Introduction
Diabetes mellitus (DM) is one of the most common metabolic disorders, a high-incident metabolic disorder of the endocrine system, described by elevated blood sugar due to insulin deficiency or insulin resistance (Nolan and Prentki, Reference Nolan and Prentki2019). Some organ dysfunction such as nephropathy, neuropathy, retinopathy, and also vascular diseases are closely related to chronic diabetes (Inzucchi, Reference Inzucchi2013). It has been shown that long-term uncontrolled hyperglycaemia in diabetic patients can damage the hypothalamic–pituitary–gonadal axis that, in turn, contributes to fertility impairment (He et al., Reference He, Yin, Li, Zeng and Duan2021). In this regard, insulin deficiency and resistance have detrimental effects on the male reproductive system, including seminiferous tubule injury and hypogonadism (Pivonello et al., Reference Pivonello, Menafra, Riccio, Garifalos, Mazzella, De Angelis and Colao2019). In addition, it has been reported that testosterone levels, sperm production, sperm count, sperm motility, as well as semen volume were decreased in diabetic men compared with healthy individuals that could reduce the pregnancy rate in their partners (Maresch et al., Reference Maresch, Stute, Ludlow, Hammes, de Kretser, Hedger and Linn2017, Reference Maresch, Stute, Alves, Oliveira, de Kretser and Linn2018; Condorelli et al., Reference Condorelli, La Vignera, Mongioì, Alamo and Calogero2018). In fact, sexual dysfunction is considered to be a common secondary complication shown in humans and animals with type 1 or type 2 diabetes (Ding et al., Reference Ding, Liu, Liu, Pan, Guo, Sheng and Huang2015; Oridupa et al., Reference Oridupa, Folasire, Owolabi and Aina2017). Recently, it has been understood that pancreatic-produced insulin plays a crucial role in male fertility by regulating the hypothalamic–pituitary–gonadal axis, together with the adjustment of the pituitary gland sensibility to the hypothalamic gonadotropin-releasing hormone (GnRH; Schoeller et al., Reference Schoeller, Albanna, Frolova and Moley2012). From this point of view, numerous prospective studies have expressed an inverse correlation between serum testosterone and insulin levels in diabetic men (Gopal et al., Reference Gopal, Bothra, Acharya, Ganesh, Bandgar, Menon and Shah2010; Al Hayek et al., Reference Al Hayek, Khader, Jafal, Khawaja, Robert and Ajlouni2013). Therefore, dysregulation of the hypothalamic–pituitary–gonadal axis would attenuate testosterone levels, which are known as a reproductive capability indication (Chan et al., Reference Chan, Chan, Inouye, Vranic and Matthews2001).
Applied drugs for the treatment of diabetic patients show detrimental side effects and high prices. Thereby, due to less toxicity and their antioxidant properties, herbal medicines have been taken into more consideration (Shukia et al., Reference Shukia, Sharma, Puri, Prabhu and Murthy2000). Stevia rebaudiana is a natural plant that has been known for its glycoside-containing sweet leaves (Madan et al., Reference Madan, Ahmad, Singh, Kohli, Kumar, Singh and Garg2010). Although the leaves of this herb taste quite sweet, intrinsically, they contain no calories. This unique characteristic can also be ideal for diabetic patients, as the plant does not raise their blood sugar levels (Mohd-Radzman et al., Reference Mohd-Radzman, Ismail, Adam, Jaapar and Adam2013). In addition, a high phenol proportion has been found in the extracted compounds from Stevia; proposing that these agents may contribute to the antioxidant potential of the plant (Shukla et al., Reference Shukla, Mehta, Mehta and Bajpai2012). An anti-hyperglycaemic effect of Stevia in rats has been noted by several studies in which blood sugar levels were alleviated by reducing bowel glucose uptake and glycogenolysis (Jeppesen et al., Reference Jeppesen, Gregersen, Rolfsen, Jepsen, Colombo, Agger, Xiao, Kruhøffer, Ørntoft and Hermansen2003; Ahmad and Ahmad, Reference Ahmad and Ahmad2018). In addition to these benefits, Stevia rebaudiana also improved spermatogenesis and histological parameters in diabetic rat testes (Gholizadeh et al., Reference Gholizadeh, Dastghaib, Koohpeyma, Bayat and Mokarram2019).
Considering the adverse effects of hyperglycaemia on the reproductive system. As far as we know, the current study is the first to evaluate the protective effects of the hydroalcoholic extract of Stevia rebaudiana on serum testosterone levels, sperm parameters, in vitro fertilization (IVF) rate, and subsequent in vitro embryo development in streptozotocin-induced diabetic mice.
