Cultural shellworks (or shell mounds, sensu lato) are among the most conspicuous and durable material features of ancient coastal Indigenous lifeways in the Americas (Grier et al. Reference Grier, Angelbeck and McLay2017; Klokler Reference Klokler2017; Sanger et al. Reference Sanger, Ouitmyer, Colaninno, Cannarozzi and Ruhl2020; Schwadron Reference Schwadron2017). Recognizing repeated or continuous occupation at many Native shell mounds spanning centuries to millennia, archaeologists have come to understand them as “persistent places” that preserve social memory and reinforce social relationships by linking people to ancestors and ancestral landscapes (Gamble Reference Gamble2017; Schlanger Reference Schlanger, Rossignol and Wandsnider1992; Thompson Reference Thompson, Thomas and Sanger2010). This is particularly true along the Gulf Coast of Florida, where many Native shell mounds were constructed at monumental scale and were situated at prominent points on the landscape bearing histories of ancestral land use (Figure 1). Among these sites are several elaborately terraformed civic-ceremonial centers featuring platform mounds, mortuaries, middens, and plazas (Pluckhahn and Thompson Reference Pluckhahn and Thompson2018; Sasssaman et al. Reference Sassaman, Blessing, Goodwin, Jenkins, Mahar, Boucher, Barbour and Donop2020; Wallis and McFadden Reference Wallis and McFadden2016; Wallis et al. Reference Wallis, LeFebvre, Blessing and McFadden2022). These mound centers were repeatedly altered and expanded through large-scale coordination of resources and labor over centuries to millennia, representing what Thompson and Pluckhahn (Reference Thompson and Pluckhahn2012:50) term “persistent monumental places” where ceremonial aggregations involved mound building and other terraforming. By terraforming we mean the anthropogenic construction and modification of durable physical environments to suit the builders’ broader vision of the landscape (Grier et al. Reference Grier, Angelbeck and McLay2017). Such actions serve economic and political purposes, imbue the landscape with symbolic power, and significantly modify topography and sedimentary properties—producing dialectical relationships between civic-ceremonial infrastructure and ecological conditions (Randall and Sassaman Reference Randall and Sassaman2017).

Figure 1. Reference maps depicting the physiographic setting and locations of sites mentioned in text: (a) map of Florida with bathymetric contours and relic marine terraces displayed in grayscale. Light-gray regions are less than 10.5 m, medium-gray regions are more than 10.5 and less than 30.5 m, and dark-gray regions are more than 30.5 m; (b) map of Tampa Bay depicting major sub-basins and the locations of sites mentioned in text; (c) modern orthophotography of Bishop Harbor, Harbor Key (8MA15) and associated Native shellwork sites. (Color online)
Within coastal-estuarine contexts, long-term histories of occupation, ceremonial aggregation, and shell terraforming are inherently intertwined with morphodynamic processes—time- and scale-dependent feedback loops between topography and fluid dynamics—where sea-level rise, hurricane landfalls, tidal flow, and waves influence the formation and taphonomy of shell-bearing sites (see Cowell and Thom Reference Cowell, Thom, Carter and Woodroffe1995). In turn, we propose that long-term interactions between coastal processes and shellworks alter biophysical conditions within encompassing coastal systems and influence trajectories of estuary development. However, although Gulf Coast archaeologists have amply considered how ancient Native societies adapted settlement patterns and subsistence strategies to trends and perturbations of Holocene coastal evolution (e.g., Marquardt et al. Reference Marquardt, Walker, Thompson, Savarese, Roberts Thompson and Newsom2022; McFadden Reference McFadden2016; Sassaman et al. Reference Sassaman, Blessing, Goodwin, Jenkins, Mahar, Boucher, Barbour and Donop2020), the roles of coastal processes in site formation histories are poorly understood, and the effects of shell-terraformed sites on biophysical conditions within estuaries have gone essentially uninvestigated.
We attribute this problem to several interrelated issues. First, many Gulf Coast shell mounds were destroyed during the late nineteenth and early twentieth centuries to produce material for road construction, and major portions of Gulf Coast estuaries have been heavily altered by dredge-and-fill operations, seawall construction, ditching, and other civil-engineering impacts. Second, although detailed stratigraphic-sedimentary analyses have advanced the geoarchaeological understanding of terraformed earthworks throughout the Eastern Woodlands (e.g., Arco et al. Reference Arco, Adelsberger, Hung and Kidder2006; Sherwood and Kidder Reference Sherwood and Kidder2011; Van Nest et al. Reference Van Nest, Charles, Buikstra and Asch2001), there is a lack of rigorous sedimentological research on shell mounds, which drastically limits their empirical categorization, comparison, and interpretation. For example, we note that Marquardt's (Reference Marquardt2010:551) call for a “sediment-oriented approach to the study of [shell] mound deposits” has gone largely unanswered (for an exception, see McFadden Reference McFadden2016). Third, normative interpretations of Indigenous shellworks as unintentional accumulations of quotidian subsistence refuse predominated in the archaeological literature from the mid-nineteenth century until the 1990s (Claassen Reference Claassen1991; Waselkov Reference Waselkov1987). Late nineteenth-century explanations for shell mound formation on the Florida Gulf Coast by Stearns (Reference Stearns1870, Reference Stearns1872) and Walker (Reference Walker1880:416–418) proposed that they formed “due to chance rather than design” through refuse disposal on the periphery of settlements and the intermittent relocation of habitation areas to higher ground atop previous refuse dumps—resulting in the accumulation of massive “shell heaps” on natural points or islands where settlement footprints were spatially constrained. Although seldom cited, these early explanations continue to function as implicit null models for shellwork studies on the Florida Gulf Coast (see Marquardt Reference Marquardt2010) and discourage the conceptualization of Native shellworks as planned architecture or coastal infrastructure. Lastly, despite early observations by Cushing (Reference Cushing1897) that shell-terraformed islands in southwest Florida altered tidal flow, sediment transport, and estuarine habitat distributions, archaeologists in the region have historically sampled and studied coastal shellworks in relative isolation from their surrounding coastal environments (for exceptions, see Cook-Hale et al. Reference Cook-Hale, Hale and Garrison2019; McFadden Reference McFadden2016). We see this as part of a larger methodological disjuncture between terrestrial and aquatic/marine archaeological practice (see Halligan Reference Halligan2021) and as a manifestation of the entrenched dualism in Western thought between land and sea that parallels deeper problematic dualisms between nature and culture or subject and object (Collingwood Reference Collingwood1945:7; Sharp Reference Sharp2002:152–177).
