Introduction
Common bean (Phaseolus vulgaris L.) (Fabales: Fabaceae) is an important human food source consumed worldwide, with a remarkable content of proteins, carbohydrates, vitamins, among other essential elements for human nutrition (Carović-Stanko et al., Reference Carović-Stanko, Liber, Vidak, Barešić, Grdiša, Lazarević and Šatović2017). The common bean has its origin in the Americas (Borém and Miranda, Reference Borém and Miranda2013), and is currently cultivated in virtually all parts of the world (Ozturk et al., Reference Ozturk, Kara, Yildiz and Ercisli2009), especially in African and Latin American countries (Kamfwa et al., Reference Kamfwa, Cichy and Kelly2015).
Common bean is attacked by arthropod pests such as Bemisia tabaci (Gennadius) (Hemiptera: Aleyrodidae), Tetranychus urticae Koch (Acari: Tetranychidae), Chrysodeixis includens (Walker) (Lepidoptera: Noctuidae), and Diabrotica speciosa (Germar) (Coleoptera: Chrysomelidae) (Oliveira et al., Reference Oliveira, Oliveira, Wendland, Guimarães, Quintela, Barbosa, Carvalho, Lobo Junior and Silveira2018). The latter is a major defoliator of common bean in South America, e.g., 25% defoliation by D. speciosa can reduce grain production by up to 21.7% (Salas and Bueno, Reference Salas and Bueno2019). In addition, this species is regarded as a threat to the agriculture of other countries where it is currently absent, such as the United States (CAPS, 2020).
Diabrotica speciosa is holometabolous, and both the larvae and adults are agricultural pests (Medina et al., Reference Medina, Trecha and Da Rosa2013). During the larval phase, D. speciosa feeds on roots of maize (Zea mays L.) (Cyperales: Poaceae), peanut (Arachis hypogaea L.) (Fabales: Fabaceae) and potato tubers (Solanum tuberosum L.) (Solanales: Solanaceae), whereas adults feed on common bean, soybean [Glycine max (L.) Merr.] (Fabales: Fabaceae), cotton (Gossypium L.) (Malvales: Malvaceae), potato, sunflower (Helianthus L.) (Asterales: Asteraceae), tobacco (Nicotiana tabacum L.) (Solanales: Solanaceae), canola (Brassica napus L.) (Brassicales: Brassicaceae) and many more plants (Cabrera Walsh et al., Reference Cabrera Walsh, Ávila, Cabrera, Nava, Pinto and Weber2020). In Brazil, D. speciosa is managed with insecticides and Bt crops. However, the intensive application of insecticides to control insect pests has produced many negative effects on non-target organisms, such as beneficial insects and birds, and other environmental impacts (Salazar-Magallon et al., Reference Salazar-Magallon, Hernandez-Velazquez, Alvear-Garcia, Arenas-Sosa and Pena-Chora2015).
Overall, the few options considered promising to control D. speciosa (especially in bean crops) are entomopathogenic microorganisms, e.g., Beauveria bassiana and Heterorhabditis bacteriophora, semiochemical attractants, and plant resistance (Cabrera Walsh et al., Reference Cabrera Walsh, Ávila, Cabrera, Nava, Pinto and Weber2020).
Plant resistance to insects is originally classified in three distinct categories: antibiosis, antixenosis (or non-preference), and tolerance (Painter, Reference Painter1951). Antibiosis is observed when a resistant plant adversely affects insect growth, survival, and/or its offspring (Painter, Reference Painter1951). Antixenosis comprises negative effects of a resistant plant on insect behavior, thus discouraging oviposition, feeding, or even shelter (Painter, Reference Painter1951; Kogan and Ortman, Reference Kogan and Ortman1978). Conversely, a tolerant plant can withstand or recover from insect attack and exerts no significant impact on the growth or survival of the herbivore (Smith and Clement, Reference Smith and Clement2012).
Few studies on resistance in common bean genotypes to D. speciosa have been published. Boiça Júnior et al. (Reference Boiça Júnior, Costa, Souza, Ribeiro and Carbonell2015) evaluated resistance of 10 common bean cultivars to the feeding of D. speciosa, and found that cultivar ‘Ônix’ was the least preferred by adults in a no-choice feeding preference test and one of the more tolerant to larval feeding. Paron and Lara (Reference Paron and Lara2005) examined resistance levels in six common bean cultivars, and concluded that cultivar ‘Goiano Precoce’ was the least preferred by D. speciosa. However, none of them evaluated resistance of common bean landraces to this important pest, levels of resistance-related enzymes (peroxidase [POD] and superoxide dismutase [SOD]), or seed production of infested and uninfested plants.