Materials and methods
Hydroalcoholic Stevia extraction
Stevia plant was collected and confirmed by Kurdistan University’s faculty of agriculture, Kurdistan, Iran. After rinsing, the Stevia leaves were air dried in the shade at room temperature and finely ground with the aid of a grinder (MJ-176-NR-3899). The dried powder leaves of Stevia were mixed with ethanol 70% (70% ethanol and 30% distilled water v/v). Then, the mixture was kept in the laboratory for 72 h in a percolator and filtered through Whatman filter papers. Afterwards, the extract was purified, and vaporized in a high-vacuum desiccator for 24 h. Finally, the extract powder was weighed and diluted with distilled water. Before administration, all extracts were filtered through 0.22-µm pore syringe filters (Ganjiani et al., Reference Ganjiani, Ahmadi and Raayat Jahromi2020; Ranjbar et al., Reference Ranjbar, Nekooeian, Tanideh, Koohi-Hosseinabadi, Masoumi, Amanat, Azarpira and Monabati2020).
Animals
NMRI male mice (6–8-week-old, 20–25 g) were obtained from the Kurdistan University of Medical Sciences (Iran). As females are less vulnerable to this β-cell toxin, most STZ-induced diabetes mice investigations used male mice (Kolb, Reference Kolb1987). At 1 week prior to the study, mice were kept under sterile laboratory conditions (12 h light/12 h dark cycle, optimum temperature 21 ± 2°C) with free access to food and water. Study procedures were approved by the Institutional Animal Care and Use Committee (IACUC) and Ethics Committees of Kurdistan University of Medical Sciences (KUMS), Sanandaj, Iran (IR.MUK.REC.1397/350). Every endeavour was made to reduce the numbers of animals and their suffering.
Induction of diabetes
Streptozotocin (STZ) seems to be the most popular compound used to evaluate the anti-hyperglycaemic activity of leaf extracts in animal models of in vivo diabetes. A high intravenous or intraperitoneal (i.p.) dose of STZ (100–200 mg/kg) preferentially destroys pancreatic beta cells in mice, while sparing extrapancreatic cells and induces quick and long-lasting hyperglycaemia (Furman, Reference Furman2015; Kottaisamy et al., Reference Kottaisamy, Raj, Prasanth Kumar and Sankaran2021). In this study, an animal model of type 1 DM was established using a single high dose of streptozotocin (Sigma-Aldrich, Germany; Furman, Reference Furman2015). At 4 h prior to STZ treatment, the normal chow diet was removed from the cages of all groups and only access to water was permitted. To induce the diabetes model, mice were injected with an STZ (150 mg/kg body weight, i.p.) solution in citrate buffer (0.1 M, pH 4.5; Yrga Adugna et al., Reference Yrga Adugna, Mequanint Adinew, Ayalew Getahun, Endale Gurmu, Yirga Berhie, Awoke and Tessema Desta2022). The mice in the control group received an equal volume of citrate buffer (pH 4.5) by the i.p. route. To prevent initial drug-induced hypoglycaemic mortality due to massive and rapid beta cell necrosis, which leads to the release of large amounts of insulin, STZ-injected animals were given 1 ml/kg of 5% glucose solution for 24 h. At 72 h after the STZ injection, blood was taken from the mouse tail vein, and fasting blood sugar (FBS) levels were determined using a glucometer (Touch Ultra Lifescan). Mice with an FBS > 200 mg/dL were classified as diabetic animals (Kifle et al., Reference Kifle, Yesuf and Atnafie2020; Yrga Adugna et al., Reference Yrga Adugna, Mequanint Adinew, Ayalew Getahun, Endale Gurmu, Yirga Berhie, Awoke and Tessema Desta2022) and favoured for treatment with 400 mg/kg of Stevia extracts.
Study groups
In total, 30 male NMRI mice were distributed randomly into the following three groups (n = 10 for each group): (i) control group (Ctrl), which was fed the normal diet and gavaged with 200 µl distilled water every day for 6 weeks; (ii) diabetic group (DIA), which were injected intraperitoneally (i.p.) with STZ and received 200 µl distilled water every day for 6 weeks; (iii) treatment group (DIA + STV), which were i.p. injected with STZ followed by a daily oral administration of 400 mg/kg of Stevia hydroalcoholic extract dissolved in distilled water (200 µl) for 6 weeks. The appropriate dosage was chosen based on previous research that demonstrated the protective effects of Stevia in STZ-induced rodent models (Ahmad and Ahmad, Reference Ahmad and Ahmad2018; Gholizadeh et al., Reference Gholizadeh, Dastghaib, Koohpeyma, Bayat and Mokarram2019).
Testosterone assay
An enzyme-linked immunosorbent assay (ELISA) kit was purchased from the Bioassay Technology Laboratory to assess testosterone levels. First, serum was isolated by centrifuging (3 min, 3000 rpm) collected blood samples. Next, in a 96-well plate coated with antibodies, both the samples and the standards were subjected to an incubation with anti-testosterone according to the specifications provided by the manufacturer.