In this study, we apply a coastal-geoarchaeological approach drawing from Sherwood and Kidder's (Reference Sherwood and Kidder2011) integrated geoarchaeological approach, coastal morphodynamic principles (Cowell and Thom Reference Cowell, Thom, Carter and Woodroffe1995), and longitudinal study of surficial and buried sediments in the region. Our approach emphasizes (1) seascape-scale sampling and analyses, (2) the application of well-supported sea-level reconstructions, (3) sampling across the modern tidal frame, and (4) rigorous sedimentological and mollusk-zooarchaeological analyses that enable quantitative categorization, comparison, and interpretation of sediment deposits. Our study is centered at Bishop Harbor, an inshore sub-basin of Tampa Bay on the central peninsular Gulf Coast of Florida (Figure 1). Like many of Tampa Bay's inshore bayous, Bishop Harbor is partially blocked from the open bay by supratidal landforms bearing Indigenous shellworks, and most conspicuously by Harbor Key (8MA15)—a Native shell mound complex containing a steep-sided, 5.5 m tall pyramidal platform mound, a lower (1.5 m tall) platform mound, a sand mortuary mound, a well-defined plaza, and a linear ridge of shell-bearing deposits along the bay shoreline (Burger Reference Burger1979). We synthesize stratigraphic, sedimentological, mollusk-zooarchaeological, and radiometric datasets from sediment cores, test excavations, and auger probes to meet three major research objectives: (1) situating the Harbor Key shellworks within a seascape-scale stratigraphic and paleoenvironmental framework, (2) resolving the composition and chronostratigraphy of the Harbor Key mound complex, and (3) reconstructing cultural and natural site-formation processes, with a focus on interactions among shellworks, coastal processes, and Late Holocene coastal evolution.
Tampa Bay Estuary
Tampa Bay is a large (1,000 km2) and shallow (4 m mean depth) microtidal estuary on the central peninsular Gulf Coast of Florida (Figure 1). The estuary system is situated near the center of the Florida carbonate platform (Scott Reference Scott, Buster and Holmes2011), which forms a vast low-gradient continental shelf extending westward beneath the Gulf of Mexico. Much of this shelf was sub-aerially exposed during the Last Glacial Maximum and was subsequently inundated by deglacial and Holocene sea-level rise (Joy Reference Joy2019). Tampa Bay's enigmatic morphology was formed by the estuarine flooding of antecedent karst depressions, which produced numerous interconnected sub-basins separated by areas of more durable limestone (Hine et al. Reference Hine, Suthard, Locker, Cunningham, Duncan, Evans and Morton2009). Before incipient marine flooding about 7500 BP, Tampa Bay's karst landscape supported persistent freshwater wetlands, including a paleo-lake system that once occupied the Middle Tampa Bay basin (Cronin et al. Reference Cronin, Brooks, Hastings, Larson, Hine, Locker and Southard2007; Willard et al. Reference Willard, Bernhardt, Brooks, Cronin, Edgar and Larson2007). Consistent with Thulman's (Reference Thulman2009) settlement model, artifact and site distributions suggest significant human occupation of the Tampa Bay region during the terminal Pleistocene and Early Holocene (Anderson et al. Reference Anderson, Echeverry, Shane Miller, White, Yerka, Kansa, Kansa, Thulman and Ervan2019; Wisenbaker and Daniel Reference Wisenbaker and Daniel1987). A widespread transition to estuarine conditions in Tampa Bay during the mid-Holocene, about 6500 BP (Cronin et al. Reference Cronin, Brooks, Hastings, Larson, Hine, Locker and Southard2007; van Soelen et al. Reference van Soelen, Lammertsma, Cremer, Donders, Sangiorgi, Brooks and Larson2010), corresponded with a climatic optimum that drove pine-forest expansion and the establishment of vast estuarine wetland systems (Jackson et al. Reference Jackson, Wang, Pluckhahn, Rogers and Thompson2023; van Soelen et al. Reference van Soelen, Brooks, Larson, Sinninghe Damste and Reichart2012). Although older sites may be preserved in submerged contexts, the earliest preserved Indigenous shell-bearing sites in the region date to the mid-Holocene, yielding fiber-tempered ceramics along with shell- and bone-tool technologies (Saunders and Russo Reference Saunders and Russo2011). Continued sea-level rise throughout the Late Holocene—particularly after AD 200—exposed estuarine sub-basins to increased wave forcing and converted marsh-dominated tidal creek systems to open sand flats and seagrass meadows, which currently dominate shallow subtidal and intertidal zones (Jackson et al. Reference Jackson, Wang, Pluckhahn, Rogers and Thompson2023; also see Goodbred et al. Reference Goodbred, Wright and Hine1998). Most large shell-terraformed Native settlements on the peninsular Gulf Coast were constructed during the first millennium, with major intensifications in mound building after around AD 500 (Austin et al. Reference Austin, Mitchem, Weisman, Wallis and Randall2014; Pluckhahn and Thompson Reference Pluckhahn and Thompson2018; Wallis and McFadden Reference Wallis and McFadden2016). Within Tampa Bay, shell mound sites are commonly situated at prominent points near the mouths of major tidal bayous; in many cases they armor or comprise the seaward-most supratidal landforms that protect inshore sub-basins from relatively energetic open bay waves. As noted by Pluckhahn and colleagues (Reference Pluckhahn, Jackson and Rogers2022a), this pattern appears exaggerated on the bay's southeastern shoreline.
Bishop Harbor and the Harbor Key Mound Complex (8MA15)
Bishop Harbor is a shallow estuarine sub-basin on the southeastern shore of Tampa Bay encompassing an approximately 3.5 km2 water body dominated by tidal flats, oyster reefs, and mangrove swamps (Figure 1; Supplemental Figure 1). All supratidal landforms within the basin are associated with shell-bearing archaeological sites (Burger Reference Burger1979). Bishop Harbor is connected to Tampa Bay by a narrow (150 m wide) inlet that is constricted on both ends by Native shellworks: on the southwest by the Mariposa Key (8MA302) and on the northeast by Harbor Key (8MA15).