The current study tested the hypothesis that common bean landraces may express higher resistance levels to the feeding of D. speciosa adults than the commercial susceptible cultivar, ‘BRS Esplendor’. The susceptibility of this cultivar was reported by Boiça Júnior et al. (Reference Boiça Júnior, Costa, Souza, Ribeiro and Carbonell2015). Thus, the current study aimed to evaluate resistance in several genotypes (landraces and cultivars) of common bean to D. speciosa, under laboratory and greenhouse conditions, correlating the resistance data with trichome density, protein content, and levels of POD and SOD in leaves of infested and uninfested plants.
Materials and methods
Insect rearing
Larvae of D. speciosa were reared on maize roots from the variety Milho Roxo, Z. mays. Seventy 5-d-old maize seedlings were offered to seventy neonate larvae in a plastic container (17 × 9 cm) filled with fine-grained vermiculite moistened with 50 g of deionized water; 10 days later, the larvae were transferred to larger containers (27 × 16 × 9.5 cm) and the maize seedlings were replaced by other 140 fresh maize seedlings. Differently, while adults were fed on leaves of common bean from Carioca commercial group (Ávila et al., Reference Ávila, Tabai and Parra2000). Bean leaves were replaced twice a week to provide continually fresh leaves for beetle feeding. The insect colony and laboratory experiments were held at the Laboratory of Entomology of the Federal University of Grande Dourados (UFGD; Universidade Federal da Grande Dourados), municipality of Dourados, MS, Brazil. Environmental conditions in the laboratory were 25°C temperature, 70% relative humidity, and 12/12 h light/dark regime.
Laboratory experiment
Fourteen landraces and two cultivars were initially screened for resistance to D. speciosa. The landraces were: Argentino, Carioca Pintinho, Cavalo Bell, Cavalo BRPR, Chumbinho Branco, Chumbinho Lustoso, Costa Rica, Dobalde, Jalo Bege, Manteigado, Paco Mineiro, Pombinho, Vinagrinho, and 90D Mouro; and the cultivars were: IPR Tuiuiú (developed by Instituto Agronômico do Paraná – IAPAR) and BRS Esplendor (developed by Empresa Brasileira de Pesquisa Agropecuária – Embrapa). The latter cultivar was adopted as a susceptible standard based on a previous study (Boiça Júnior et al., Reference Boiça Júnior, Costa, Souza, Ribeiro and Carbonell2015). The landraces used in this study are cultivated and preserved by small farmers from the state of Paraná, Brazil.
Plants were grown in a greenhouse of the School of Agricultural Sciences (FCA; Faculdade de Ciências Agrárias), UFGD, which has an exhaust fan and a pad-fan cooling system used to maintain internal temperature at 28°C, under natural conditions of luminosity and relative humidity. Plants were cultivated in 7 L-plastic pots filled with a blend of three parts of soil (Dystroferric Red Latosol) and one part of organic compost (Plante Verde, Guarapuava, PR, Brazil). Eight seeds of each common bean genotype were sown per pot, and watered when necessary. Thinning was carried out 15 d after plant emergence (DAE), keeping three plants per pot. Four pots were used for each common bean genotype, totaling 12 plants per genotype. Pots were covered with voile fabric (12-m length, 4-m diameter) supported by eight 1.5-m aluminum columns.
Antixenosis in common bean to D. speciosa was evaluated by choice and no-choice feeding experiments. The tests were arranged in a completely randomized design and used leaf disks obtained from leaflets detached from the upper part of 25-d-old plants, approximately V3-V4 phenological stage (Oliveira et al., Reference Oliveira, Oliveira, Wendland, Guimarães, Quintela, Barbosa, Carvalho, Lobo Junior and Silveira2018). For both experiments, 10 replicates were used per treatment.
Due to space limitations of the plastic arenas (15.0 cm diameter × 1.0 cm height), in the choice test, leaf disks of the 16 common bean genotypes were distributed into three different groups. The first group was constituted by the genotypes Argentino, Carioca Pintinho, Cavalo Bell, Cavalo BRPR, Chumbinho Branco, and BRS Esplendor. In the second group, the genotypes tested were Chumbinho Lustoso, Costa Rica, Dobalde, Jalo Bege, Manteigado, and BRS Esplendor. The third group was composed of the genotypes Paco Mineiro, Pombinho, IPR Tuiuiú, Vinagrinho, 90D Mouro, and BRS Esplendor. One 2.6-cm-diameter leaf disk of each genotype was placed in the plastic containers lined with moistened filter paper, at ca. 4 cm between each other (total of 6 leaf disks per plastic container). Next, six 7-d-old D. speciosa adults were released in the center of each container. One plastic container with one leaf disk of each common bean genotype group and six D. speciosa adults represented a replicate. Each experiment was ended when one of the leaf disks had a consumption of 80%. Thus, experiment of group 1 lasted 17 h; group 2 was concluded 21 h after releasing the insects; and group 3 lasted 19 h.