Sperm parameters assessment
Sperm preparation was carried out at the end of the study. For this purpose, animals were anaesthetised by i.p. injection of ketamine/xylazine (80 mg/kg and 8 mg/kg, respectively) and bilaterally removed mice cauda epididymis were incubated in Ham’s F10 medium (1 ml, 30 min), after gentle tearing was done to allow spermatozoa to swim out. Sperm were counted using a Neubauer haemocytometry slide. A drop (10 µl) of sperm suspension was placed on the Neubauer slide, and sperm concentration was calculated in five squares under a light microscope at ×40 magnification. Results were reported as 106 cells/ml. For sperm motility assessment, a volume of a 10-µl specimen was used on a preheated Neubauer slide with a coverslip. At least five microscopic fields (×40 magnification) were inspected thoroughly to describe 200 sperm. Motility was represented as a percentage of progressive (rapid and slow), nonprogressive and immotile spermatozoa. A Diff-Quik™ staining set was used to determine the sperm morphology. It was expected that normal sperm would be free of deformities in the head, neck, and tail, but abnormal sperm may have one or more of these (Abdollahi et al., Reference Abdollahi, Dehghan, Balochkhaneh, Moghadam and Mohammadi2021). At least 200 spermatozoa from each mouse were examined to evaluate sperm motility, normal morphology, and sperm count.
In vitro fertilization and embryo development
Female NMRI mice (6–8-week-old) were superovulated by an intraperitoneal (i.p.) injection of 8 IU pregnant mare serum gonadotropin (Sigma-Aldrich, Saint Louis, MO, USA), followed by receiving 7.5 IU human chorionic gonadotropin (Tehran, Iran) 48 h later. Mature oocytes (metaphase meiosis II) were obtained from oviducts 14 h after hCG injection and transferred to 100-µl human tubal fluid (HTF; Millipore) medium droplets at 37°C. For IVF, sperm were harvested from the cauda epididymides of the experimental groups and capacitated by incubating for 1–1.5 h at 37°C. A final sperm concentration (0.7–1.3 × 106 sperm/ml) was achieved by diluting the sperm in the HTF medium. The spermatozoa were added to the collected oocytes and incubated for 6 h before being washed to remove any remaining sperm. Then, oocytes were cultured in modified potassium simplex optimized medium (KSOM; Sigma-Aldrich, St. Louis, MO, USA) droplets (overnight, 37°C), and the percentages of 2-cell embryos were used to determine the fertilization rate. The embryos were then cultured at 37°C and 5% CO2 under mineral oil until they had developed into the blastocyst stage.
Statistical analysis
GraphPad Prism (Version 6.01, CA, USA) was used for statistical analysis. The findings of the current investigation were represented as mean ± standard deviation (M ± SD). One-way analysis of variance (ANOVA) followed by Tukey’s test was applied to compare the results between different groups. P-values ≤ 0.05 were regarded as significant. The significance is reported by *P < 0.05, **P < 0.01 and ***P < 0.001.
Results
Body and testis weight, blood glucose
Measurement of body weight at the end of the experiment revealed a significant (***P < 0.001) reduction (22.8 ± 2.2 g) in the diabetic group, compared with the control group (30.8 ± 1.9 g). Conversely, treatment with Stevia for 6 weeks significantly (**P < 0.01) restored (28 ± 1.82 g) final body weight, compared with the diabetic group (Figure 1a). Similarly, our results demonstrated that the testis weight of diabetic mice (0.99 ± 0.03 g) significantly (***P < 0.001) decreased, compared with controls (0.118 ± 0.04 g). However, Stevia-received mice (DIA + STV) showed a significant (**P < 0.01) increase in testis weight (0.108 ± 0.02 g), compared with diabetic mice (Figure 1b).
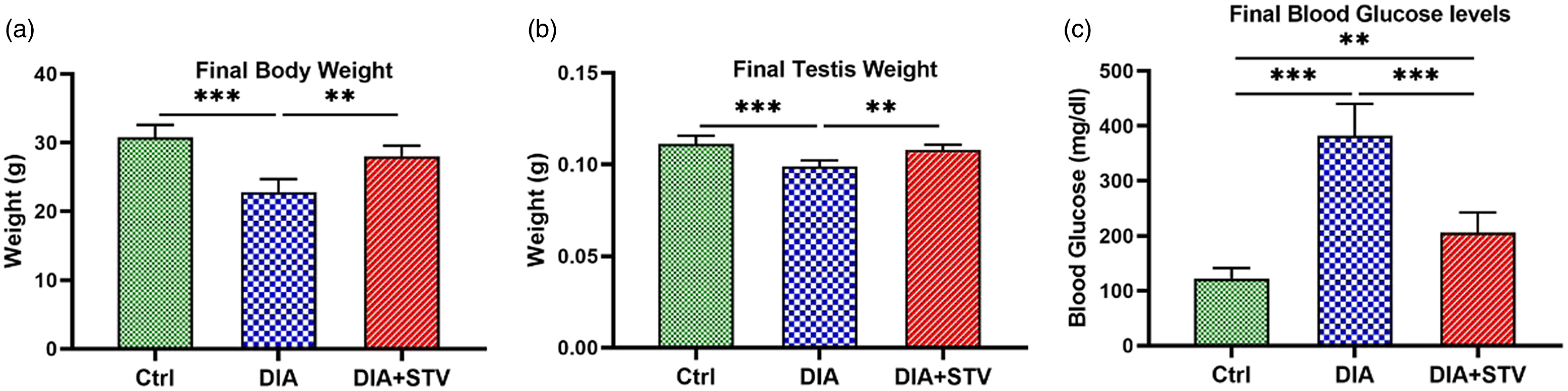
Figure 1. Effect of hydroalcoholic Stevia extracts on final body weight, final testis weight, and final blood FBS levels in different experimental groups. (a) Body weight at the end of the study. Final body weight was significantly reduced in the diabetic group relative to the controls, which was restored by Stevia treatment. (b) Testis weight at the end of the study. STZ administration significantly decreased final testis weight; however, Stevia considerably increased testis weight. (c) Blood glucose levels at the end of the experiment. Diabetes significantly induced a significant increase in the serum glucose levels, which were significantly diminished by 6 weeks of Stevia exposure. Data are shown as the mean ± standard deviation (SD). **P < 0.01, and ***P < 0.001.
