu, Lillehoj, Neumann and Siragusa2011a; Wang and Gu, Reference Wang and Gu2010; Liu et al., Reference Liu, Yan, Lv, Xu, Yin, Zhang, Wang and Hu2012; Sen et al., Reference Sen, Ingale, Kim, Kim, Kim, Lohakare, Kim, Kim, Ryu, Kwon and Chae2012; Ahmed et al., Reference Ahmed, Islam, Mun, Sim, Kim and Yang2014; Jeong and Kim, Reference Jeong and Kim2014; Park and Kim, Reference Park and Kim2014). The application of several other probiotic bacteria such as Enterococcus faecium (Samli et al., Reference Samli, Senkoylu, Koc, Kanter and Agma2007; Kabir et al., Reference Kabir, Rahman, Rahman, Rahman and Ahmed2004), Clostridium butyricum (Yang et al., Reference Yang, Cao, Ferket, Liu, Zhou, Zhang, Xiao and Chen2012; Zhao et al., Reference Zhao, Guo, Guo and Tan2013a; Liao et al., Reference Liao, Ma, Cai, Fu, Yan, Wei and Zhang2015), Rhodopseudomonas palustris (Xu et al., Reference Xu, Yan, Liu, Lv, Yin and Wang2014) also significantly increased the daily weight gains with decreased feed conversion ratio (FCR). Research trials have also been conducted with multi-microbe probiotic mixtures composed of combinations of different beneficial bacteria and/or yeast and were shown to exhibit a growth-promoting effect (Chiang and Hsieh, Reference Chiang and Hsieh1995; Huang et al., Reference Huang, Choi, Houde, Lee, Lee and Zhao2004; Mountzouris et al., Reference Mountzouris, Tsirtsikos, Kalamara, Nitsch, Schatzmayr and Fegeros2007, Reference Mountzouris, Tsirtsikos, Palamidi, Arvaniti, Mohnl, Schatzmayr and Fegeros2010; Nayebpor et al., Reference Nayebpor, Farhomand and Hashemi2007; Talebi et al., Reference Talebi, Amirzadeh, Mokhtari and Gahri2008; Torshizi et al., Reference Torshizi, Moghaddam, Rahimi and Mojgani2010; Kim et al., Reference Kim, Ingale, Kim, Kim, Sen, Ryu, Lohakare, Kwon and Chae2012; Bai et al., Reference Bai, Wu, Ding, Lei, Bai, Zhang and Chio2013; Alimohamadi et al., Reference Alimohamadi, Taherpour, Ghasemi and Fatahnia2014; Zhang and Kim, Reference Zhang and Kim2014). Faria Filho et al. (Reference Faria Filho, Torres, Faria, Campos and Rosa2006) performed a meta-analysis of 27 studies involving 30,146 broiler chickens that were conducted in Brazil during 1995–2005 to investigate the performance effects of 12 different probiotics. The results of their analysis showed that overall the probiotic supplementation improved the body weight gain by 0.14 and reduced FCR by 0.10 points compared with non-supplemented controls. A similar meta-analysis of several randomized controlled research trials that were carried out from 1980 to 2012 was conducted by Blajman et al. (Reference Blajman, Frizzo, Zbrun, Astesana, Fusari, Soto, Rosmini and Signorini2014) to investigate the effects of probiotics on body weight gain and feed efficiency in broilers. They concluded that probiotics inclusion increased body weight gain and improved feed efficiency, and also showed that probiotics application via water was more efficacious than through feed. The analysis also showed that there were no differences between the use of mono- or multi-strain probiotics and the effects observed may vary with the type of strain used.
In addition to the improved growth performance, probiotics supplementation was also shown to enhance the general immune function of broilers, as evidenced by the augmented serum/plasma immunoglobulin levels, increased antibody titers to pathogens, and changes in immune cell numbers (Nayebpor et al., Reference Nayebpor, Farhomand and Hashemi2007; Apata, Reference Apata2008; Lee et al., Reference Lee, Li, Lillehoj, Lee, Jang, Babu, Lillehoj, Neumann and Siragusa2011a; Bai et al., Reference Bai, Wu, Ding, Lei, Bai, Zhang and Chio2013; Salim et al., Reference Salim, Kang, Akter, Kim, Kim, Kim, Na, Jong, Choi, Suh and Kim2013; Ahmed et al., Reference Ahmed, Islam, Mun, Sim, Kim and Yang2014). The intestines of broilers that were given probiotics showed better development and an increase in villus height and crypt depth compared with controls (Samli et al., Reference Samli, Senkoylu, Koc, Kanter and Agma2007; Lee et al., Reference Lee, Lee, Lillehoj, Li, Jang, Babu, Park, Kim, Lillehoj, Neumann, Rehberger and Siragusa2010a, Reference Lee, Li, Lillehoj, Lee, Jang, Babu, Lillehoj, Neumann and Siragusa2011a; Kim et al., Reference Kim, Ingale, Kim, Kim, Sen, Ryu, Lohakare, Kwon and Chae2012; Sen et al., Reference Sen, Ingale, Kim, Kim, Kim, Lohakare, Kim, Kim, Ryu, Kwon and Chae2012). Probiotics supplementation also positively modulated the intestinal microbiota and increased numbers of beneficial bacteria such as Lactobacillus and Bifidobacterium spp. (Mountzouris et al., Reference Mountzouris, Tsirtsikos, Kalamara, Nitsch, Schatzmayr and Fegeros2007, Reference Mountzouris, Tsirtsikos, Palamidi, Arvaniti, Mohnl, Schatzmayr and Fegeros2010; Samli et al., Reference Samli, Senkoylu, Koc, Kanter and Agma2007; Nakphaichit et al., Reference Nakphaichit, Thanomwongwattana, Phraephaisarn, Sakamoto, Keawsompong, Nakayama and Nitisinprasert2011; Yang et al., Reference Yang, Cao, Ferket, Liu, Zhou, Zhang, Xiao and Chen2012; Jeong and Kim, Reference Jeong and Kim2014; Mookiah et al., Reference Mookiah, Sieo, Ramasamy, Abdullah and Ho2014; Zhang and Kim, Reference Zhang and Kim2014).
The beneficial effects of probiotics supplementation were also reported in laying hens. Kurtoglu et al. (Reference Kurtoglu, Kurtoglu, Seker, Coskun, Balevi and Polat2004) showed that hens fed diets supplemented with probiotics showed increased egg production compared with controls. Lei et al. (Reference Lei, Li, Yu, Rajput and Li2013) reported that dietary inclusion of B. licheniformis improved laying performance and egg mass. Consistent with these findings, various DFM product supplementation was also shown to improve body weight and performance in turkeys (Russell and Grimes, Reference Russell and Grimes2009; Wolfenden et al., Reference Wolfenden, Pumford, Morgan, Shivaramaiah, Wolfenden, Pixley, Green, Tellez and Hargis2011). Lactobacillus-based probiotics significantly improved market body weight and average daily gain of commercial turkeys (Torres-Rodriguez et al., Reference Torres-Rodriguez, Donoghue, Donoghue, Barton, Tellez and Hargis2007). Albeit numerous publications show the performance improvement in broilers, layers, and turkeys, reports also exist that probiotics show limited and variable growth-promoting effect and in some instances none (Karaoglu and Durdag, Reference Karaoglu and Durdag2005; O'Dea et al., Reference O'Dea, Fasenko, Allison, Korver, Tannock and Guan2006; Lee et al., Reference Lee, Lee, Lillehoj, Li, Jang, Babu, Park, Kim, Lillehoj, Neumann, Rehberger and Siragusa2010a; Waititu et al., Reference Waititu, Yitbarek, Matini, Echeverry, Kiarie, Rodriguez-Lecompte and Nyachoti2014). This inconsistency in the results can be attributed to the differences in the type and dose of strain used, processing variations, administration time and period, diet, and environment.