In the no-choice test, the same 16 common bean genotypes were tested. One 2.6-cm-diameter leaf disk of each genotype was placed in the center of a Petri dish (9.0 cm diameter × 1.2 cm height) lined with moistened filter paper. Then, one adult was released in each Petri dish, and the experiment lasted 23 h. One leaf disk placed in a Petri dish and exposed to the feeding of a D. speciosa adult represented a replicate. Percentage of leaf consumption was measured using the mobile app BioLeaf (Machado et al., Reference Machado, Orue, Arruda, Santos, Sarath, Gonçalves, Silva, Pistori, Roel and Rodrigues2016).
Greenhouse experiment
Six common bean genotypes were selected according to the resistance levels shown in the choice and no-choice tests described above: Chumbinho Branco (resistant in the no-choice test), Dobalde (resistant in the no-choice test), Manteigado (resistant in the choice and no-choice tests), IPR Tuiuiú (resistant in the choice and no-choice tests), 90D Mouro (moderately resistant in the choice and no-choice tests), and BRS Esplendor (susceptible standard).
Plants were grown using the same materials and methodologies as detailed in the previous section. However, plants were individually covered with voile fabric bags/cages (1.50-m length, 0.50-m diameter) supported by two bamboo stakes driven into the soil and tied with string. Thinning was accomplished 15 d after plant emergence, keeping two plants per pot (replicate).
This experiment was performed using a completely randomized design, with six treatments (common bean genotypes) and 10 replicates. However, data on plant height and numbers of leaves were arranged in a factorial scheme 6 × 2 (genotypes × infestation treatment [infested or uninfested plants]). A replicate was composed of two bean plants infested with a D. speciosa pair. In total, we used 20 infested and 20 uninfested plants per genotype.
One 7-d-old D. speciosa pair was released into each cages (plants at V2-V3 phenological stage) using a 50-ml Falcon tube. They were kept in the cages for a period of 30 days. Percentage of survival of D. speciosa, plant height (cm), numbers of leaves per plant, and percentage of injured leaves were assessed at this point. The percentage of injury per leaf and weight (mg) of seeds were evaluated at 90 days after sowing. The percentage of injury per leaf was assessed for five leaves from the top of bean canopy, with the aid of the mobile app BioLeaf (Machado et al., Reference Machado, Orue, Arruda, Santos, Sarath, Gonçalves, Silva, Pistori, Roel and Rodrigues2016). Weight of seeds was measured using a precision analytical balance (model 2204, Bioscale, China).
Trichome density, total proteins, and resistance-related enzymes
Ten leaves from different plants (infested and uninfested) of each common bean genotype were collected randomly in the upper canopy (youngest leaves) 15 days after releasing the D. speciosa pairs. Leaves were kept in a styrofoam box with ice and brought to the laboratory, where they were kept in a freezer (model DF42, Electrolux, Brazil) at −20°C. Trichome density was evaluated using a stereoscopic microscope (model GFP241DA-1220, Motic, Xiamen, China). Counting of trichome density consisted of four areas of 1 cm2 per leaf, two in the adaxial face and other two in the abaxial face. These leaves were stored at −20°C until performing analyses of total proteins and enzymes, peroxidase (POD) and superoxide dismutase (SOD). This analysis was carried out under a completely randomized design, using 10 replicates (leaves) per genotype.
Total proteins contents in leaves of the different common bean genotypes were obtained according to the methodology of Bradford (Reference Bradford1976), while the levels of POD and SOD were determined based on methodology developed by Broetto (Reference Broetto2014). For these analyses, four leaves collected from four different common bean plants of the same landrace/cultivar composed a replicate. The experiments used a completely randomized design, with six treatments (common bean genotypes) and four replicates. However, data on trichome density used a factorial design 6 × 2 (genotypes × infestation treatment [infested or uninfested plants]).