A significant (***P < 0.001) elevation in FBS levels was seen in diabetic mice (382 ± 58 mg/dl), compared with control mice (122 ± 19 mg/dl). However, 6 weeks of Stevia treatment (DIA + STV) significantly (***P < 0.001) lowered FBS levels (206 ± 36 mg/dl), compared with diabetic mice (Figure 1c). Although Stevia administration reduced the mice FBS levels, there was a statistically significant difference (**P < 0.01) between treatment and control mice (Figure 1c).
Serum testosterone level
In diabetic mice (0.24 ± 0.04), blood testosterone levels were found to be significantly lower (***P < 0.001) compared with control mice (0.47 ± 0.04). When compared with diabetic mice, Stevia administration significantly (*P < 0.05) elevated serum testosterone levels in diabetic animals (0.36 ± 0.01; Figure 2).
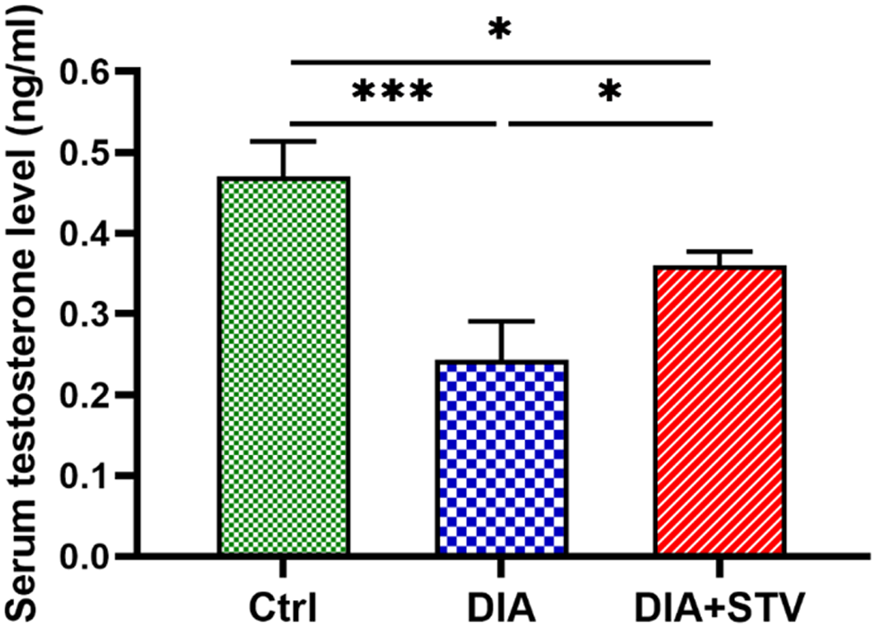
Figure 2. Assessment of serum testosterone levels by ELISA. Serum testosterone levels were significantly decreased in the diabetic group, compared with the control. Nevertheless, Stevia administration considerably raised serum testosterone levels. Significance is presented by *P < 0.05, ***P < 0.001.
Sperm parameters
Sperm count was examined to determine the effect of diabetes on spermatogenesis. Our data revealed that the number of sperms was significantly (***P < 0.001) reduced in the diabetic group, compared with the control group. Nevertheless, exposure to Stevia (DIA + STV) significantly (*P < 0.05) increased the sperm count, compared with the diabetic group (Figure 3a). The percentage of sperms with abnormal morphology was significantly (***P < 0.001) higher in the diabetes group compared with the control group. Nonetheless, when compared with the diabetic group, Stevia administration significantly (***P < 0.001) reduced the percentage of abnormal sperms (Figure 3b). Also, compared with the control group, results showed a significant (***P < 0.001) decrease in the percentage of rapid progressive sperms, but a significant (**P < 0.01) increase in the percentage of slowly progressive, nonprogressive, and immotile sperms in the diabetic group were observed. Conversely, Stevia therapy significantly (## P < 0.01) raised the percentage of rapidly progressive sperms while significantly (# P < 0.05) reducing the percentage of slowly progressive, nonprogressive, and immotile sperms compared with the diabetes group (Figure 3c).