Although the modes of action by which probiotics improve performance and promote gut health are not completely understood, a few have been proposed and reviewed (Edens, Reference Edens2003; Parvez et al., Reference Parvez, Malik, Ah Kang and Kim2006; Kabir, Reference Kabir2009; Ng et al., Reference Ng, Hart, Kamm, Stagg and Knight2009; Vilà et al., Reference Vilà, Esteve-Garcia and Brufau2010; Lee et al., Reference Lee, Lee, Lillehoj, Li, Jang, Babu, Park, Kim, Lillehoj, Neumann, Rehberger and Siragusa2010a). The two most important mechanisms through which probiotics exert beneficial effects include balancing the gut microflora and immune regulation. Probiotics help establish a microenvironment in the gut that favors beneficial microorganisms and reduces the colonization of pathogenic bacteria (competitive exclusion) by: (1) creating a hostile environment for harmful bacterial species (through production of lactic acid, SCFA, and reduction in pH); (2) competing for nutrients with undesired bacteria; (3) production and secretion of antibacterial substances (e.g. bacteriocins by Lactobacillus, Bacillus spp.); and (4) inhibition of bacterial adherence and translocation (Nurmi and Rantala, Reference Nurmi and Rantala1973; Fuller, Reference Fuller1989; Netherwood et al., Reference Netherwood, Gilbert, Parker and O'Donnell1999; Schneitz, Reference Schneitz2005; Ng et al., Reference Ng, Hart, Kamm, Stagg and Knight2009; Brown, Reference Brown2011). Probiotics are also known to improve intestinal function by maintaining epithelial cell homoeostasis, promoting cytoprotective responses and cell survival (through production of cytokines that enhance epithelial cell regeneration and inhibit apoptosis), improving barrier function (modulation of cytoskeletal and epithelial tight junctions), and increasing mucin synthesis (Chichlowski et al., Reference Chichlowski, Croom, McBride, Havenstein and Koci2007; Ng et al., Reference Ng, Hart, Kamm, Stagg and Knight2009; Brown, Reference Brown2011). They also play an important role in digestion and nutrient retention by increasing digestive enzyme activity and improving the breakdown of indigestible nutrients (Jin et al., Reference Jin, Ho, Abdullah and Jalaludin2000; Ciorba, Reference Ciorba2012; Ng et al., Reference Ng, Hart, Kamm, Stagg and Knight2009; Wang and Gu, Reference Wang and Gu2010). Probiotics also exert their action by reducing toxic amine production and ammonia levels in the gut (Chiang and Hsieh, Reference Chiang and Hsieh1995). Another important mechanism of probiotics action includes modulating and regulating intestinal immune responses by reducing pro-inflammatory cytokines, increasing secretory IgA production, and promoting specific and non-specific immune responses against pathogens (activation of macrophages, increase cytokine production by intraepithelial lymphocytes) (Ng et al., Reference Ng, Hart, Kamm, Stagg and Knight2009; Lee et al., Reference Lee, Lee, Lillehoj, Li, Jang, Babu, Park, Kim, Lillehoj, Neumann, Rehberger and Siragusa2010a, Reference Lee, Li, Lillehoj, Lee, Jang, Babu, Lillehoj, Neumann and Siragusa2011a).
Thus, an ideal probiotic organism should be able to withstand processing and storage, survive in the gastric acidic environment, adhere to epithelium or mucus in the intestines, produce antimicrobial compounds, and modulate immune responses (Edens, Reference Edens2003; Patterson and Burkholder, Reference Patterson and Burkholder2003; Cheng et al., Reference Cheng, Hao, Xie, Wang, dai, Huang and Yuan2014). However, not all strains exhibit all of the above properties and care must be taken to select the strains or their combinations that will achieve maximum beneficial effect in vivo. Measures to protect the organisms during their passage through the upper alimentary tract such as a microencapsulation should be considered to ensure viability and colonization in the intestine (Han et al., Reference Han, Zhang, Wang, Li, Liu and Zhao2013). Overall, it can be said that probiotics can serve as potential alternatives to antibiotics for increasing poultry performance.
Prebiotics
Prebiotics are defined as ‘non-digestible feed ingredients that beneficially affect the host by selectively stimulating the growth and/or activity of one or a limited number of bacteria in the gut’ (Gibson and Roberfroid, Reference Gibson and Roberfroid1995; Patterson and Burkholder, Reference Patterson and Burkholder2003). A recent definition (FAO, 2007) describes prebiotics as ‘non-viable feed components that confer a health benefit on the host associated with modulation of the microbiota.’ A variety of non-starch polysaccharides (NSP) or oligosaccharides have been considered as prebiotics, including mannan oligosaccharide (MOS), fructooligosaccharide (FOS), inulin, oligofructose, galactooligosaccharide, maltooligosaccharide, lactulose, lactitol, glucooligosaccharide, xylooligosaccharide, soya-oligosaccharide, isomaltooligosaccharide (IOS), and pyrodextrins (Patterson and Burkholder, Reference Patterson and Burkholder2003; Steiner, Reference Steiner2006).
Prebiotics are macromolecules that are either derived from plants or synthesized by microorganisms. MOS, derived from the outer cell-wall layer of Saccharomyces cerevisiae, has been studied extensively as a prebiotic supplement in poultry diets. The addition of various levels of MOS to the broiler diets significantly increased their body weight and improved feed conversion efficiency (Benites et al., Reference Benites, Gilharry, Gernat and Murillo2008; Bozkurt et al., Reference Bozkurt, Küçükyilmaz, Çatli and Çinar2008; Hooge et al., Reference Hooge, Sims, Sefton, Connolly and Spring2003; Yang et al., Reference Yang, Iji and Choct2007; Mohamed et al., Reference Mohamed, Hassan and El-Barkouky2008) with increased intestinal villi height (Baurhoo et al., Reference Baurhoo, Phillip and Ruiz-Feria2007; Yang et al., Reference Yang, Iji and Choct2007), improved immune-competence in the intestine (Janardhana et al., Reference Janardhana, Broadway, Bruce, Lowenthal, Geier, Hughes and Bean2009; Shanmugasundaram and Selvaraj, Reference Shanmugasundaram and Selvaraj2012), altered jejunal gene expression (Xiao et al., Reference Xiao, Power, Mallonee, Routt, Spangler, Pescatore, Cantor, Ao, Pierce and Dawson2012; Brennan et al., Reference Brennan, Graugnard, Xiao, Spry, Pierce, Lumpkins and Mathis2013), and influenced intestinal microbiota (Geier et al., Reference Geier, Torok, Allison, Ophel-Keller and Hughes2009; Corrigan et al., Reference Corrigan, Horgan, Clipson and Murphy2011; Kim et al., Reference Kim, Seo, Kim and Paik2011; Pourabedin et al., Reference Pourabedin, Xu, Baurhoo, Chevaux and Zhao2014). FOS, which is derived from plants, has also been shown to possess significant prebiotic effect and improve performance in broiler chickens (Xu et al., Reference Xu, Hu, Xia, Zhan and Wang2003; Kim et al., Reference Kim, Seo, Kim and Paik2011). Another class of prebiotics includes IOS showing promise as an antibiotic alternative owing to their efficacy in improving weight gain and FCR when fed to broilers (Mookiah et al., Reference Mookiah, Sieo, Ramasamy, Abdullah and Ho2014).
Lactulose is a non-digestible, synthetic disaccharide that was also proven to show prebiotic effect in humans and pigs. Calik and Ergün (Reference Calik and Ergün2015) showed that lactulose supplementation in broiler diets not only improved body weight and FCR, but also increased villi height, goblet cell numbers, total SCFA concentrations, and Lactobacillus counts. Similar results of improvement in FCR and Lactobacillus counts with lactulose supplementation were shown by Cho and Kim (Reference Cho and Kim2014). Various other prebiotics that were tested and found to be beneficial in poultry include lignin (Baurhoo et al., Reference Baurhoo, Phillip and Ruiz-Feria2007), inulin (Alzueta et al., Reference Alzueta, Rodríguez, Ortiz, Rebolé and Treviño2010; Rebolé et al., Reference Rebolé, Ortiz, Rodríguez, Alzeuta, Treviño and Velasco2010), and palm kernel extract (Rezaei et al., Reference Rezaei, Jahromi, Liang, Zulkifli, Farjam, Laudadio and Tufarelli2015). In contrast to the previous results, several authors reported that prebiotic supplementation had no effect on performance (Baurhoo et al., Reference Baurhoo, Phillip and Ruiz-Feria2007; Józefiak et al., Reference Józefiak, Kaczmarek and Rutkowski2008; Geier et al., Reference Geier, Torok, Allison, Ophel-Keller and Hughes2009; Corrigan et al., Reference Corrigan, Horgan, Clipson and Murphy2011; Houshmand et al., Reference Houshmand, Azhar, Zulkifli, Bejo and Kamyab2012). However, statistical analysis of numerous trials conducted with prebiotic supplementation in the diets of broiler chickens was shown to beneficially influence their growth and performance. Holo- and meta-analysis of several research trials conducted over the years using prebiotics in feed have confirmed these effects (Hooge, Reference Hooge2004; Rosen, Reference Rosen2007; Hooge and Connolly, Reference Hooge and Connolly2011). It was shown that adding a yeast cell-wall product to the diets significantly improved body weight by 1.61% and reduced FCR by 1.99%, respectively (Hooge, Reference Hooge2004). Hooge and Connolly (Reference Hooge and Connolly2011) reported that prebiotics improved body weight by 5.41%, decreased FCR by 2.54%, and reduced mortality by 10.5%.