Statistical analysis
The data obtained in all experiments were examined for normality of residuals (Shapiro and Wilk, Reference Shapiro and Wilk1965) and homogeneity of variances (Levene, Reference Levene, Olkin, Ghurye, Hoeffding, Madow and Mann1960) using a statistical software package (SAS Institute, 2003). Then, data were analyzed with one-way ANOVA, and the means that were significantly different were compared using tests of means separation (Tukey, Reference Tukey1949; Duncan, Reference Duncan1955; Student's t test) (α = 0.05) (SAS Institute, 2003). Data on choice (group 1) and no-choice feeding tests did not meet the normality assumptions. Thus, the non-parametric test of Kruskal and Wallis (Reference Kruskal and Wallis1952) was used, followed by a post-hoc test (Dunn, Reference Dunn1964). Similarly, data on levels of POD and SOD, and contents of total proteins did not meet the normality requirements, and therefore were compared using Wilcoxon–Mann–Whitney test (Moses, Reference Moses2014). Non-parametric tests were performed using R software (R Development Core Team, 2019).
Results
Laboratory experiment
In the choice test, no differences were observed in percentage of leaf consumption considering the common bean genotypes evaluated in the group 1 (H = 11.65; df = 5, 54; P > 0.05) (fig. 1a). Conversely, in the group 2 the landrace Manteigado was the least consumed, differing from the genotypes Chumbinho Lustoso, Dobalde, and BRS Esplendor (F = 12.45; df = 5, 54; P < 0.0001) (fig. 1b). In the group 3, the cultivar IPR Tuiuiú showed a lower leaf consumption than the genotypes Pombinho, Vinagrinho, and BRS Esplendor (F = 8.38; df = 5, 54; P < 0.0001) (fig. 1c).

Figure 1. Leaf consumption of Diabrotica speciosa (mean ± SE) on 16 common bean genotypes in choice tests. The genotypes were separated into 3 groups, where the cultivar BRS Esplendor was used as susceptibility standard. Different letters above the bars indicate results statistically different according to Kruskal–Wallis test (Group 1) or Tukey's test (α = 0.05). A, Group 1; B, Group 2; C, Group 3.
In the no-choice test, lower leaf consumption was noted on the genotypes Chumbinho Branco, Dobalde, Manteigado, and IPR Tuiuiú compared with the genotypes Argentino, Costa Rica, Jalo Bege, and BRS Esplendor (H = 49.44; df = 15, 144; P < 0.0001) (fig. 2). The genotypes Cavalo Bell, Cavalo BRPR, Chumbinho Lustoso, Paco Mineiro, Pombinho, and Vinagrinho were not significantly different compared with resistant and susceptible genotypes.

Figure 2. Leaf consumption of Diabrotica speciosa (mean ± SE) on 16 common bean genotypes under no-choice test. The cultivar BRS Esplendor was used as susceptibility standard. Different letters above the bars represent results statistically different by the Kruskal–Wallis test (α = 0.05).
Greenhouse experiment
Statistically significant differences in height were observed between different genotypes and between infested and non-infested plants; however, no differences were detected in plant height when considering the interaction between ‘genotype’ × ‘infestation treatment’ (F = 1.02; df = 5, 107; P = 0.4120). The varieties Chumbinho Branco and Dobalde were significantly taller than the cultivar IPR Tuiuiú (F = 3.67; df = 5, 107; P = 0.0042) (Table 1), while the genotypes Manteigado, 90D Mouro, and BRS Esplendor had similar height compared with resistant and susceptible genotypes. The D. speciosa-infested plants were significantly taller (39.42 ± 2.41) than the uninfested (or control) plants (28.55 ± 1.13) (F = 19.44; df = 1, 107; P < 0.0001).
Table 1. Plant height, percentage of injured leaves, and percentage of injury per leaf (mean ± SE) of common bean genotypes submitted to the feeding of Diabrotica speciosa
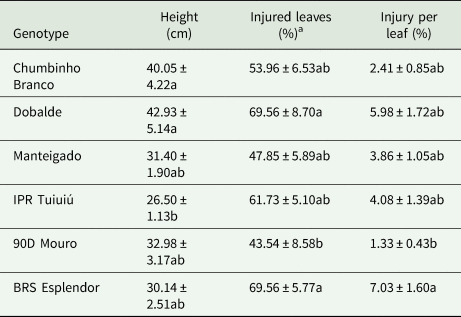
Means followed by different letters, in the column, are significantly different by Tukey's test or Duncan's testa (α = 0.05).
The number of leaves was not significantly different for the factor ‘genotype’ (F = 0.74; df = 5, 107; P = 0.5951; values varied from 9.80 to 10.90 leaves) and the interaction between ‘genotype’ vs ‘infestation treatment’ (F = 1.13; df = 5, 107; P < 0.3488). However, a greater number of leaves was produced by plants infested with a D. speciosa pair (11.38 ± 0.32) compared with uninfested plants (9.28 ± 0.30) (F = 22.28; df = 1, 107; P < 0.0001).