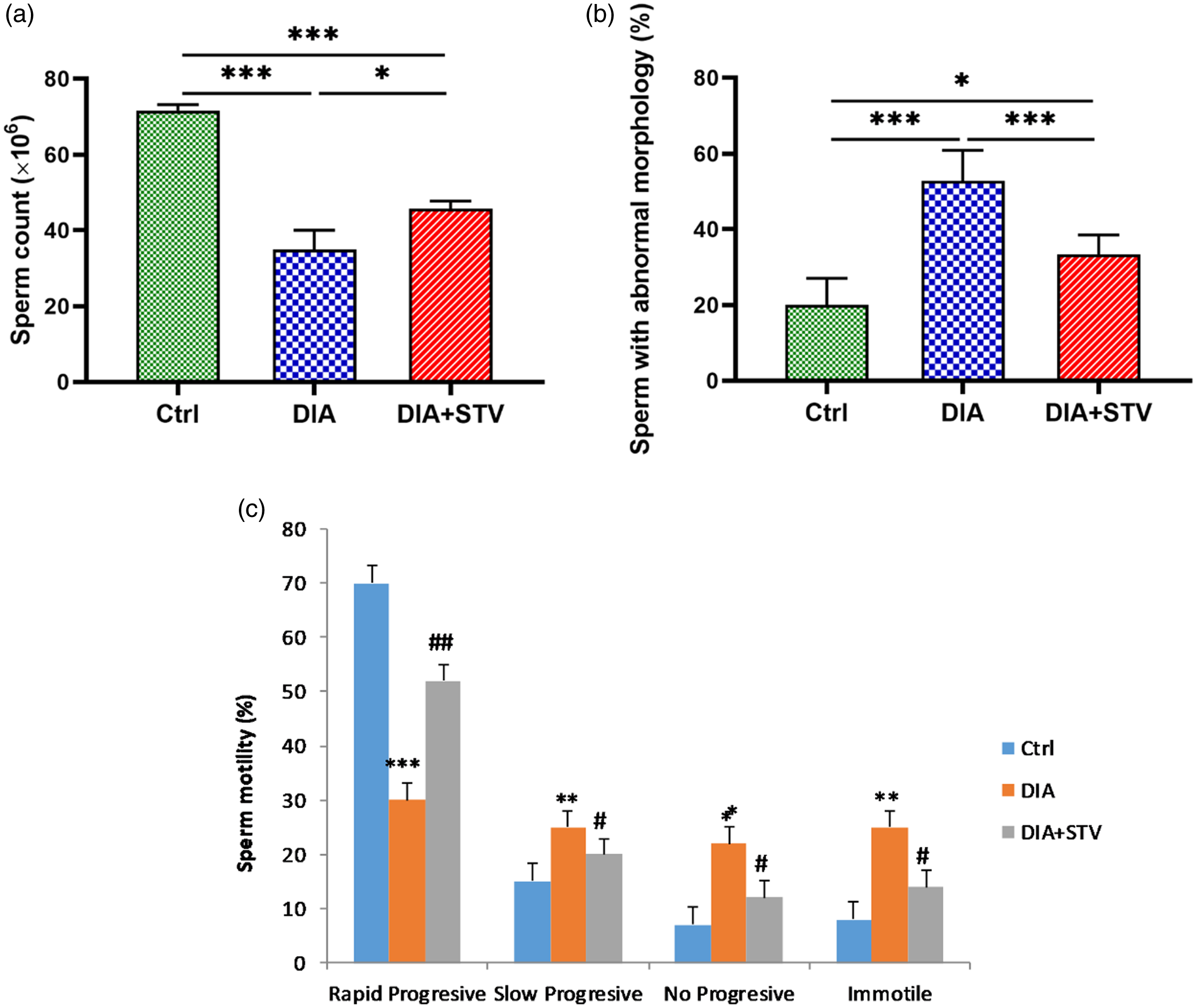
Figure 3. Comparison of sperm parameters in different experimental groups. (a) Sperm count. Diabetes caused significant decrease in sperm number, compared with the control. However, Stevia treatment considerably increased sperm count. (b) Sperm with abnormal morphology. Percentages of sperms with abnormal morphology were significantly elevated at 6 weeks, following diabetes induction. Stevia administration reduced abnormal sperms percentage. (c) Sperm motility. Sperm motility was significantly attenuated in the diabetic group relative to the control, which was promoted by Stevia treatment. Data are presented as means ± standard deviation (SD). *P < 0.05, ***P < 0.001 vs. the control group. # P < 0.05, ## P < 0.01, vs. the diabetic group.