A number of characteristics should be taken into consideration when selecting prebiotics, including resistance to gastric acidic environment, intestinal/pancreatic enzyme hydrolysis, and absorption across intestinal epithelium (Hume, Reference Hume2011; Heo et al., Reference Heo, Opapeju, Pluske, Kim, Hampson and Nyachoti2013; Ricke, Reference Ricke2015). The most important characteristic of an ideal prebiotic is the ability to selectively enrich beneficial microorganisms associated with health and well-being (Simmering and Blaut, Reference Simmering and Blaut2001; Patterson and Burkholder, Reference Patterson and Burkholder2003; Heo et al., Reference Heo, Opapeju, Pluske, Kim, Hampson and Nyachoti2013; Samantha et al., Reference Samantha, Jayapal, Senani, Kolte and Sridhar2013). Thus, the majority of the beneficial effects of prebiotics are thought to be mediated predominantly through altering the intestinal microbiota (Pourabedin and Zhao, Reference Pourabedin and Zhao2015). Prebiotics also prevent pathogen colonization either by binding directly or by competitive exclusion by promoting the growth of beneficial microbes or by stimulating them to produce bacteriocins and lactic acid (Spring et al., Reference Spring, Wenk, Dawson and Newman2000). In particular, MOS acts by binding to type 1 fimbriae of enteric pathogens and prevents their adhesion to intestinal epithelial cells (Spring et al., Reference Spring, Wenk, Dawson and Newman2000). The fermentation of prebiotics by microflora also leads to the production of SCFA that act as energy sources for intestinal epithelial cells and thus maintain the integrity of the gut lining (Ferket et al., Reference Ferket, Santos and Oviedo-Rondon2005). Prebiotics also act by beneficially altering luminal or systemic aspects of the host immune system. MOS is recognized by receptors of the innate immune system, act as adjuvants, and help boost the host immune responses (Ferket et al., Reference Ferket, Santos and Oviedo-Rondon2005).
Synbiotics
Synbiotics are additives that combine the use of probiotics and prebiotics such that they act synergistically (Alloui et al., Reference Alloui, Szczurek and Świątkiewicz2013). The use of synbiotics was based on the concept that a mixture of probiotics and prebiotics beneficially affect the host by improving the survival and implantation of probiotic organisms and by selectively promoting the growth or metabolism of beneficial bacteria in the intestinal tract (Gibson and Roberfroid, Reference Gibson and Roberfroid1995). Few research trials have been conducted to demonstrate the effects of synbiotics on broiler performance. Supplementation of diets with a synbiotic product was shown to significantly improve body weight, average daily gain, feed efficiency, and carcass yield percentage compared with controls or probiotic-fed broilers (Awad et al., Reference Awad, Ghareeb, Abdel-Raheem and Böhm2009). Ashayerizadeh et al. (Reference Ashayerizadeh, Dabiri, Ashayerizadeh, Mirzadeh, Roshanfekr and Mamooee2009) reported similar improvement in growth indices and Mohnl et al. (Reference Mohnl, Acosta Aragón, Acosta Ojeda, Rodríguez Sánchez and Pasteiner2007) showed that synbiotics increased body weight by 2.04% and reduced mortality by 0.9% compared with controls. Mookiah et al. (Reference Mookiah, Sieo, Ramasamy, Abdullah and Ho2014) reported a significant increase in weight gain and a decrease in the FCR when birds were fed diets with a combination of IOS and probiotic mixture (11 strains of Lactobacillus spp.). However, the synbiotic did not show a 2-fold synergistic effect compared with those of prebiotics or probiotics alone. A combination of yeast-derived carbohydrates and probiotics was shown to increase body weight gain compared with controls or prebiotic-supplemented pullets (Yitbarek et al., Reference Yitbarek, Echeverry, Munyaka and Rodriguez-Lecompte2015). In contrast, some of the trials conducted with in-feed inclusion of synbiotics did not show that performance was affected (Willis et al., Reference Willis, Isikhuemhen and Ibrahim2007; Jung et al., Reference Jung, Houde, Baurhoo, Zhao and Lee2008). Synbiotics were also shown to beneficially alter the intestinal microbiota composition and increase villi height and crypt depth in the intestinal mucosa (Jung et al., Reference Jung, Houde, Baurhoo, Zhao and Lee2008; Awad et al., Reference Awad, Ghareeb, Abdel-Raheem and Böhm2009; Sohail et al., Reference Sohail, Hume, Byrd, Nisbet, Ijaz, Sohail, Shabbir and Rehman2012). There is a great potential for synbiotics to be used as antibiotic alternatives for improving performance and reducing pathogenic load in the intestines of poultry. Careful consideration must be given when selecting the combinations of various prebiotics and probiotics to be used as synbiotics, and research trials should be conducted to demonstrate their synergistic effect compared with the use of either product alone (Fig. 1).
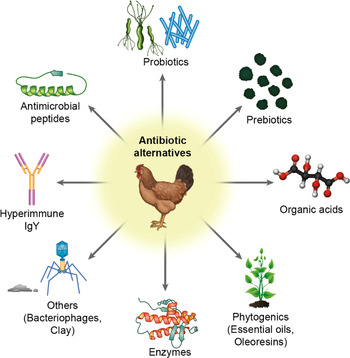
Fig. 1. Various classes of antibiotic alternatives that are available for use in poultry production.
Organic acids
Dietary organic acids have been considered as potential alternatives to AGPs, owing to their antibacterial nature. Chemically, organic acids used in food animal production can be described as either simple monocarboxylic acids (e.g., formic, acetic, propionic, and butyric acids) or carboxylic acids bearing hydroxyl group (e.g., lactic, malic, tartaric, and citric acids) (Dibner and Buttin, Reference Dibner and Buttin2002). They are widely distributed in nature as normal constituents of animal or plant tissues and some of them (specifically SCFA) are produced in the hind gut of food animals and humans through microbial fermentation of carbohydrates (Van Der Wielen et al., Reference Van Der Wielen, Biesterveld, Notermans, Hofstra, Urlings and Van Knapen2000; Ricke, Reference Ricke2003; Huyghebaert et al., Reference Huyghebaert, Ducatelle and Van Immerseel2011). They can be administered in the feed or drinking water and can be used either individually as organic acids or their salts (sodium, potassium, or calcium) or as blends of multiple acids or their salts (Huyghebaert et al., Reference Huyghebaert, Ducatelle and Van Immerseel2011).
Organic acid use has been shown to have significant benefits in swine and poultry production over the years. Dietary supplementation of fumaric acid in broiler chickens was shown to improve weight gain and feed efficiency (Patten and Waldroup, Reference Patten and Waldroup1988; Skinner et al., Reference Skinner, Izat and Waldroup1991; Biggs and Parsons, Reference Biggs and Parsons2008; Adil et al., Reference Adil, Banday, Bhat, Mir and Rehman2010, Reference Adil, Banday, Bhat, Salahuddin, Raquib and Shanaz2011; Banday et al., Reference Banday, Adil, Khan and Untoo2015). Similar effects of growth performance improvement were seen when butyric acid was included in the broiler feed (Panda et al., Reference Panda, Rama Rao, Raju and Shyam Sunder2009; Adil et al., Reference Adil, Banday, Bhat, Mir and Rehman2010, Reference Adil, Banday, Bhat, Salahuddin, Raquib and Shanaz2011). Several other organic acids that were tested and shown to improve performance in poultry include lactic (Adil et al., Reference Adil, Banday, Bhat, Mir and Rehman2010, Reference Adil, Banday, Bhat, Salahuddin, Raquib and Shanaz2011), citric (Chowdhury et al., Reference Chowdhury, Islam, Khan, Karim, Haque, Khatun and Pesti2009; Haque et al., Reference Haque, Islam, Akbar, Chowdhury, Khatun, Karim and Kemppainen2010; Salgado-Tránsito et al., Reference Salgado-Tránsito, Del Río-García, Arjona-Román, Moreno-Martínez and Méndez-Albores2011), formic (Patten and Waldroup, Reference Patten and Waldroup1988; Hernández et al., Reference Hernández, Garcia, Madrid, Orengo, Catalá and Megias2006; Panda et al., Reference Panda, Rama Rao, Raju and Shyam Sunder2009), malic, sorbic, and tartaric acids. Research has shown that the beneficial effects of organic acids can be enhanced by using them as blends rather than a single acid. Various organic acid blends were tested and shown to improve the FCR in broiler chickens (Samanta et al., Reference Samanta, Haldar and Ghosh2008, Reference Samanta, Haldar and Ghosh2010).