Despite the unusual integrity and conspicuous arrangement of Harbor Key's mound features, as well as a significant amount of previous digging, our work represents the first systematic testing at the site. Prolific and widely read antiquarians of the late nineteenth-century Gulf Coast did not mention Harbor Key. Although both Sylvanus T. Walker (Reference Walker1880) and Clarence B. Moore (Reference Moore1900) excavated at shell mounds along the southeastern shore of Tampa Bay (e.g., Shaw's Point [8MA7] and Cockroach Key [8HI2]), both parties appear to have sailed past Harbor Key without taking note. The 1874 Coast and Geodetic Survey topographical chart depicting Bishop Harbor (T-sheet #1346B; Supplemental Figure 2) shows that the site was shrouded in dense mangrove forest (much as it remains today), such that the mounds were probably not visible from the navigable waters of the open bay.
Correspondence dated 1920–1921 between local collector Charles Theodore Earle Jr. and Jesse W. Fewkes of the Bureau of American Ethnology (BAE) contains the earliest known sketch map of Harbor Key (Supplemental Text 1). Earle's letters report digging into the ancestral burial mound and the removal of the southern quarter of the large platform mound during a short-lived shell-mining operation (Supplemental Figure 3). Correspondence from 1934 reveals that the prolific antiquarian Montague Tallant visited Harbor Key in the spring of that year, oversaw the excavation of a 4.5 × 6 m (15 × 20 ft.) trench into the burial mound, and reported his findings to Matthew Stirling, then director of the Smithsonian Institution (Supplemental Text 2). Ultimately, although brief reports of contact period funerary objects piqued the interest of Fewkes and Stirling, local collectors failed to inspire BAE or Smithsonian investigations, which instead focused on more accessible Tampa Bay mound sites at Weedon Island (8PI1) and Safety Harbor (8PI2): those sites would become widely known for assemblages of ornate mortuary ceramics and be considered as type-sites for regional archaeological cultures (Willey Reference Willey1949).
Ripley Bullen visited Harbor Key in 1952 after collectors disturbed ancestor burials at the base of the platform mound ramp. Bullen toured the site's central complex, made a small collection of artifacts, and proposed an age in the late precolumbian/Safety Harbor period (around AD 1000–1500), based solely on coetaneous typologies for platform mounds and mound-plaza complexes. The ceramic assemblage Bullen analyzed contained nondiagnostic check-stamped and plain sand-tempered sherds (Bullen et al. Reference Bullen, Reeder, Bell and Whisenant1952:22). Burger (Reference Burger1979) produced the first topographic map of the site (Figure 2). Comparison with our 2021 lidar-derived topobathymetric map underscores the accuracy of Burger's work. After clearing dense brush, Burger determined that the short landward shell ridge described vaguely by Earle comprises a low, flat-topped mound. Burger speculated that this mound was constructed in the form of a bird, soaring westward. Although it is difficult to evaluate this interpretation of the mound form and orientation, especially given the rarity of effigy mounds in the region (but see Walker Reference Walker1880:402–404), the form and cardinal orientation of the mound are prominent; in the absence of published mound names or codes, we adopt Burger's nomenclature in referring to this feature as the Bird Mound. In 1995, Burger's research and longitudinal advocacy at Harbor Key led to the protection of the site and numerous others within the Terra Ceia Preserve State Park—a 2,000-acre estuarine preserve along Tampa Bay's southeastern shoreline.

Figure 2. Comparison of topographic maps for Harbor Key mound complex: (a) topographic map of Harbor Key by Burger (1979); (b) 2021 aerial lidar-based digital elevation model of Harbor Key, 50 cm contour interval. (Color online)
Most recently, Wheeler (Reference Wheeler2002) visited Harbor Key with Burger to document and backfill a 2 m deep vandal pit dug into the platform mound summit, exposing a complex stratigraphy of secondary shell fill, thin primary midden layers, and ash lenses. The team collected four carbon samples from strata representing probable mound-summit activity surfaces and were surprised by the Middle Woodland dates (about 200 BC–AD 400), which they found “much too old for the presumably Safety Harbor [i.e., Mississippian] mound.” The team attributed these dates to potential contamination from leached humic materials or their mistaking of secondary mound fill as in situ top-soil horizons (Wheeler Reference Wheeler2002:5).
Methods
Field Methods
Fieldwork involved sediment coring, test-unit excavations, and auger probing (Figure 3). To investigate Holocene paleoenvironmental history in Bishop Harbor, we collected six vibracores along a cross-shore transect running from the seaward edge of the tidal shelf to the landward head of the sub-basin. Vibracoring protocol followed standard methods (Lanesky et al. Reference Lanesky, Logan, Brown and Hine1979) using 7.6 cm diameter aluminum pipes. At Harbor Key, we excavated six 50 × 50 cm test units: four along the shoreline ridge (STPs 1–4), one atop the platform mound summit (STP7), and one into the center of the Bird Mound (STP8). Excavations proceeded in 10 cm levels within observed strata until reaching the tidal water level. STPs 1, 3, and 4 were extended by auger probing (7.6 cm diameter) in 20 cm levels to a maximum of 1.5 m depth, enabling us to document stratigraphy and collect samples below the limit of open excavation. Attempted auger probing into unit floors at STPs 2, 7, and 8 was blocked by large mollusk shells. We processed the excavated sediments through a 3.2 mm (0.125 in.) screen and collected all observed artifacts and vertebrate faunal remains. Mollusk shell was rough sorted in the field. Due to the large volume of oyster (Crassostrea virginica) shell, we counted, weighed, and sampled left (i.e., bottom) oyster valves bearing a hinge, whereas oyster fragments and right valves were weighed in aggregate. This strategy enabled us to produce accurate taxonomic composition data while also leaving material for backfilling. Small bulk-sediment samples were collected from recognized strata within excavation units for sedimentological analyses.

Figure 3. Maps of subsurface sampling across Bishop Harbor and Harbor Key: (a) vibracore locations; (b) sampling locations at Harbor Key. (Color online)
We collected three percussion cores at Harbor Key using a standard single-section method and 7.6 cm diameter aluminum pipe: one core was in a fringing mangrove swamp seaward of the shoreline ridge (PC1), another was in a mangrove swamp near the center of the plaza (PC2), and a third was in an oblong area of raised shell midden that defines the northern extent of the plaza (PC3). We collected two additional percussion cores from the platform mound summit using a multisection JMC subsurface probe, which enabled us to sample to a maximum depth of 4.5 m. A systematic 20 m grid of auger probes was excavated across the plaza area. Auger probes measured 7.6 cm in diameter and were excavated in 20 cm levels to a maximum depth of 1.5 m. Auger-excavated sediments were processed through 3.2 mm (0.125 in.) mesh, and all captured materials were collected for laboratory sorting and analyses. Surface elevation data for cores, auger probes, and excavation units were corrected to NAVD88; tidal frame elevations were derived from the NOAA datum at Port Manatee (station 8726384).