IVF and early embryo development
The success rate of IVF was determined by counting the number of mature (MII) oocytes that developed into the 2-cell stage after fertilization (Figure 4). The results of our study (Table 1) demonstrated that the IVF success rate was significantly (***P < 0.001) reduced in the diabetic group. However, Stevia supplementation (DIA + STV) could significantly (## P < 0.01) increase 2-cell embryos, compared with the diabetic group. As shown in Table 1, the developmental rates of the 2-cell embryos to the 8-cell stage and blastocysts (Figure 4) were significantly (***P < 0.001) diminished in the diabetic group compared with the control group. Nevertheless, Stevia treatment significantly (# P < 0.05) promoted early in vitro development (IVD) success in the DIA + STV group, compared with the diabetic group. In the diabetic group, the numbers of fragmented or arrested embryos (Figure 4) during IVF and IVD were significantly (***P < 0.001) higher compared with the control group, however Stevia therapy could significantly (# P < 0.05) attenuate embryo blockage and degeneration (Figure 4), compared with the diabetic group.
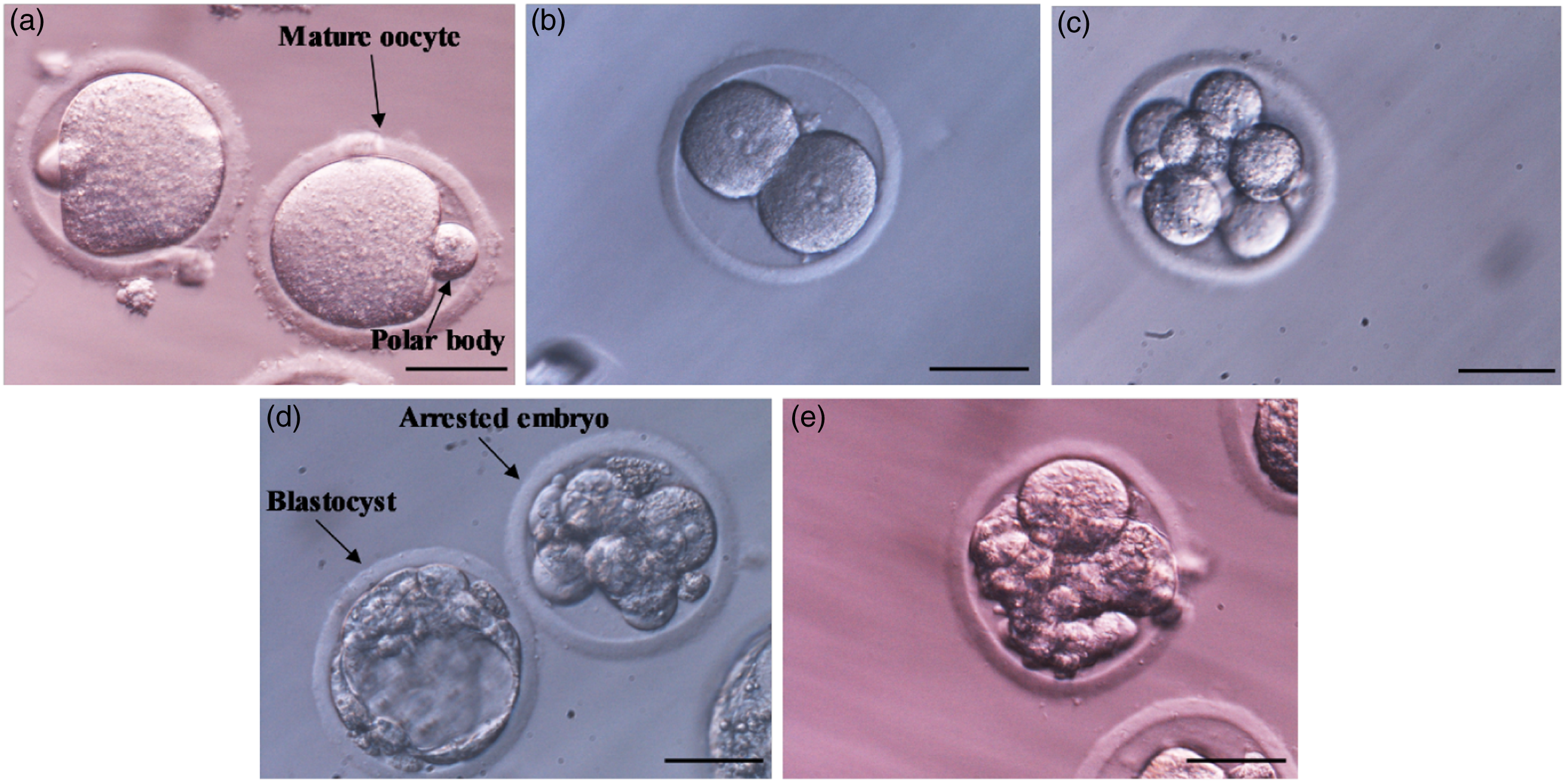
Figure 4. Morphology of the early embryo development resulted from in vitro fertilization. (a) Mature oocytes (MII) with polar body. (b) Two-cell stage embryo after IVF. (c) Eight-cell stage embryo. (d) Blastocyst. Upper arrow indicates arrested embryo. (e) Fragmented embryo. Scale bars: 50 μm.