Though the mechanism of action of organic acids is not clearly understood, it can be attributed to their antibacterial activity. Several possible mechanisms include the following: (1) reducing the pH level of the upper gastrointestinal tract (crop, proventriculus, gizzard) and associated physiological changes in the gut mucosa (Samanta et al., Reference Samanta, Haldar and Ghosh2008; Panda et al., Reference Panda, Rama Rao, Raju and Shyam Sunder2009); (2) altering the gut microflora either by directly killing through cell-wall penetration or by indirectly modifying pH and reducing the numbers of pathogenic bacteria, increasing acid-tolerant beneficial species such as Lactobacilllus spp. and reducing competition for nutrients by the altered microbes (Biggs and Parsons, Reference Biggs and Parsons2008; Nava et al., Reference Nava, Attene-Ramos, Gaskins and Richards2009; Czerwiński et al., Reference Czerwiński, Højberg, Smulikowska, Engberg and Mieczkowska2010; Boroojeni et al., Reference Boroojeni, Vahjen, Mader, Knorr, Ruhnke, Röhe, Hafeez, Villodre, Männer and Zentek2014); (3) increasing nutrient digestibility by elevating protein and dry matter retention, improving mineral absorption and phosphorous utilization (Rafacz-Livingston et al., Reference Rafacz-Livingston, Parsons and Jungk2005; Nezhad et al., Reference Nezhad, Gale-Kandi, Farahvash and Yeganeh2011); and (4) improving gut health through direct effects on epithelial cells (e.g. SCFA are a direct energy source for the growth of epithelial cells). In spite of the demonstrated beneficial effects, using organic acids to improve performance lacks consistency. This can be attributed to various factors such as inclusion rates, the source of the organic acids, and the buffering capacity of other dietary ingredients (Dibner and Buttin, Reference Dibner and Buttin2002; Kim et al., Reference Kim, Kim and Kil2015). Further research should address inconsistency issues and understand their mechanism of action to develop organic acids as effective antibiotic replacements.
Enzymes
Dietary enzymes are biologically active proteins that facilitate chemical breakdown of nutrients to smaller compounds for further digestion and absorption (Thacker, Reference Thacker2013). Various enzymes, derived from microbes (bacteria and fungi) through fermentation, have been used in swine and poultry feeds for the past several years, and their value in enhancing growth and feed efficiency is well noted. The different classes of enzymes commonly employed include phytase, carbohydrases (xylanase, cellulase, α-galactosidase, β-mannanase, α-amylase, and pectinase), and proteases. The effect of various in-feed enzymes in improving the growth and feed efficiency in poultry is well documented and reviewed (Bedford and Schulze, Reference Bedford and Schulze1998; Choct, Reference Choct2006; Selle and Ravindran, Reference Selle and Ravindran2007; Adeola and Cowieson, Reference Adeola and Cowieson2011; Slominski, Reference Slominski2011; Woyengo and Nyachoti, Reference Woyengo and Nyachoti2011).
It is now well accepted that exogenous enzymes act on anti-nutritional factors that are present in plant-based feedstuffs such as phytic acid, NSP, and cell-wall complex carbohydrates. The improved performance that is a result of enzyme supplementation thus has been linked to an increase in the overall digestibility and availability of nutrients for absorption (Bedford, Reference Bedford2000; Verstegen and Williams, Reference Verstegen and Williams2002; Rebolé et al., Reference Rebolé, Ortiz, Rodríguez, Alzeuta, Treviño and Velasco2010). The possible mechanisms of action of in-feed enzymes include the following: (1) increase in the digestibility of nutrients that are otherwise not degraded by host enzymes (e.g. phytic acid); (2) elimination of the nutrient-encapsulating effect of cell-wall polysaccharides and an increase in the availability of starches, amino acids, and minerals; (3) inactivation of anti-nutritional factors (e.g., phytic acid or soluble NSP) and reduced intestinal viscosity; (4) an increase in the solubility of non-soluble NSP and promotion of cecal fermentation; and (5) supplementation of endogenous enzymes that may be in insufficient amounts, especially in young animals in which the digestive system is not fully developed (Choct, Reference Choct2009; Kiarie et al., Reference Kiarie, Romero and Nyachoti2013). In addition to the effects enzymes have on nutrient digestibility, they are also thought to influence the composition of the gut microbiota. The enzyme-induced microbiota changes are mostly indirect and are thought to be mediated by two main mechanisms: (1) reducing the undigested substrates and (2) generating short-chain oligosaccharides from cell-wall NSP with potential prebiotic effects (Bedford, Reference Bedford2000; Bedford and Cowieson, Reference Bedford and Cowieson2012; Kiarie et al., Reference Kiarie, Romero and Nyachoti2013). These mechanisms influence the nutrient supply and intestinal environment thus altering selection pressures on bacterial species (Bedford and Cowieson, Reference Bedford and Cowieson2012; Cheng et al., Reference Cheng, Hao, Xie, Wang, dai, Huang and Yuan2014).
The potential for use of in-feed enzymes, as antibiotic alternatives, to improve performance in poultry is significant. Various meta-analyses conducted corroborate these beneficial effects in broilers upon enzyme supplementation. A meta-analysis performed by Hooge et al. (Reference Hooge, Pierce, McBride and Rigolin2010) showed that supplementation of a dietary multi-enzyme complex involving phytase and NSP enzymes improved final body weight by 3.73% and lowered FCR by 2.64%. Jackson and Hanford (Reference Jackson and Hanford2014) conducted a meta-analysis of seven pen trials investigating the effects of β-mannanase supplementation in male broilers raised to market age. They reported that the weight gain and FCR, analyzed across trials, were improved by 4.2% and 4.8 points, respectively, and concluded that β-mannanase supplementation is effective in broilers. A similar meta-analysis conducted by Swann and Romero (Reference Swann and Romero2014) investigated the beneficial effects of a xylanase, amylase, and protease combination. Their results, based on ten broiler studies, showed that the particular enzyme combination increased the apparent digestibility of undigested crude protein, starch and fat by 22.7, 88.9, and 33.4%, respectively. However, it should be noted that the beneficial effects of enzyme supplementation are sometimes inconsistent owing to the differences in the enzyme type, source, amount of enzyme used, presence of enzyme side effects, diet composition, and genetic variations among animals (Ravindran and Son, Reference Ravindran and Son2011; Cheng et al., Reference Cheng, Hao, Xie, Wang, dai, Huang and Yuan2014).
Phytogenics
Phytogenic feed additives (PFAs), also referred as phytobiotics or botanicals, are natural bioactive compounds that are derived from plants and incorporated into animal feed to enhance productivity (Windisch et al., Reference Windisch, Schedle, Plitzner and Kroismayr2008). A wide range of plants and their products fall under this category and, based on their origin (part of the plant), they can be broadly classified as herbs (flowering, non-woody, non-persistent plants from which leaves and flowers are used) or spices (non-leaf parts of plants, including seeds, fruits, bark or root with intensive taste or smell) (Windisch et al., Reference Windisch, Schedle, Plitzner and Kroismayr2008; Van Der Klis and Vinyeta-Punti, Reference Van Der Klis and Vinyeta-Punti2014). They can be used in solid, dried, and ground form or as extracts (crude or concentrated). Depending on the process used to derive the active ingredients, PFA can also be classified as essential oils (EOs; volatile lipophilic substances obtained by cold extraction or by steam or alcohol distillation) and oleoresins (extracts derived by non-aqueous solvents) (Windisch et al., Reference Windisch, Schedle, Plitzner and Kroismayr2008; Van Der Klis and Vinyeta-Punti, Reference Van Der Klis and Vinyeta-Punti2014). The main bioactive compounds of the PFAs are polyphenols and their composition and concentration vary according to the plant, parts of the plant, geographical origin, harvesting season, environmental factors, storage conditions, and processing techniques (Windisch et al., Reference Windisch, Schedle, Plitzner and Kroismayr2008; Applegate et al., Reference Applegate, Klose, Steiner, Ganner and Schatzmayr2010).