Laboratory Analyses
After splitting, core samples were described using qualitative assessments of sediment texture, Munsell color, and macrofossil content. Macrobotanical inclusions and organic sediments suitable for AMS-radiocarbon dating were collected; subsequently, cores were subsampled stratigraphically for granulometric, loss-on-ignition, and macrofossil analyses.
Sediment samples from cores and shovel tests were analyzed to produce grain-size distributions for stratigraphic interpretation. Subsamples were dispersed into a sodium hexametaphosphate solution (50 g/L) and agitated regularly over a 12-hour soaking period. After soaking, the samples were wet-sieved through 63 micron (4 phi) mesh, separating coarse and fine fractions. Coarse fractions were dried and processed through standard sieves to yield percent-composition values for sand-sized particles at 0.25 phi intervals. Mean grain size and sorting statistics were calculated by the moment method (i.e., weighted averaging) following Balsillie (Reference Balsillie1995).
Total organic matter (TOM) and carbonate content (CaCO3) were derived by sequential loss-on-ignition following established methods (Dean Reference Dean1974). Crucibles were prepared, weighed, and loaded with 1.5 cm3 of sediment before being weighed again and dried at 60oC for 48 hours. After measuring dry sediment weights, we combusted the samples in a muffle furnace for five hours at 550oC and measured the loss of mass, yielding TOM values. The samples were then combusted again for five hours at 900oC and weighed once more to yield CaCO3 values.
Analyses of mollusk shell assemblages followed established protocols (Reitz and Wing Reference Reitz and Wing2008) and identified all collected shell (>3.2 mm) to the lowest possible taxonomic unit. Intact and fragmentary shells were analyzed to estimate the minimum number of individuals (MNI) for each taxon within an assemblage through the identification of nonrepeating shell features (Mason et al. Reference Mason, Peterson and Tiffany1998:307). Because oyster fragments without hinge elements (i.e., not contributing to MNI) were weighed and discarded in the field, we cannot derive reliable estimates of number of identified specimens (NISP) for oysters.
A total of 35 plant-macrofossil and organic-sediment samples were analyzed at the Center for Applied Isotope Studies at the University of Georgia to produce AMS radiocarbon assays. We calibrated raw 14C dates in OxCal software version 4.4.4 and used IntCal20 to derive probability distributions for calendar ages (Reimer et al. Reference Reimer, Austin, Bard, Bayliss, Blackwell, Ramsey and Butzin2020).
To correlate our work with past sea-level dynamics, we used two well-resolved sea-level reconstructions developed from salt-marsh sediment deposits on Florida's Atlantic and Gulf Coasts: a study from the northeast Florida coast by Hawkes and colleagues (Reference Hawkes, Kemp, Donnelly, Horton, Richard Peltier, Cahill and Hill2016) that modeled sea-level rise across 25 conservative index points (i.e., basal marsh peats) spanning about 8000–2000 cal BP and a study from Tampa Bay by Gerlach and coworkers (Reference Gerlach, Engelhart, Kemp, Moyer, Smoak, Bernhardt and Cahill2017) that analyzed botanical and foraminifera datasets to track salt-marsh accretion and model sea-level rise across the common era.
Results
Our program of sampling and analyses enabled us to set the Harbor Key site within a seascape-scale stratigraphic framework, establish chronostratigraphy of the mound complex, and reconstruct site-formation processes. Figure 4 displays a cross-sectional stratigraphic diagram of the major sampling transect across Bishop Harbor and Harbor Key, representing depositional environments and cultural features extending from about −5 m elevation at the base of the deepest vibracore (BH-VC5) to about +5 m at the summit of the Harbor Key platform mound (e.g., STP7, MC1, MC2). Radiocarbon dates are presented in Table 1; in this section we report calibrated radiocarbon ages with corresponding 2-sigma error ranges.

Figure 4. Cross-sectional stratigraphy and interpretation of samples from Bishop Harbor and Harbor Key depicting sedimentary facies and chronostratigraphy. (Color online)
Table 1. Radiocarbon Results from Subsurface Samples Collected at Bishop Harbor and Harbor Key.

Estuarine Sediment Cores
The seaward set of vibracores (VC1, VC2, and VC3) encountered estuarine sediments overlying a sharp contact with Miocene-age limestone (Figure 5). The relatively shallow base rock in the area, coupled with the exposure of the bay's southeastern shoreline to waves from the Gulf of Mexico, has resulted in relatively poor preservation potential for Late Pleistocene and Holocene depositional environments. However, as is typical of the region, karst processes created an uneven limestone surface, and VC2 intersected a depression feature containing well-preserved organic-rich seagrass beds that accumulated between 5380 ± 79 cal BP and 4676 ± 144 cal BP. Sand-flat and shoal deposits bearing layers of shell hash overlie a sharp contact with these buried tidal wetland beds, making up the major portion of the estuarine sedimentary unit seaward of Harbor Key and representing the considerable influence of large waves on the open shoreline during normal and storm conditions. Core VC3 encountered a buried seaward section of the Harbor Key shellworks that overlies a thin paralic or terrestrial soil dated to the mid-Holocene (4467 ± 58 cal BP).

Figure 5. Core photos and diagrams of seaward vibracores (VC1, 2, and 3), with sedimentological data from VC2. (Color online)
Vibracores landward of Harbor Key (VC4, VC5, and VC6) also intersected an uneven limestone surface, overlain by unconsolidated shelly muddy sand beds that represent the reworking of Pleistocene shell deposits within deglacial / Early Holocene fluvial environments (Figure 6). Fluvial marsh sediments accumulated atop the more consolidated shelly substrates at VC4 and VC5 during the mid-Holocene (4672 ± 144 cal BP). Subsequently, after 2242 ± 89 cal BP, oyster bioherms became established at these locations and accreted approximately 1 m of reef before conversion to the modern sand-flat environment (likely around 1000 cal BP). The estuarine unit encountered in VC6, at the head of Bishop Harbor, lacks preserved wetland beds and attests to the relatively recent reworking of unconsolidated sediments by wave action and bioturbation in open areas of the bayou.