Table 1. Effect of hydroalcoholic extraction of Stevia on the IVF success rate and the percentage of in vitro embryo development

Note. Data are presented as mean ± SD from three independent experiments using 100 MII oocytes each trial. Significant differences within the same column: ***P < 0.001 vs. the control group. #P < 0.05, ##P < 0.01 vs. the diabetic group.
Discussion
The results of this investigation supported previous findings that Stevia administration dramatically reduces raised FBS serum levels in streptozotocin-induced diabetic mice (Ahmad and Ahmad, Reference Ahmad and Ahmad2018; Gholizadeh et al., Reference Gholizadeh, Dastghaib, Koohpeyma, Bayat and Mokarram2019). Moreover, we showed that Stevia treatment improved sperm parameters and promoted IVF and IVD success in the streptozotocin diabetes model.
It is widely understood that diabetes-affected men frequently experience sexual dysfunction and reproductive disorders (Amaral et al., Reference Amaral, Oliveira and Ramalho-Santos2008). According to research, testicular oxidative stress caused by diabetes is the primary reason for male reproductive impairment (Zhao et al., Reference Zhao, Song, Wang, Wang, Jin, Xu, Bai, Li, Cui and Cai2018; Nna et al., Reference Nna, Bakar, Ahmad and Mohamed2020). Specifically, reactive oxygen species (ROS) can suppress steroid hormone synthesis by affecting steroidogenic enzymes (Quinn and Payne, Reference Quinn and Payne1984).
STZ induces diabetes by causing a rapid drop in pancreatic beta cells, which leads to a marked decrease in insulin supply. Hyperglycaemia produced by insulin insufficiency, in turn, triggers oxidative damage by ROS production (Stadler, Reference Stadler2013). Moreover, it has been reported that blood levels of LH and testosterone, testis volume, and weight, seminiferous tubule, and germinal epithelium volume, sperm count, and motility were all reduced in the STZ model of diabetes (Ballester et al., Reference Ballester, Muñoz, Domínguez, Rigau, Guinovart and Rodríguez-Gil2004; Kianifard et al., Reference Kianifard, Sadrkhanlou and Hasanzadeh2011).
Consistent with previous studies, our findings revealed that elevated serum FBS levels accompanied STZ-induced diabetes in mice due to the fast depletion of beta cells. (Karganov et al., Reference Karganov, Alchinova, Tinkov, Medvedeva, Lebedeva, Ajsuvakova, Polyakova, Skalnaya, Burtseva, Notova, Khlebnikova and Skalny2020). As expected, our results showed that the administration of hydroalcoholic extraction of Stevia for 6 weeks was able to reduce blood FBS levels in diabetic mice. Stevia regulates blood glucose levels by revitalizing beta cells that, consequently, restored the glycogen synthase system by enhancing insulin production and liver glycogen storage (Ray et al., Reference Ray, Kumar, Laor, Shereen, Nwamaghinna, Thomson, Perez, Soni and McFarlane2020; Dastghaib et al., Reference Dastghaib, Koohpeyma, Khazayel, Gholizadeh, Pour, Mokaram and Noorafshan2022). Our findings are in line with prior research that found that aqueous Stevia extract decreased fasting blood glucose levels in diabetic rats (Assaei et al., Reference Assaei, Mokarram, Dastghaib, Darbandi, Darbandi, Zal, Akmali and Ranjbar Omrani2016; Hussain and Hafeez, Reference Hussain and Hafeez2021).
Testosterone, a fundamental Leydig cell-secreted sex hormone, is necessary for producing spermatozoa and the development of secondary sexual characteristics (Zirkin and Papadopoulos, Reference Zirkin and Papadopoulos2018). Previous research has shown that low serum testosterone levels in diabetic men lead to impaired spermatogenesis (Ding et al., Reference Ding, Liu, Liu, Pan, Guo, Sheng and Huang2015). Concerning the effect of hyperglycaemia on male fertility, we observed lower blood testosterone levels in diabetic mice compared with nondiabetic mice. However, Stevia treatment significantly increased serum testosterone levels in diabetic mice. These results are in contrast with previous research that showed that Stevia administration did not affect low testosterone levels in STZ-induced diabetic rats (Gholizadeh et al., Reference Gholizadeh, Dastghaib, Koohpeyma, Bayat and Mokarram2019). In agreement, Gil et al. (Reference Gil, Lingan, Flores and Chimoy2008) reported that prolonged Stevia medication resulted in elevated testosterone levels in albino mice. It has been discovered that Leydig cells possess insulin receptors that have been related to testosterone production. Because of insufficient insulin synthesis, people with diabetes have experienced lower testosterone levels (Pitteloud et al., Reference Pitteloud, Hardin, Dwyer, Valassi, Yialamas, Elahi and Hayes2005; Ahn et al., Reference Ahn, Gang, Kim, Ahn, Harris, Lee and Choi2013). Moreover, it has been shown that reduced insulin levels have an adverse effect on Leydig cell activity by lowering blood LH levels, perhaps by a process involving follicle-stimulating hormone (FSH; Ballester et al., Reference Ballester, Muñoz, Domínguez, Rigau, Guinovart and Rodríguez-Gil2004; Schoeller et al., Reference Schoeller, Albanna, Frolova and Moley2012).