In recent years, PFAs have been used as natural growth promoters in the pig and poultry industries (Windisch et al., Reference Windisch, Schedle, Plitzner and Kroismayr2008; Franz et al., Reference Franz, Baser and Windisch2010). A wide variety of herbs and spices (e.g., thyme, oregano, rosemary, marjoram, yarrow, garlic, ginger, green tea, black cumin, coriander, and cinnamon) have been used in poultry for their potential application as AGP alternatives. Guo et al. (Reference Guo, Kwakkel, Soede, Williams and Verstegen2004) showed a significant increase in body weight gain and improvement in feed efficiency when broilers were given diets supplemented with a mixture of 14 herbs. Similar results were shown with the addition of oregano (Florou-Paneri et al., Reference Florou-Paneri, Giannenas, Christaki, Govaris and Botsoglou2006), dried ground leaves of stevia (Atteh et al., Reference Atteh, Onagbesan, Tona, Decuypere, Geuns and Buyse2008), black cumin seeds (Khalaji et al., Reference Khalaji, Zaghari, Hatami, Hedari-Dastjerdi, Lotfi and Nazarian2011), fermented Ginkgo biloba leaves (Cao et al., Reference Cao, Zhang, Yu, Zhao and Wang2012), and dried and ground Scrophularia striata and Ferulago angulata (Rostami et al., Reference Rostami, Ghasemi and Taherpour2015) to poultry feed. Various plant extracts used as PFAs were also shown to improve the performance of broilers. Research trials conducted with the inclusion of sugar cane extract (El-Abasy et al., Reference El-Abasy, Motobu, Shimura, Na, Kang, Koge, Onodera and Hirota2002), aniseed extract (Durrani et al., Reference Durrani, Sultan, Ahmed, Chand, Khattak and Durrani2007), chestnut wood extract (Schiavone et al., Reference Schiavone, Guo, Tassone, Gasco, Hernandez, Denti and Zoccarato2008), Forsythia suspensa extract (Wang et al., Reference Wang, Piao, Kim, Piao, Shen and Lee2008), and Portulaca oleracea extract (Zhao et al., Reference Zhao, He, Yang and Zhong2013b) showed a significant increase in body weight gain and a lower FCR. In contrast, several other PFAs such as grape pomace, cranberry fruit extract, Macleaya cordata extract, garlic powder, grape seed extract, and yucca extract tested as growth promoters did not show any effects on performance parameters (Goñi et al., Reference Goñi, Brenes, Centeno, Viveros, Saura-Calixto, Rebolé, Arija and Estevez2007; Brenes et al., Reference Brenes, Viveros, Goñi, Centeno, Sáyago-Ayerdy, Arija and Saura-Calixto2008; Leusink et al., Reference Leusink, Rempel, Skura, Berkyto, White, Yang, Rhee, Xuan, Chiu, Silversides, Fitzpatrick and Diarra2010; Juskiewicz et al., Reference Juskiewicz, Gruzauskas, Zdunczyk, Semaskaite, Jankowski, Totilas, Jarule, Sasyte, Zdunczyk, Raceviciute-Stupeliene and Svirmickas2011; Viveros et al., Reference Viveros, Chamorro, Pizarro, Arija, Centeno and Brenes2011; Issa and Omar, Reference Issa and Omar2012; Chamorro et al., Reference Chamorro, Viveros, Centeno, Romero, Arija and Brenes2013).
In addition to herbs and spices, various EOs (thymol; carvacrol; cinnamaldehyde; EOs from clove, coriander, star anise, ginger, garlic, rosemary, turmeric, basil, caraway, lemon, and sage) have been used either individually or as blends to improve animal health and performance. Variable results have been reported with the use of EOs in poultry diets. Including a blend of thymol and cinnamaldehyde in feed which was shown to improve body weight gain in broilers (Tiihonen et al., Reference Tiihonen, Kettunen, Bento, Saarinen, Lahtinen, Ouwehand, Schulze and Rautonen2010; Amerah et al., Reference Amerah, Péron, Zaefarian and Ravindran2011). Similar results were shown when supplementing diets with EO from oregano (Malayoğlu et al., Reference Malayoğlu, Baysal, Misirlioğlu, Polat, Yilmaz and Turan2010; Hashemipour et al., Reference Hashemipour, Kermanshahi, Golian and Veldkamp2013, Reference Hashemipour, Kermanshahi, Golian and Khaksar2014) and coriander (Ghazanfari et al., Reference Ghazanfari, Mohammadi and Moradi2015), blends of clove and cinnamaldehyde (Chalghoumi et al., Reference Chalghoumi, Belgacem, Trabelsi, Bouatour and Bergaoui2013), thymol and EO from star anise (Kim et al., Reference Kim, Lee, Kang and An2016a), and an herbal EO mix (Alçiçek et al., Reference Alçiçek, Bozkurt and Çabuk2004; Khattak et al., Reference Khattak, Ronchi, Castelli and Sparks2014). EO supplementation was also shown to improve feed efficiency as seen by reduced FCRs (Çabuk et al., Reference Çabuk, Bozkurt, Alçiçek, Akbaş and Küçükyilmaz2006; Isabel and Santos, Reference Isabel and Santos2009; Amad et al., Reference Amad, Männer, Wendler, Neumann and Zentek2011; Kim et al., Reference Kim, Lee, Kang and An2016a). In contrast, several other trials did not show any beneficial effects of including EO on performance (Lee et al., Reference Lee, Everts, Kappert, Frehner, Losa and Beynen2003; Basmacioğlu et al., Reference Basmacioğlu, Tokuşoğlu and Ergül2004; Hernández et al., Reference Hernández, Madrid, García, Orengo and Megías2004; Zhang et al., Reference Zhang, Yan, Keen and Waldroup2005; Jang et al., Reference Jang, Ko, Kang and Lee2007). The variations in the results could be attributed to the differences in the composition, type, and origin of the EO that were used, inclusion level, and the environmental conditions of the trials (Franz et al., Reference Franz, Baser and Windisch2010). Nevertheless, one commercial blend of phytonutrients (containing carvacrol, cinnamaldehyde, and capsicum oleoresin) was approved in the EU as the first botanical feed additive for improving performance in broilers. Several research trials performed with this commercial blend demonstrated consistent improvement in growth and feed efficiency (Bravo et al., Reference Bravo, Pirgozliev and Rose2014; Karadas et al., Reference Karadas, Pirgozliev, Rose, Dimitrov, Oduguwa and Bravo2014; Pirgozliev et al., Reference Pirgozliev, Bravo, Mirza and Rose2015). A meta-analysis of 13 broiler studies involving the use of this commercial blend showed that its inclusion in diets increased body weight gain and decreased FCR and mortality (Bravo and Ionescu, Reference Bravo and Ionescu2008).
The mechanism of action of PFAs is not clearly understood and depends greatly upon the composition of the active ingredients in the product being used. In general, the beneficial effects of PFAs are attributed to their antimicrobial and antioxidant properties. The inclusion of PFAs in the diets was shown to alter and stabilize intestinal microflora and reduce microbial toxic metabolites in the gut owing to their direct antimicrobial properties on various pathogenic bacteria, which results in relief from intestinal challenge and immune stress, thus improving performance (Tiihonen et al., Reference Tiihonen, Kettunen, Bento, Saarinen, Lahtinen, Ouwehand, Schulze and Rautonen2010; Viveros et al., Reference Viveros, Chamorro, Pizarro, Arija, Centeno and Brenes2011; Zhang et al., Reference Zhang, Piao, Zhang, Li, Yi, Liu, Li and Wang2013; Zhao et al., Reference Zhao, He, Yang and Zhong2013b; Liu et al., Reference Liu, Liu, Hu, Suo, Zhang, Jin, Feng, Teng and Li2014). Another important beneficial effect of dietary inclusion of PFAs is reduction in oxidative stress and increase in antioxidant activity in various tissues and thus improved health (Basmacioğlu et al., Reference Basmacioğlu, Tokuşoğlu and Ergül2004; Brenes et al., Reference Brenes, Viveros, Goñi, Centeno, Sáyago-Ayerdy, Arija and Saura-Calixto2008; Wang et al., Reference Wang, Piao, Kim, Piao, Shen and Lee2008; Cao et al., Reference Cao, Zhang, Yu, Zhao and Wang2012; Mueller et al., Reference Mueller, Blum, Kluge and Mueller2012; Zhang et al., Reference Zhang, Piao, Zhang, Li, Yi, Liu, Li and Wang2013; Liu et al., Reference Liu, Liu, Hu, Suo, Zhang, Jin, Feng, Teng and Li2014; Settle et al., Reference Settle, Leonard, Falkenstein, Fix, Van Dyke and Klandorf2014). PFAs also exert their action through immunomodulatory effects such as increased proliferation of immune cells, elevated expression of cytokines, and increased antibody titers (Kim et al., Reference Kim, Lillehoj, Lee, Jang and Bravo2010; Lee et al., Reference Lee, Lillehoj, Hong, Jang, Lillehoj, Ionescu, Mazuranok and Bravo2010b; Park et al., Reference Park, Cha, Kang, So, Go, Mun, Ryu and Jang2011; Pourhossein et al., Reference Pourhossein, Qotbi, Seidavi, Laudadio, Centoducati and Tufarelli2015). The addition of PFAs to the diet was also shown to increase intestinal and pancreatic enzyme production and activity and increase bile flow (Lee et al., Reference Lee, Everts, Kappert, Frehner, Losa and Beynen2003; Jang et al., Reference Jang, Ko, Kang and Lee2007; Malayoğlu et al., Reference Malayoğlu, Baysal, Misirlioğlu, Polat, Yilmaz and Turan2010; Hashemipour et al., Reference Hashemipour, Kermanshahi, Golian and Veldkamp2013, Reference Hashemipour, Kermanshahi, Golian and Khaksar2014). PFAs also help maintain and improve gut histology, increase villi height and thus expand absorptive surface of the intestine (Ghazanfari et al., Reference Ghazanfari, Mohammadi and Moradi2015; Murugesan et al., Reference Murugesan, Syed, Haldar and Pender2015). Increase in digestive enzyme secretion and absorption results in improved apparent nutrient digestibility and thus improves performance (Jamroz et al., Reference Jamroz, Orda, Kamel, Wiliczkiewicz, Wertelecki and Skorupinska2003; Hernández et al., Reference Hernández, Madrid, García, Orengo and Megías2004; Jørgensen et al., Reference Jørgensen, Zhao, Theil and Jakobsen2008; Wang et al., Reference Wang, Piao, Kim, Piao, Shen and Lee2008; Amad et al., Reference Amad, Männer, Wendler, Neumann and Zentek2011; Amerah et al., Reference Amerah, Péron, Zaefarian and Ravindran2011; Issa and Omar, Reference Issa and Omar2012). They also might play a role in maintaining the intestinal barrier function as evidenced by the increase in the trans-epithelial electrical resistance of duodenal mucosa of broilers that included thymol in their diets (Placha et al., Reference Placha, Takacova, Ryzner, Cobanova, Laukova, Strompfova, Venglovska and Faix2014).