Figure 6. Core photos and diagrams of landward vibracores (VC4, 5, and 6), with sedimentological data from VC5. (Color online)
Harbor Key Mound Complex (8MA15)
Figure 7 displays the cross-sectional stratigraphy of the Harbor Key site corrected to NAVD88 and set against past and present sea-level stands following Hawkes and colleagues (Reference Hawkes, Kemp, Donnelly, Horton, Richard Peltier, Cahill and Hill2016) and Gerlach and coworkers (Reference Gerlach, Engelhart, Kemp, Moyer, Smoak, Bernhardt and Cahill2017). Cultural shell-bearing deposits extend from about −2.5 m (VC3) to +5.5 m elevation (STP7, MC1, MC2), with approximately 3 m of the site situated below the present mean sea level (MSL)—indicating a deep history of interaction between shellwork formation and coastal dynamics. The buried cultural shell stratum encountered in VC3 and the preserved mid-Holocene estuarine deposits recovered in VC2 suggest that the settlement at Harbor Key once extended between 100 and 200 m seaward from the present shoreline. In the following subsections we draw on sedimentological, mollusk-zooarchaeological, radiometric, and artifact data to describe site features and reconstruct site-formation processes at Harbor Key.

Figure 7. Cross-sectional stratigraphy of subsurface samples from Harbor Key. (Color online)
Harbor Key Platform Mound
The most conspicuous cultural feature at Harbor Key is the approximately 5.5 m tall pyramidal platform mound. The stratigraphy (STP7, MC1, and MC2) and sedimentological data (MC2) from the platform mound reveal numerous layers rich in fine organic sediments consistent with primary middens, buried-A (Ab) horizons, and fire features alternating with larger packages of secondary shell-rich mound fill (Figure 8). STP7 encountered dense deposits of whole and fragmentary shell (538 kg/m3) in a dark-gray sand matrix. The unit produced an assemblage of 7,042 individual mollusks dominated by oyster (63.8%) but also containing an appreciable percentage (21.1%) of large gastropods (principally Melongena corona, 15.4%) and an unusual abundance (6.9%) of Balanus sp. (Table 2). A thin layer of compact charcoal-rich clayey sand with relatively few shells (level 9, 82–85 cmbs) in STP7 yielded an atypical abundance (31.7%) of soil-dwelling terrestrial snails (Polygyra sp.) and likely represents a previous platform mound stage. Ceramics were recovered in moderate concentration (750 g/m3) and included smooth and gritty varieties of plain sand-tempered ware. Vertebrate faunal remains were found in greater concentration in STP7 (about 2 kg/m3) than in other site features. Seventeen AMS radiocarbon dates from the upper 4.5 m of the platform mound show several inversions characteristic of mound building with mined secondary fills; however, internal consistency among the dates constrains the construction interval, conservatively, to the first six centuries AD and most likely between about AD 200 and 600. Notably, our work produced a complete absence of Safety Harbor period dates and justifies the reclassification of Harbor Key platform mound as Middle Woodland period architecture (about AD 200–600).

Figure 8. Stratigraphic diagram of subsurface samples from Harbor Key platform mound with sedimentary data from core MC2, displaying numerous fine organic layers consistent with possible activity surfaces. (Color online)
Table 2. Mollusk-Zooarchaeological Data in MNI and Percent MNI, Aggregated by Site Feature.

* Rare mollusk taxa are those that make up less than 1% of any site feature assemblage.
Bird Mound
Excavations into the Bird Mound (STP8 and AP5) encountered dense whole and fragmentary shell (500 kg/m3) in a dark gray-to-black muddy sand matrix situated between about +1.1 to −0.1 m elevation and exhibiting a distinctive greasy texture typical of primary midden. These sediments, as well as those of adjacent areas at 8MA15 where shell midden features peek through the mangrove-forest floor (e.g., PC3), comprise 59%–71% shell, 21%–37% sand, and 4%–11% mud, with considerable (2%–9%) organic content. Excavation of STP8 produced a total mollusk assemblage of 3,396 individuals dominated by oyster (79.1%), with minor percentages of M. corona (4.2%), Strombus alatus (3.6%), and Sinistrofulgur sinistrum (3%; Table 2). Pottery was recovered in moderate concentration (1,165 g/m3) from STP8 and included gritty sand-tempered plain wares. Three radiocarbon assays from STP8 fall between cal AD 118 ± 89 and 515 ± 79, indicating that Bird Mound and the larger platform mound across the plaza may have been constructed simultaneously.
Shoreline Ridge / Coastal Berm
Excavations along the low (about 1 m tall) shore-parallel ridge were intended to document and sample in situ domestic contexts given that such shoreline midden features in the region have long been interpreted as areas of concentrated occupation (e.g., Luer and Almy Reference Luer and Almy1982). However, our testing revealed that the original morphology and distribution of midden features at Harbor Key were significantly obscured by coastal taphonomy. Within the shoreline ridge we encountered a distinctive reworked sediment unit comprising medium-fine quartz sand (27%–97%) and shell (315 kg/m3), with superimposed fining-upward sequences and buried lenses of weathered wrack (Figure 9). ANOVAs confirm that sand (0.063–2.0 mm) and medium sand (0.0125–0.5 mm) are significantly more abundant within the shoreline ridge than in adjacent mound or midden contexts (Supplemental Tables 1 and 2). Excavations into the shoreline ridge produced a total mollusk MNI of 12,992 (Table 2). The most striking pattern within the assemblage is the relative abundance of small tidal-zone gastropods (27.8%), including Cerithium sp. (17%), Prunum apicinum (9.5%), and several rarer taxa (1.8%). ANOVAs confirm that shoreline ridge sediments contain significantly higher relative frequencies of small tidal-zone snails than mound or midden contexts (Supplemental Table 3). These small gastropod shells frequently retain their natural pigments, suggesting rapid burial, whereas the remainder of the mollusk assemblage (53% C. virginica, 2.7% M. corona, and 2.1% S. sinistrum) has been sub-aerially weathered much like the cultural shell within the adjacent mound and midden features. Pottery, bone points/pins, and shell scoops/spoons occur within the shoreline ridge in a low to moderate concentration (e.g., pottery = 624 g/m3). Almost without exception, artifacts recovered from the shoreline ridge exhibit substantial rounding and polish by wave-induced abrasion (Supplemental Figure 4). Radiocarbon assays on buried charcoal inclusions from near-surface and basal levels of STP1 produced inverted dates, and charcoal inclusions recovered from buried contexts at STP2 (60–70 cmbs) and STP3 (70–80 cmbs) yielded recent-historical dates. Synthesizing these characteristics and drawing on experience monitoring post-storm effects at estuarine shellworks in the region, we interpret the shoreline ridge at Harbor Key as a coastal berm produced under storm conditions—where tidal surge and large waves entrain seaward-lying sediment beds (including cultural deposits eroding from the sand flats) and small tidal-zone gastropods (clustered within fringing mangrove forest habitats) and deposit them landward.
