References
Published online by Cambridge University Press: 18 September 2020
Summary
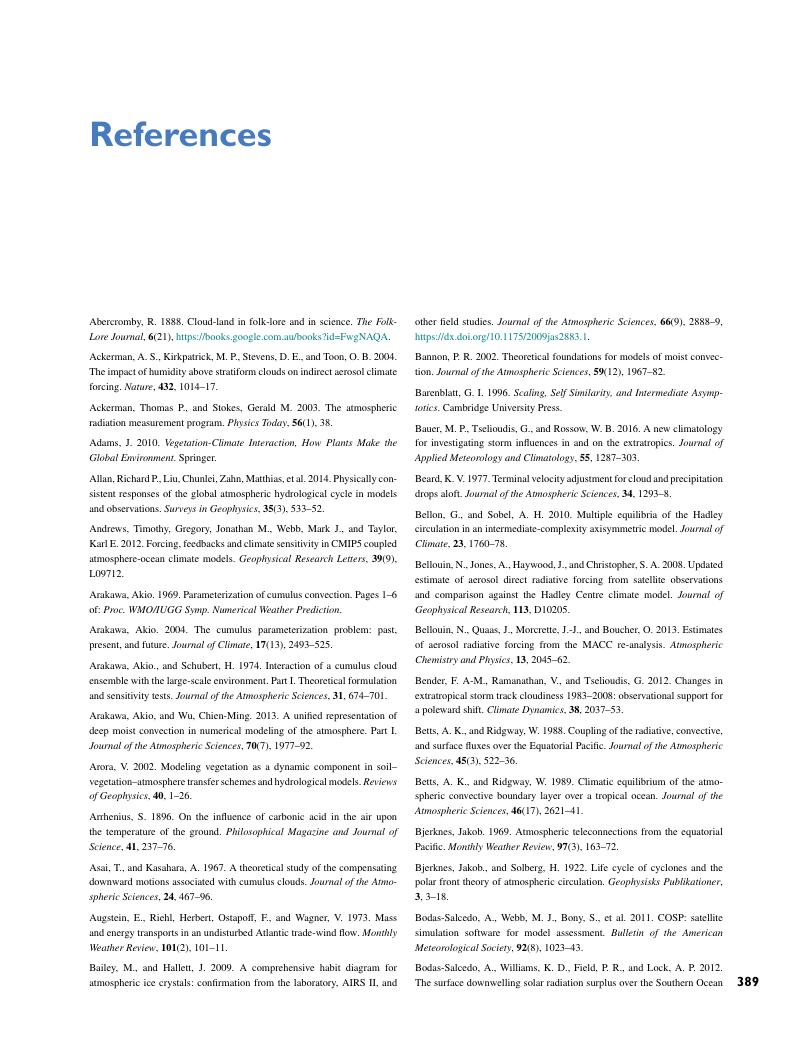
- Type
- Chapter
- Information
- Clouds and ClimateClimate Science's Greatest Challenge, pp. 389 - 400Publisher: Cambridge University PressPrint publication year: 2020