A growing body of scientific evidence has demonstrated that many of the health-promoting activities of phytochemicals are also mediated through their ability to enhance the host's defense against microbial infections and tumors (Lillehoj et al., Reference Lillehoj, Kim, Bravo and Lee2011). The immune-activating properties of medicinal plants such as dandelion (Taraxacum officinale), mustard (Brassica juncea), and safflower (Carthamus tinctorius) have been evaluated in vitro using avian lymphocytes and macrophages (Lee et al., Reference Lee, Lillehoj, Chun, Tuo, Park, Cho, Lee and Lillehoj2007). All three extracts inhibited tumor cell growth and exhibited antioxidant effects. Further, the safflower extract stimulated chicken lymphocyte proliferation, whereas the mustard extract induced nitric oxide production by macrophages. In a separate study, organic phase extracts from milk thistle (Silybum marianum), turmeric (Curcuma longa), reishi mushroom (Ganoderma lucidum), and shiitake mushroom (Lentinus edodes) were tested for their effects on chicken innate immunity and tumor cell cytotoxicity (Lee et al., Reference Lee, Lee, Lillehoj, Li, Jang, Babu, Park, Kim, Lillehoj, Neumann, Rehberger and Siragusa2010a). In chicken macrophages treated with extracts of turmeric (Curcuma longa) or shiitake mushroom (Lentinus edodes) in vitro (Lee et al., Reference Lee, Lillehoj, Hong, Jang, Lillehoj, Ionescu, Mazuranok and Bravo2010b), the levels of gene transcripts for IL-1β, IL-6, IL-12, IL-18, and TNFSF15 were increased. The phagocytic activity of chicken heterophils was shown to be significantly improved with the addition of non-dialyzable materials of cranberry extract at 4 mg ml−1 concentration (Islam et al., Reference Islam, Oomah and Diarra2016). Cinnamaldehyde ((2E)-3-phenylprop-2-enal) is a constituent of cinnamon (Cinnamomum cassia), a widely used flavoring compound that has been traditionally used to treat human diseases, including dyspepsia, gastritis, and inflammation. Chicken spleen lymphocytes that were stimulated in vitro with cinnamaldehyde showed good cell proliferation, and cinnamaldehyde activated cultured macrophages to produce higher nitric oxide levels (Lee et al., Reference Lee, Lillehoj, Jang, Lee, Park, Bravo and Lillehoj2011b). The effects of carvacrol, cinnamaldehyde, and Capsicum oleoresin on the regulation of the expression of genes associated with immunology, physiology, and metabolism were investigated in chickens using high-throughput microarray analysis (Lillehoj et al., Reference Lillehoj, Kim, Bravo and Lee2011). These studies revealed that Capsicum oleoresin stimulated a great number of gene changes when compared with unsupplemented controls, and many of the altered genes were associated with metabolism and immunity. The most reliable genetic network induced by dietary cinnamaldehyde treatment was related to the functions of antigen presentation, humoral immunity, and inflammatory disease. Further studies to delineate the intestinal immune pathways affected by phytochemical feeding were conducted by mRNA microarray hybridization (Kim et al., Reference Kim, Lillehoj, Lee, Jang and Bravo2010). When compared with chickens fed an unsupplemented diet, carvacrol-fed chickens showed altered levels of 74 gene transcripts in gut lymphocytes (26 increased, 48 decreased), cinnamaldehyde supplementation was associated with altered levels of 62 mRNAs (31 increased, 31 decreased), and Capsicum oleoresin-fed chickens had altered levels of 254 mRNAs (98 increased, 156 decreased), compared with unsupplemented controls. Among the transcripts that showed greater than twofold altered expression levels, most were encoded by genes associated with metabolic pathways. In the case of Capsicum oleoresin, the transcripts included pathways for lipid metabolism, small molecule biochemistry, and cancer. In another investigation, global gene expression analysis by microarray hybridization identified 1810 transcripts (677 increased, 1133 decreased) whose levels were significantly altered in intestinal lymphocytes of anethole-fed birds when compared with unsupplemented controls (Kim et al., Reference Kim, Lillehoj, Lee, Jang, Park, Min, Lillehoj and Bravo2013a). From these, 576 corresponding genes were identified that were related to the inflammatory response. A similar analysis was reported for the garlic metabolites, propyl thiosulphinate PTS) and PTS oxide (PTSO) (Kim et al., Reference Kim, Lillehoj, Lee, Lillehoj and Bravo2013b). In that study, 1227 transcripts (552 increased, 675 decreased) were identified in intestinal lymphocytes whose levels were significantly altered in PTS/PTSO-fed birds when compared with unsupplemented controls. Many of these transcripts were encoded by genes related to innate immunity, including Toll-like receptor 3 (TLR3), TLR5, and nuclear factor (NF)-κB.
Hyperimmune egg yolk antibodies
Hyperimmune egg yolk antibodies (IgY), produced by repeated immunization of hens with specific antigens and collection of antibodies thereafter from their egg yolks, have been commonly employed in the prevention and treatment of various enteric diseases in humans and animals (Gadde et al., Reference Gadde, Rathinam and Lillehoj2015). Limited research exists on the use of egg yolk antibodies as viable alternatives to AGP in improving growth and feed efficiency in poultry (Cook, Reference Cook2004). Earlier studies were focused on generation of egg antibodies in breeding hens that could be passively transferred to the progeny and improve their productivity. Pimentel and Cook (Reference Pimentel and Cook1988) and Pimentel et al. (Reference Pimentel, Cook and Jonsson1991) showed that progeny from hens injected with jack bean urease had improved body weight at 3 weeks of age. It was proposed that urease antibodies maternally transferred to the progeny decreased ammonia production in the intestinal tract by inhibiting bacterial urease enzyme and improving growth. As IgY technology evolved, research trials conducted later on involved the use of antibodies in feed to improve performance or enhance host immunity (Lee et al., Reference Lee, Lillehoj, Park, Jang, Morales, Garcia, Lucio, Larios, Victoria, Marrufo and Lillehoj2009a, Reference Lee, Lillehoj, Park, Jang, Morales, Garcia, Lucio, Larios, Victoria, Marrufo and Lillehojb). The majority of these studies encompassed the use of antibodies that were raised against components involved in the immune regulation of growth. The growth suppression associated with immune stimulation is well established, and it is hypothesized that interleukin 1 (IL-1) released during inflammation causes anorexia (through the release of neuropeptides like cholecystokinin (CCK), neuropeptide Y into gut lumen) and muscle wasting (Goldberg et al., Reference Goldberg, Baracos, Rodemann, Waxman and Dinarello1984; Klasing et al., Reference Klasing, Laurin, Peng and Fry1987). Cook (Reference Cook2004) reported that hyperimmune egg yolk antibodies raised against various neuropeptides (CCK, neuropeptide Y) improved body weight and feed efficiency when fed to broiler chickens up to 3 weeks of age. They showed that supplementing diets with egg powder containing CCK antibodies at 0.25 g kg−1 dose improved the feed conversion efficiency by 13 points compared with that of birds fed egg powder from unimmunized hens. Similar results were shown in a series of trials in which chicks were fed dried egg yolk powder from hens vaccinated with neuropeptide Y or from control unimmunized hens. The average improvement in weight gain and FCR at 3 weeks of age was shown to be 9% and 8 points, respectively, compared with controls (Cook, Reference Cook2004). Eicosanoids are also believed to play a proinflammatory role in immune stimulation, and supplementing feed with egg antibodies (BIG™) developed against phospholipase A2 (an enzyme involved in eicosanoid synthesis) for 3 weeks improved the mean weight gain of broilers by 5.4% and the FCR by 6.2 points (Cook, Reference Cook2001, Reference Cook2002).
The use of egg yolk antibodies offers several advantages. Large quantities of antibodies can be produced in laying hens and non-invasively collected. Their use is environmentally friendly, less toxic and does not select for resistance. Although the existing results seemed encouraging, much more research is needed on using egg antibodies for growth promotion in poultry.