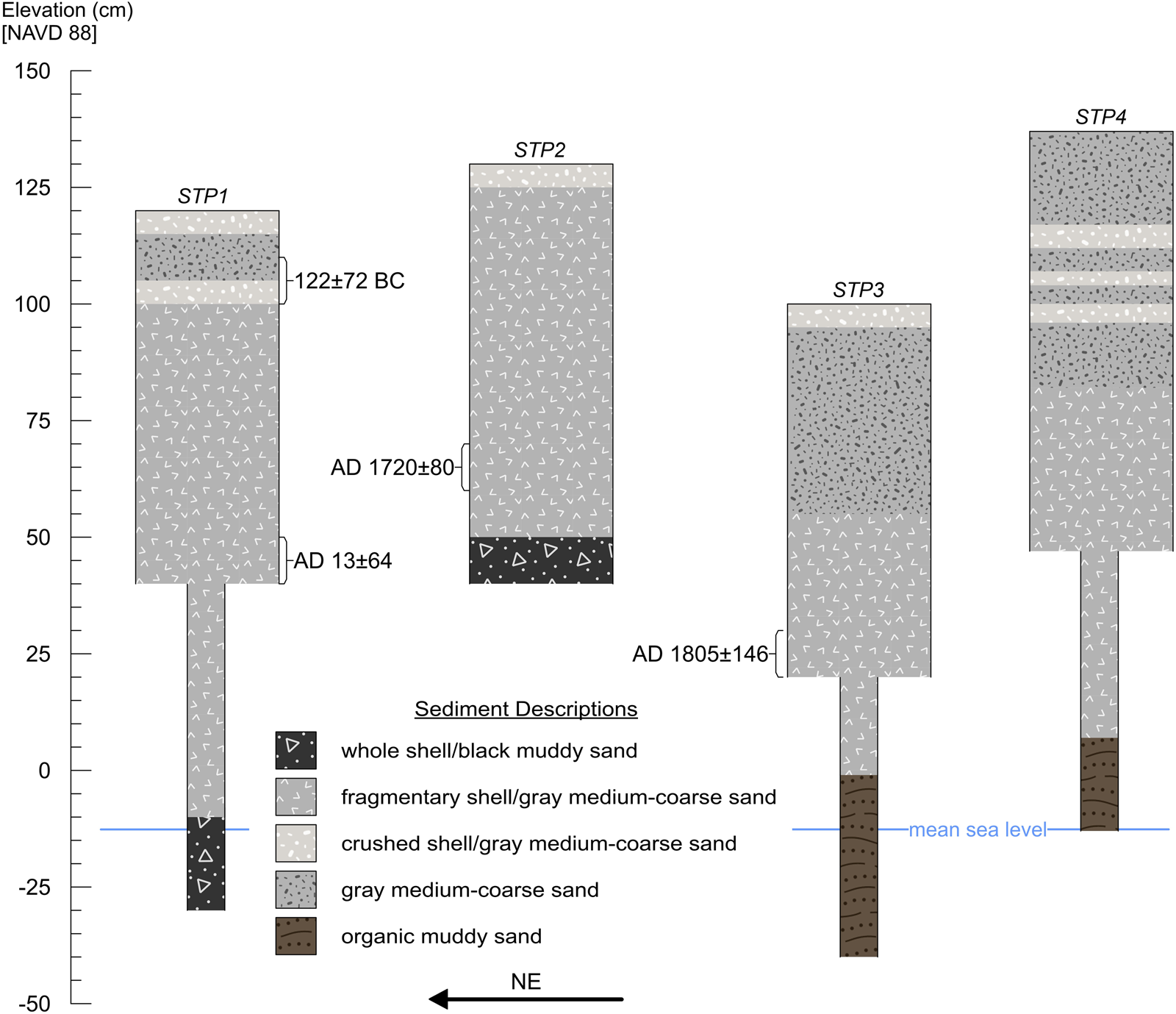
Figure 9. Stratigraphic diagram of subsurface samples from the shoreline ridge / coastal berm. (Color online)
Supra-Plaza Mangrove Peat Bed
The plaza at Harbor Key is a low-lying (i.e., intertidal) and flat area (about 9,000 m2) bounded by the site's mounded features that supports a dense forest of mature mangroves (Avicennia germinans and Rhizophora mangle). Our survey of the area consistently located the uppermost surface of the former plaza beneath about 0.5 m of mangrove peat. Basal peat samples collected near the contact with the buried plaza surface in PC1 and PC2 (about −0.55 m elevation) yielded radiocarbon ages (cal AD 1557 ± 78 and cal AD 1661 ± 134, respectively) consistent with the expected incipient tidal inundation of the ancient plaza following the sea-level curve by Gerlach and colleagues (Reference Gerlach, Engelhart, Kemp, Moyer, Smoak, Bernhardt and Cahill2017).
Submound Shell Platform
Extending beneath the plaza surface (PC2, AP2-4, AP6-17) we encountered a shell-rich (310 kg/m3) sedimentary unit exhibiting striking similarity to the shoreline ridge/coastal berm. Importantly, we also documented this same reworked sediment deposit beneath the base of the platform mound (AP1), the base of the Bird Mound (AP5), raised midden areas (PC3, PC15), and the modern tidal flat (VC3). Our mapping and analyses of this sediment deposit suggest that the sub-aerial (currently inter- and supratidal) features of the Harbor Key mound complex were built atop a previously undocumented platform of sand and reworked cultural shell. Within these buried (currently subtidal) deposits, total sand (45%–82%) and medium sand (17%–40%) are conspicuously abundant, and the samples group with coastal berm sediments in ANOVA-based comparisons of site features (Supplemental Tables 1 and 2). Submound platform sediments also exhibit the superimposed fining-upward sequences observed in the coastal berm, consistent with deposition during high-energy storm conditions (Figure 10). Cores and auger probes from the submound platform produced a total mollusk MNI of 984 (Table 2). As within the shoreline ridge assemblage, these deposits contain a conspicuously high relative abundance of small tidal-zone gastropods (42%), particularly Cerithium sp. shells (38%) with preserved pigments (suggesting rapid burial). The ANOVA-based comparison of small tidal-zone gastropod abundance among site features grouped the submound platform samples with those from the coastal berm and showed significant differences from mound and midden sediments (Supplemental Table 3). Submound platform deposits also contain ceramics and vertebrate faunal material throughout (ubiquity = 0.82), and all recovered pottery sherds exhibit rounding and polish consistent with wave-induced abrasion (Supplemental Figure 5). Charcoal inclusions recovered from submound platform deposits in PC2 and PC3 yielded dates (cal 242 ± 122 BC and 87 ± 87 BC, respectively) that precede the construction of the Bird Mound and platform mound (Figure 10). Given the recovery of predominantly modern/recent-historical charcoal inclusions from the extant coastal berm/shoreline ridge feature, these late BC dates from the buried reworked deposits may best indicate the interval of disturbance and redeposition. Although the submound platform deposits extended below the maximum sampling depth in the plaza area and beneath mounds (about 1.5 m), core VC3 intersected this sedimentary unit and located a basal contact at about a −2.5 m elevation atop a paralic soil dated 4467 ± 58 cal BP—suggesting a thickness of approximately 2 m and providing a tentative terminus post quem for the early shellwork deposition. Much like the extant coastal berm feature forming the shore-parallel ridge, our testing suggests that the buried shell-rich sedimentary unit underlying the mound center is composed of antecedent cultural shell deposits (about 2450–1 BC) that were reworked by ancient storms. Given the level upper surface of this feature, we presume that Native people recontoured and otherwise artificially prepared the submound shell platform to provide a raised foundation for the Middle Woodland mound complex.