As previously noted, diabetes might have triggered oxidative stress, which is deleterious to the male reproductive system, sperm morphology, sperm function, and DNA integrity (Laleethambika et al., Reference Laleethambika, Anila, Manojkumar, Muruganandam, Giridharan, Ravimanickam and Balachandar2019; Zhong et al., Reference Zhong, Ji, Wang, Lei and Huang2021). In line with previous research, our findings showed that STZ administration had a detrimental effect on mice sperm count, sperm motility, and morphology (Talebi et al., Reference Talebi, Mangoli, Nahangi, Anvari, Pourentezari and Halvaei2014). However, long-term Stevia therapy significantly improved sperm parameters, in agreement with previous studies (Gholizadeh et al., Reference Gholizadeh, Dastghaib, Koohpeyma, Bayat and Mokarram2019). Stevia has also been shown to improve the oxidative response in the testis of diabetic rats (Gholizadeh et al., Reference Gholizadeh, Mokarram, Dastgheib and Rahpeima2018). Moreover, a recent study revealed that Stevia rebaudiana markedly improved antioxidant-related enzymes and spermatogenesis in rats suffering from ischaemia/reperfusion injury (Ganjiani et al., Reference Ganjiani, Ahmadi and Raayat Jahromi2020). The process of sperm production is linked to the normal function of Leydig and Sertoli cells, as well as adequate levels of male sex hormones, which have been affected by diabetes-associated oxidative stress (SenGupta et al., Reference SenGupta, Arafa and Elbardisi2019). As expected, due to possessing antioxidant properties, Stevia could promote sperm parameters in STZ-induced mice. In fact, we are still in the early stages of understanding how Stevia regulates testosterone biosynthesis, and additional research is required before we can propose a precise mechanism.
Even though both sexes contribute genetically and epigenetically to fertilization and embryo development, the male effect on embryo development is well understood. Therefore, sperm quality has a detrimental role in embryo formation (Danis and Samplaski, Reference Danis and Samplaski2019). For the first time, we found that STZ-induced diabetes mice had a decreased IVF success rate and a lower potential to reach the blastocyst stage compared with control mice. Conversely, Stevia significantly promoted IVF outcomes in diabetic mice. Moreover, we demonstrated that Stevia therapy significantly improved in vitro development of fertilized oocytes when compared with diabetic animals. Similarly, recent research has suggested that Stevia ameliorates letrozole-induced PCOS through anti-inflammatory, antioxidant, and angiogenesis-regulating mechanisms (Morsi et al., Reference Morsi, Mersal, Farrag, Abdelmoneim, Abdelmenem and Salim2022). It has been well understood that fertilization and embryo development are negatively influenced by low sperm quality (Simon et al., Reference Simon, Proutski, Stevenson, Jennings, McManus, Lutton and Lewis2013). In fact, improved sperm parameters as a result of antioxidant therapy may lead to an increase in the pregnancy rate (Panti et al., Reference Panti, Shehu, Saidu, Tunau, Nwobodo, Jimoh, Bilbis, Umar and Hassan2018). Stevia’s antioxidant features are credited to its high flavonoid, phenol, catalase, and peroxidase contents (Harismah et al., Reference Harismah, Mirzaei and Fuadi2018). Therefore, using herbal remedies such as Stevia may improve sperm parameters, which will then increase fertilization outcomes.
In conclusion, our study showed that, although experimental-induced diabetes had a decreased sperm fertility potential and fertilization success rate, the hydroalcoholic extract of Stevia, a powerful anti-diabetic and antioxidant agent, improved sperm parameters, IVF outcome, and in vitro embryonic developmental potential. This finding indicated that Stevia has promising applicability in diabetic patients, however additional studies are required.
Acknowledgements
This study was supported by a grant from the Kurdistan University of Medical Sciences and Health Services, Sanandaj, Iran (grant number: IR.MUK.REC.1397/350). We express our appreciation to all members of the Cellular and Molecular Research Center for their helpful consultation and deliberation during this study.
Funding
The current study was supported by a grant (number IR.MUK.REC.1397/350) to Erfan Daneshi, from the Kurdistan University of Medical Sciences and Health Services, Sanandaj, Iran.
Competing interests
The authors declare that they have no competing financial or nonfinancial interests.
Ethical standard
The experiments were conducted with the approval of the Ethics Committee of the Kurdistan University of Medical Sciences.