Antimicrobial peptides
AMPs are widely distributed, small, gene-encoded peptides that have germicidal properties. They have been seen in all kingdoms of life and have shown activity against a wide range of pathogens such as Gram-negative and Gram-positive bacteria, fungi, enveloped viruses, and parasites (Koczulla and Bals, Reference Koczulla and Bals2003; Li et al., Reference Li, Xiang, Zhang, Huang and Su2012; Kim et al., Reference Kim, Lillehoh and Gay2016b). Mature AMPs generally contain 12–100 amino acids, are rich in hydrophobic cationic residues, and have an amphipathic structure that facilitates interaction with negatively charged membranes of microbials as well as other cellular targets (Yeaman and Yount, Reference Yeaman and Yount2007; Linde et al., Reference Linde, Ross, Davis, Dib, Blecha and Melgarejo2008; Wang et al., Reference Wang, Yan, Dang, Xie, Yan, Yan, Sun, Zhang, Ma, Zhao, Jia, Zhu, Chen and Wang2014). To date, over 2600 endogenous AMPs have been isolated and many more synthetic analogues were reported in various publications (http://aps.unmc.edu/AP/main.php; Fosgerau and Hoffmann, Reference Fosgerau and Hoffmann2015). The studies that have been done on AMPs and their applications in poultry have been mostly focused on their protective potential against diverse pathogens causing infectious diseases rather than growth-promoting activities. However, a few research trials investigating the effect of AMPs on poultry growth performance, intestinal morphology, and gut microbiology as potential AGP alternatives have been explored. One such trial demonstrated that supplementing with yeast-expressed cecropin A (1-11)-D(12-37)-Asn (CADN), a chimeric peptide derived from insects, in poultry diets increased weight gain, feed intake, feed:gain ratio, and intestinal villus height while decreasing aerobic bacterial counts in both jejunal and cecal digests (Wen and He, Reference Wen and He2012). In consistency with the previous result, Choi et al. (Reference Choi, Ingale, Kim, Park, Kwon and Chae2013) reported the beneficial effects of diets supplemented with a chemically synthesized AMP-P5, analog of hybrid AMP cecropin A(1-8)-magainin 2(1-12) (CAMA), on chicken performance, nutrient retention, intestinal morphology, as well as excreta and intestinal microflora. One Chinese research group investigated the effects of naturally synthesized AMPs obtained from swine and rabbit. The AMPs were extracted from swine gut and rabbit sacculus rotundus, respectively, and were orally inoculated or supplemented in water or diets. They reported that the birds that were given naturally synthesized AMPs showed improvement in growth performance, intestinal ability to absorb nutrients and mucosal immune parameters such as intraepithelial lymphocytes or mast cell counts, and in secretory IgA levels when compared with unsupplemented or non-inoculated birds (Liu et al., Reference Liu, She, Wang, Bao, Zhang, Luo, Hu, Ding, Wang and Peng2008; Bao et al., Reference Bao, She, Liu, Zhang, Peng, Luo, Yue, Ding, Hu, Liu and Zhai2009; Wang et al., Reference Wang, Ma, She, Sun, Liu, Hu, Liu, Yang and Peng2009).
Based on the origins of AMPs, there is a particular group of AMPs called bacteriocins. Bacteriocins are defined as ribosomally synthesized peptides that are secreted by various bacteria that have antibacterial activity against other similar or closely related bacteria. In the past, bacteriocins were mostly used as food preservatives and were believed to be produced only by specific bacterial strains (Cleveland et al., Reference Cleveland, Montville, Nes and Chikindas2001). Thus, its production had been considered an important feature in the selection of probiotic strains, but now one or more bacteriocins have been identified and believed to exist in all species of bacteria and archaea (Cotter et al., Reference Cotter, Hill and Ross2005; Willey and van der Donk, Reference Willey and van der Donk2007). Currently, 177 bacteriocins have been identified in 31 genera, including Gram-positive and Gram-negative bacteria as well as archaea (http://bactibase.pfba-lab-tun.org/main.php). They are mainly cationic, hydrophobic, or amphiphilic like other AMPs (Riley and Wertz, Reference Riley and Wertz2002). Generally, they have been shown to possess a relatively narrow spectrum of antimicrobial activity when compared with AMPs produced by non-bacterial origin. One of the most reported bacteriocins as a dietary supplement in poultry is divercin AS7, which is produced by Carnobacterium divergens AS7, a lactic acid-producing bacterium isolated from fish, which has been extensively studied by Józefiak and colleagues. The authors have focused on the application of divercin AS7 to improve growth performance, nutrient retention, intestinal histomorphology, and balance of gastrointestinal microbiota. They demonstrated that supplementing broiler diets with divercin AS7 has an in vivo growth-promoting effect, increasing digestibility as well as a modulatory effect on intestinal microbiota (Józefiak et al., Reference Józefiak, Sip, Kaczmarek and Rutkowski2010, Reference Józefiak, Sip, Rawski, Steiner and Rutkowski2011a, Reference Jozefiak, Sip, Rawski, Rutkowski, Kaczmarek, Hojberg, Jensen and Engbergb, Reference Józefiak, Sip, Rutkowski, Rawski, Kaczmarek, Wołuń-Cholewa, Engberg and Højberg2012). Supplementation of divercin AS7 reduced intestinal digesta pH in a series of their studies, which reflected the activity of the gastrointestinal microbiota and digestion physiology (Engberg et al., Reference Engberg, Hedemann and Jensen2002). In addition, dietary nisin, which is produced by Lactococcus lactis and is the sole bacteriocin approved for use as a food additive by the FDA, exerted a modulatory effect on the microbial ecology of the gastrointestinal tract with decreased counts of Bacteroides and Enterobacteriacae, but unchanged counts of Clostridium perfringens, Lactobacillus spp.,Enterococcus spp., and total bacteria (Józefiak et al., Reference Józefiak, Kierończyk, Juśkiewicz, Zduńczyk, Rawski, Długosz, Sip and Højberg2013). Albusin B, which is another bacteriocin that is produced by Ruminococcus albus 7, was added to poultry feed and also showed improved growth performance, increased intestinal absorption and Lactobacillus counts, modulated lipid metabolism, and activated systemic antioxidant defense (Wang et al., Reference Wang, Yu, Hsieh, Chen, Chen and Chen2011, Reference Wang, Li, Chou, Hsieh, Chen and Chen2013a).
Despite the fact that limited research exists on the use of AMPs as alternatives to AGP, collectively dietary supplementation of AMPs in poultry seems to affect the birds in a positive way by improving their intestinal balance and creating gut micro-ecological conditions that suppress harmful microorganisms like Clostridium spp. and coliforms while favoring beneficial microorganisms like Lactobacillus spp. (Ohh et al., Reference Ohh, Shinde, Jin, Choi, Hahn, Lim, Kim, Park, Hahm and Chae2009). In conclusion, the AMPs including bacteriocins have the potential to considerably enhance poultry health as alternatives to AGP and their potential might be improved when a number of obstacles such as high production cost, resistance development, and instability of the AMPs are addressed in the future.
Bacteriophages
Bacteriophages, which were discovered in the early 1900s (Twort, Reference Twort1915; d'Herelle, Reference d'Herelle1917), are highly species-specific viruses that kill bacteria through the production of endolysins and the subsequent lysis of the bacterial cells (Joerger, Reference Joerger2003; Huff et al., Reference Huff, Huff, Rath, Balog and Donoghue2005). Bacteriophages can be considered safe antibiotic alternatives as they exhibit no activity against animal and plant cells. They have been used to prevent and treat various bacterial diseases in humans and animals (Huff et al., Reference Huff, Huff, Rath, Balog and Donoghue2003; Miller et al., Reference Miller, Skinner, Sulakvelidze, Mathis and Hofacre2010). A significant amount of research was also done on their use in control of food-borne pathogens on agricultural and poultry products (Goode et al., Reference Goode, Allen and Barrow2003; Huff et al., Reference Huff, Huff, Rath, Balog, Donoghue, Beier, Pillai, Phillips and Ziprin2004). Very few studies demonstrated the effects of supplementing diets with bacteriophages on growth performance. Zhao et al. (Reference Zhao, Baek and Kim2012) evaluated the effects in laying hens and reported that incorporating 0.035 or 0.05% of bacteriophages in their diet significantly improved egg production. Increased body weight gain and reduced FCR were reported in broilers given diets supplemented with 0.10 and 0.15% (Kim et al., Reference Kim, Kim, Kim and Kim2013c) or 0.5 g kg−1 of bacteriophages, respectively (Wang et al., Reference Wang, Yan, Lee and Kim2013b). However, further research is needed to establish the performance effects of bacteriophages and make their use practical in poultry production systems.