Figure 10. Core photos and diagrams of subsurface samples from lower-elevation areas at Harbor Key (PC1, 2, and 3) with granulometric data depicting superimposed fining-upward sequences (high-energy event bedding) in the submound shell platform feature. (Color online)
Discussion
Sedimentary records preserved within the Bishop Harbor sub-basin reveal that productive and relatively low-energy estuarine habitats (for example, seagrass beds and coastal marsh) were established both landward and seaward of Harbor Key by the mid-Holocene—contemporaneous with the broader expansion of paralic wetlands within central peninsular Gulf Coast estuaries. New stratigraphic data from buried—now subtidal—contexts at Harbor Key attest to Native occupation during the Mid- to Late Holocene (likely 2450–1 BC), which is consistent with the recovery of fiber-tempered ceramics from seaward tidal flats (Burger 1979). Major transformations of the inshore estuarine zone by about AD 200 into more open, wave-dominated environments brought on by sea-level rise and increased hurricane landfall resulted in the erosion and reworking of early shell-bearing components at Harbor Key—producing coastal berm-like features composed of quartz sand, ex situ cultural mollusk shell, taphonomic mollusk-inclusions (small tidal-wetland dwelling gastropods), and wave-abraded artifacts. With subsequent sea-level rise and continued terraforming by Indigenous people, these features came to make up the major topographic barrier protecting Bishop Harbor's shallow estuarine habitats from the energetic conditions of the open bay and swells from the nearby Gulf of Mexico during storms. As marine transgression transformed Bishop Harbor from a restricted tidal creek system into a shallow open-water basin, Indigenous shellworks constrained the mouth of the drainage to form a relatively deep and narrow (and thus, stable) tidal inlet. While the transition to open estuarine conditions generated significant erosional forcing in exposed areas of the sub-basin, Indigenous shellworks at Harbor Key provided protection for landward inshore zones where oyster reefs and seagrass meadows established and proliferated.
Radiocarbon dates on the sub-aerial shell mound architecture at Harbor Key indicate that Native peoples reinitiated mound building by about AD 200 and continued using the ceremonial center until around AD 600. These data falsify at least 70 years of faulty interpretation based on the uncritical typological association of flat-topped pyramidal platform mounds (or “temple mounds”) with the Safety Harbor period (after AD 1000) and Native societies described in early European accounts from the region (Bullen Reference Bullen1955; Luer and Almy Reference Luer and Almy1981). Our chronological revision at Harbor Key parallels a larger trend in southeastern archaeology toward recognizing the early emergence of platform mound ceremonialism (Kassabaum Reference Kassabaum2021; Thompson et al. Reference Thompson, Holland-Lulewicz, Butler, Hunt, Wendt, Wettstaed and Williams2022) and early monumental architecture in general (e.g., Saunders et al. Reference Saunders, Mandel, Garth Sampson, Allen, Thurman Allen, Bush and Feathers2005; Schwadron 2017). The temporality, monumental scale, arrangement, and elaborate morphology of the shellwork complex place Harbor Key among relatively few early civic-ceremonial centers documented on the Florida peninsula where relatively dense populations aggregated around ritually charged locations bearing evidence of ancestral—Late Archaic to Early Woodland—land use (Lawres and Colvin Reference Lawres and Colvin2021; Pluckhahn and Thompson Reference Pluckhahn and Thompson2018; Sassaman et al. Reference Sassaman, Blessing, Goodwin, Jenkins, Mahar, Boucher, Barbour and Donop2020; Wallis and McFadden Reference Wallis and McFadden2019; Wallis et al. Reference Wallis, LeFebvre, Blessing and McFadden2022). Previously studied early civic-ceremonial centers in the region are concentrated along Florida's northern Gulf Coast, with conspicuous inland peninsular examples (e.g., 8GL23, 8PB6292) set within the Lake Okeechobee basin (Figure 1). The present study at Harbor Key, along with recent work at Cockroach Key (8HI2; Pluckhahn et al. Reference Pluckhahn, Jackson and Rogers2022b) and Bayshore Homes (8PI41; Austin and Mitchem Reference Austin and Mitchem2014), indicates that mound centers on the central peninsular Gulf Coast may have served as important nodes of interaction and exchange within ritual and political economies that connected Native peoples from the southern peninsula to the greater southeastern coastal plain and farther afield (Pluckhahn et al. Reference Pluckhahn, Wallis and Thompson2020). Our findings call for further investigation of understudied mound centers on the central and southern Florida Gulf Coast, particularly sites at the mouths of the Anclote (e.g., 8PA10) and Pithlachascotee Rivers (e.g., 8PA2), as well as shell mounds within Charlotte Harbor, Pine Island Sound, and Estero Bay.
Despite compelling temporal and architectural similarities, Harbor Key demonstrates previously unrecognized variation in the stratigraphy and formation histories of early civic-ceremonial centers. At Spring Warrior (8TA154), Garden Patch (8DI4), Shell Mound (8LV42), and Crystal River (8CI1), common era mound complexes were built atop quartz-sand substrates associated with relic marine terraces or remnants of terminal Pleistocene parabolic dune ridges where ancestral peoples had developed mortuaries. In contrast, Native peoples constructed the civic-ceremonial complex at Harbor Key atop a large platform of storm deposits comprising largely ex situ ancestral shellworks. At a seascape scale, the Late Holocene geoarchaeological record from Bishop Harbor suggests that morphodynamic interactions between Indigenous shell terraforming, storm landfalls, and sea-level rise conditioned estuarine development in the leeward bayou by limiting fetch, attenuating waves, and detaining terrestrial freshwater input—all of which likely aided the establishment and persistence of inshore tidal wetland systems, such as oyster reefs and sea-grass meadows. Research on modern artificial reefs (e.g., Donadi et al. Reference Donadi, van der Heide, van der Zee, Eklof, van de Koppel, Weerman, Piersma, Olff and Eriksson2013; Hancock and zu Ermgassen Reference Hancock, Ermgassen, Smaal, Ferreira, Grant, Petersen and Strand2019) and First Nations’ terraformed estuarine terrace-gardens (e.g., Augustine and Dearden Reference Augustine and Dearden2014; Grosbeck et al. Reference Groesbeck, Rowell, Lepofsky and Salomon2014) demonstrates that anthropogenic coastal-estuarine barriers can modify habitats and augment ecological production at spatially extended scales; that is, tens to hundreds of meters leeward. Along the Northwest Coast, such features came to play important roles within Indigenous systems of proprietorship, sea tenure, and resource redistribution (Deur and Turner Reference Deur and Turner2005; Grier et al. Reference Grier, Angelbeck and McLay2017). We propose that, by altering the character and distribution of leeward estuarine habitats, Native shell-terraforming at Harbor Key coproduced spaces in which subsequent social relations took/take place and in which relationships between humans and nonhuman biota were/are negotiated. Renewed/sustained civic-ceremonial aggregation and mound building at Harbor Key during the early common era indicates that Native peoples recognized and took advantage of the morphodynamic effects produced by reworked ancestral sites and the associated augmentation of inshore estuarine production. Indeed, Middle Woodland-period ceremonial provisioning—feasting—at Harbor Key almost certainly involved harvesting leeward resources supported by centuries to millennia of ancestral land use and terraforming.
Despite the apparent ecological suitability of the seascape for continued intensive settlement, as observed at civic-ceremonial centers along the Northern Gulf Coast, archaeological evidence suggests the decline of depositional activities—habitation, mound building, and the like—at Harbor Key after around AD 600, as well as apparently scrupulous avoidance by descendant Safety Harbor period peoples. However, there is compelling and unambiguous ethnohistoric evidence from the sixteenth century that Native peoples in Lower Tampa Bay used large artificial estuarine enclosures—much like the artificially enclosed Harbor Key / Bishop Harbor sub-basin—to facilitate the mass capture of fishes and other fauna (see Pluckhahn et al. Reference Pluckhahn, Jackson and Rogers2022a), suggesting that ancestral terraformed sites like Harbor Key continue to function as durable coastal infrastructures.
Conclusions
In this study, we apply a coastal-geoarchaeological approach to the shell-terraformed Indigenous mound complex at Harbor Key and the Holocene sedimentary record preserved within Bishop Harbor, an inshore sub-basin of Tampa Bay on the central peninsular Gulf Coast of Florida. Synthesizing sedimentological, mollusk-zooarchaeological, and chronostratigraphic data, we set the Harbor Key shellworks into a seascape-scale geologic and paleoenvironmental framework, significantly revising the chronological and stratigraphic understanding of the site and reconstructing a complex history of interaction between coastal processes—sea-level rise, hurricane landfalls, and wave forcing—and Native American land use. These interactions played out through the erosional reworking of early (Late Archaic to Early Woodland, about 2450–1 BC) shell-bearing sites by ancient storms. The resulting landform provided a supratidal barrier that protected Bishop Harbor from energetic conditions and produced recursive biophysical effects in the leeward bayou that were favorable for the establishment and proliferation of inshore oyster reefs and other tidal wetland habitats. Subsequently, across the first half-millennium AD, Native peoples took advantage of these conditions to provision civic-ceremonial aggregations and construct a shell mound complex at Harbor Key atop a large platform composed of antecedent storm-reworked cultural deposits. We contend that the construction of the early common era mound center at Harbor Key represents monumental placemaking that materializes the recognition and reuse of ancestral shellworks, as well as the persistent management of the encompassing seascape.
Acknowledgments
We dedicate this article to the memory of archaeologist B. W. Burger (1951–2022) in recognition of his lifelong devotion to the preservation and study of Tampa Bay's natural and historical resources. We also thank Jeff Moates, Libby Royer, Chandler Burchfield, and Morrison Hatlen for invaluable assistance with field and laboratory work. Finally, we thank two anonymous reviewers for insightful comments that improved the quality of this article. Sampling permits were granted by the Florida Department of Environmental Protection (permit 10301914) and the Florida Division of Historical Resources (permit 1920.045).
Funding Statement
Funding was provided by the National Science Foundation (awards 2024397 and 1821963), the Alliance for Weedon Island Archaeological Research and Education, and the PaleoWest Foundation.
Data Availability Statement
The data used in this study are available online at tdar.org on request from the corresponding author.
Competing Interests
The authors declare none.
Supplemental Material
For supplemental material accompanying this article, visit https://doi.org/10.1017/aaq.2023.45.
Supplemental Figure 1. 2021 Aerial lidar derived topobathymetric model of Bishop Harbor, Lower Tampa Bay.
Supplemental Figure 2. 1874 US Coast and Geodetic Survey Topographic Chart No. 1346B depicting Bishop Harbor, Lower Tampa Bay.
Supplemental Figure 3. 2021 Aerial lidar derived topographic model of Harbor Key Platform Mound, 50 cm contour intervals.
Supplemental Figure 4. Wave-abraded artifacts from the shoreline ridge/coastal berm.
Supplemental Figure 5. Wave-abraded artifacts from the submound shell platform.
Supplemental Text 1. 1920–1921 correspondence between C. T. Earle Jr. and Jesse W. Fewkes.
Supplemental Text 2. 1934 correspondence between Montague Tallant and Matthew Stirling.
Supplemental Table 1. Results of ANOVA Comparing Percent Sand (0.063–2.0 mm) by Site Feature.
Supplemental Table 2. Results of ANOVA Comparing Percent Medium Sand (0.125–0.25 mm) by Site Feature.
Supplemental Table 3. Results of ANOVA Comparing Percent MNI for Small Tidal-Zone Gastropods by Site Feature.