Clay
Clay minerals (also designated as phyllosilicates) are formed by a net of stratified tetrahedral and octahedral layers containing molecules of silicon, aluminum, and oxygen, and interconnected by hydrogen bonds or a group of cations (Vondruskova et al., Reference Vondruskova, Slamova, Trckova, Zraly and Pavlik2010). The natural extracted clays (bentonite, zeolite, kaolin, etc.) are a mixture of various clay minerals that differ in chemical composition (Vondruskova et al., Reference Vondruskova, Slamova, Trckova, Zraly and Pavlik2010). Clay minerals, because of their stratified structure, have great adsorption capacity and can bind aflatoxins, plant metabolites, heavy metals, enterotoxins, and pathogens. The factors affecting the extent of adsorption include the chemistry of the clay minerals, the fine structure of the clay particles, and their surface properties, pH, dosage, and exposure time (Thacker, Reference Thacker2013). Many research trials were conducted to show the antibacterial and detoxifying effects of clay in poultry (Kubena et al., Reference Kubena, Harvey, Bailey, Buckley and Rottinghaus1998; Phillips, Reference Phillips1999; Fowler et al., Reference Fowler, Li and Bailey2015), but very few trials investigated their growth-promoting effects. Xia et al. (Reference Xia, Hu and Xu2004) reported that including copper-bearing montmorillonite in broiler diets significantly improved growth performance, reduced Escherichia coli and Clostridium spp. counts in the intestine, and increased intestinal digestive enzyme activity. Dietary inclusion of hydrated aluminosilicate (5 g kg−1) significantly increased body weight gain of broilers at 1 and 3 weeks of age and increased serum amylase and lactate dehydrogenase activity (Prvulović et al., Reference Prvulović, Kojić, Grubor-Lajsić and Kosarcić2008). Similar improvement results in performance were shown upon supplementing diets with kaolin, bentonite, zeolite (Katouli et al., Reference Katouli, Boldaji, Dastar and Hassani2010), clay (Ani et al., Reference Ani, Ogbu and Iloh2014), and kaolin (Jorge de Lemos et al., Reference Jorge de Lemos, Calixto, Alves, Santos de Souza, Moura and Reis2015). Wu et al. (Reference Wu, Wu, Zhou, Ahmad and Wang2013) showed that adding clinoptilolite to diets improved antioxidant capacity in broilers as evidenced by increased glutathione peroxidase, catalase, and total superoxide dismutase activities and decreased malondialdehyde content of liver. However, results of including clay minerals appeared to be inconsistent, as some research trials showed no effect on performance (Wu et al., Reference Wu, Wu, Zhou, Ahmad and Wang2013).
The mechanism by which clays and clay minerals influence growth is unclear, but it depends largely on their ability to physically bind and remove toxins, anti-nutritional components, and pathogenic organisms. This results in reducing microbial metabolites, toxins, and enzymes in the intestine and thus preventing irritation and damage and improving morphological characteristics of the intestinal mucosa (Xia et al., Reference Xia, Hu and Xu2004; Jorge de Lemos et al., Reference Jorge de Lemos, Calixto, Alves, Santos de Souza, Moura and Reis2015) and thus performance. The inclusion of clay was also shown to improve nutrient digestibility by reducing digest transit time and also decreasing litter moisture (Olver, Reference Olver1997; Jorge de Lemos et al., Reference Jorge de Lemos, Calixto, Alves, Santos de Souza, Moura and Reis2015). Further research should be done on the role of clay as a potential alternative to AGP and its effects when combined with other products.
Metals
Heavy metals such as copper, zinc, iron, selenium and manganese, often referred as trace minerals in animal nutrition, are extensively used in food animal production to maintain general health and normal physiology (Scott, Reference Scott2012). They play a vital role in growth and metabolism as they are critical for many digestive, physiological, and biosynthetic processes (Richards et al., Reference Richards, Zhao, Harrell, Atwell and Dibner2010; Attia et al., Reference Attia, Qota, Zeweil, Bovera, Abd Al-Hamid and Sahledom2012). Traditionally, heavy metals have been supplemented in animal diets in the form of inorganic salts such as carbonates, chlorides, oxides, and sulfates (Pierce et al., Reference Pierce, Ao, Charlton and Tucker2009; Attia et al., Reference Attia, Qota, Zeweil, Bovera, Abd Al-Hamid and Sahledom2012), but chelated or organic forms have also been used lately. The use of trace minerals to increase animal productivity and performance has been gaining importance in the recent years and they are being substituted in levels beyond the recommended nutritional requirements. Copper, an essential trace mineral, plays a significant role in hemoglobin synthesis, angiogenesis, connective tissue, bone development, and more importantly serves as a cofactor for many metabolic enzymes (Brainer et al., Reference Brainer, Menten, Vale and Morais2003; Richards et al., Reference Richards, Zhao, Harrell, Atwell and Dibner2010; Vasanth et al., Reference Vasanth, Dipu, Mercy and Shyama2015). The use of copper as growth promotant in poultry diets has been well demonstrated. Supplementation of diets with copper sulfate, citrate, or carbonate at levels ranging from 125 to 250 mg Cu kg−1 showed improvement in body weight and feed efficiency (Hoda and Maha, Reference Hoda and Maha1995; Pesti and Bakalli, Reference Pesti and Bakalli1996). Ewing et al. (Reference Ewing, Pesti, Bakalli and Menten1998) demonstrated that addition of cupric sulfate pentahydrate and copper oxychloride to the broiler diets increased weight gain by 4.9% and cupric citrate increased weight gain by 9.1% compared with non-supplemented controls. In-feed supplementation of tribasic copper chloride or copper sulfate was also shown to significantly increase average daily gain and carcass weight in broilers (Arias and Koutsos, Reference Arias and Koutsos2006; Lu et al., Reference Lu, Wang, Zhang, Steward, Luo and Liu2010). Zinc is another important essential trace mineral that has been used to improve performance in poultry. Zinc serves as a cofactor for several cellular enzymes and transcription factors and plays an essential role in cell proliferation, immune response, reproduction, gene regulation, and defense against oxidative stress and damage (Richards et al., Reference Richards, Zhao, Harrell, Atwell and Dibner2010). Supplementation of zinc sulfate up to 80 mg kg−1 in the basal diets was shown to significantly improve body weight gain of broilers but no differences in mortality and feed efficiency were observed (Burrell et al., Reference Burrell, Dozier, Davis, Compton, Freeman, Vendrell and Ward2004). Similar improvements in performance were shown when broilers were given diets with zinc oxide along with sodium selenite for 4 weeks (Fawzy et al., Reference Fawzy, El-Sadawi, El-Dien and Mohamed2016). In contrast to these studies, several other research trials investigating the beneficial effects of zinc supplementation did not show any performance effects, but in general observed improved immune status of the birds (Sunder et al., Reference Sunder, Panda, Gopinath, Rama Rao, Raju, Reddy and Vijay Kumar2008; Yogesh et al., Reference Yogesh, Deo, Shrivastava, Mandal, Wadhwa and Singh2013). The growth promotant effect seen following in-feed supplementation of some of the metals such as zinc, copper can be attributed to their antimicrobial properties (Cromwell, Reference Cromwell, Miller, Ullrey and Lewis1991; Brainer et al., Reference Brainer, Menten, Vale and Morais2003; Yazdankhah et al., Reference Yazdankhah, Rudi and Bernhoft2014). From studies conducted in pigs, it was proposed that zinc and copper alter the intestinal microbiota by reducing the levels of both commensal and pathogenic bacteria, and also by reducing fermentation loss of nutrients (Højberg et al., Reference Højberg, Canibe, Poulsen, Hedemann and Jensen2005; Yazdankhah et al., Reference Yazdankhah, Rudi and Bernhoft2014). Use of metals as growth promotants should be adapted with caution as they may come with some disadvantages. Inclusion of metals in excess amounts raises environmental concerns in terms of their accumulation in soil and surface water (Burrell et al., Reference Burrell, Dozier, Davis, Compton, Freeman, Vendrell and Ward2004). Also, excess use of metals has been shown to develop metal resistance with concomitant cross-resistance to antibiotics among enteric bacteria in farm animals (Yazdankhah et al., Reference Yazdankhah, Rudi and Bernhoft2014).
Conclusions
Owing to the rise in consumer demand for livestock products from antibiotic-free production systems, there exists a great need for the development of antibiotic alternatives that can help improve performance and maintain optimal health of food animals. Several products have been evaluated in poultry over the past several years for their potential to replace antibiotics. Though the beneficial effects of many of the alternatives tested have been well demonstrated, there is the general consensus that these products lack consistency, as results vary greatly from farm to farm. Care must be taken in the choice of alternatives, such that they fit the needs of the individual production program. Further research is needed regarding understanding their mechanism of action, identifying means to standardize the effects, improving delivery methods (e.g. microencapsulation) for site-targeted delivery, and increasing their in vivo efficacy. Combinations of products may prove more beneficial than using them alone to achieve an effect similar to that of antibiotics. Using optimal combinations of various alternatives coupled with good management and husbandry practices will be the key to maximizing performance and maintaining animal productivity, while we move forward with the ultimate goal of reducing antibiotic use in the animal industry.
Acknowledgment
This project was supported by ARS-USDA CRIS in Animal Health (NP103) # 8042-32000-107-00D.