A higher percentage of injured leaves was found in plants of the genotypes Dobalde and BRS Esplendor than in plants of the landrace 90D Mouro (F = 2.56; df = 5, 54; P = 0.0380) (Table 1). In addition, the landrace 90D Mouro showed a lower percentage of injury per leaf than the cultivar BRS Esplendor (F = 2.97; df = 5, 45; P = 0.0212) (Table 1). No significant differences were seen with regards to survival of D. speciosa (H = 3.96; df = 5, 54; P = 0.5552), in which values ranged from 20 to 50%.
Attack of a D. speciosa pair did not reduce the total weight of seeds of the genotypes Dobalde (F = 0.81; df = 1, 18; P = 0.3805) (fig. 3a), Manteigado (F = 2.12; df = 1, 15; P = 0.1664) (fig. 3b), IPR Tuiuiú (F = 0.15; df = 1, 14; P = 0.7067) (fig. 3c), and 90D Mouro (F = 0.72; df = 1, 18; P = 0.4076) (fig. 3d). In contrast, infested plants of the cultivar BRS Esplendor showed a lower total weight of seeds than uninfested plants (F = 4.77; df = 1, 17; P = 0.0433) (fig. 3e). Overall, infested or uninfested plants of the landrace Chumbinho Branco yielded an insufficient number of seeds to allow for statistical analysis. This may be due to its longer period to complete seed formation.

Figure 3. Mean weight of seeds (mean ± SE) produced by plants of different common bean genotypes infested or uninfested with a Diabrotica speciosa pair (greenhouse experiment). The cultivar BRS Esplendor was used as susceptibility standard. Different letters above the bars represent results statistically different by the Student's t-test (α = 0.05).
Trichome density
Significant differences in trichome density were observed between bean genotypes and infestation categories, as well as for the interaction between these factors (F = 3.85; df = 5, 108; P = 0.0030). A higher trichome density was found in leaves of the varieties Manteigado and 90D Mouro compared with the genotypes Chumbinho Branco, Dobalde, IPR Tuiuiú, and BRS Esplendor (F = 38.49; df = 5, 108; P < 0.0001) (fig. 4).

Figure 4. Numbers of trichomes (1 cm2, mean ± SE) found in leaves of different common bean genotypes (factor ‘genotype’ of the factorial analysis) 15 days after D. speciosa release. The cultivar BRS Esplendor was used as susceptibility standard. Different letters above the bars indicate results statistically different by the Tukey's test (α = 0.05).
Overall, common bean plants infested with a D. speciosa pair presented a lower trichome density (276.42 ± 44.67) than uninfested plants (512.97 ± 78.09) (F = 42.89; df = 1, 108; P < 0.0001). Regarding the interaction between ‘genotype’ and ‘infestation treatment’, statistical differences were observed depending on the genotype. A lower trichome density was seen in infested leaves of the genotypes Chumbinho Branco (F = 13.66; df = 1, 108; P = 0.0003), Dobalde (F = 20.53; df = 1, 108; P < 0.0001), Manteigado (F = 17.16; df = 1, 108; P = 0.0001), and IPR Tuiuiú (F = 10.36; df = 1, 108; P = 0.0017) compared with uninfested leaves (Table 2). In contrast, no significant differences in trichome density were detected when comparing infested and uninfested leaves of the genotypes 90D Mouro (F = 0.03; df = 1, 108; P = 0.8549) and BRS Esplendor (F = 0.41; df = 1, 108; P = 0.5249) (Table 2).
Table 2. Interaction between common bean genotypes and numbers of trichomes per cm2 (mean ± SE) in leaves of plants infested or uninfested with a Diabrotica speciosa pair
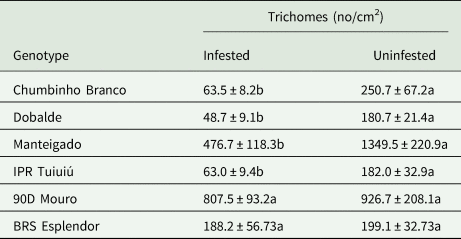
Evaluation performed at 15 days after infestation.
Means followed by different letters, in the row, are significantly different by the Tukey's test (α = 0.05).
Total proteins
The total protein contents was unchanged relative to D. speciosa-infested and uninfested leaves of the genotypes Chumbinho Branco (U = 8.50; df = 1, 6; P > 0.05), Dobalde (U = 4.00; df = 1, 6; P = 0.3429), IPR Tuiuiú (U = 11.00; df = 1, 6; P = 0.4857), and BRS Esplendor (U = 11.00; df = 1, 6; P = 0.4857) (Table 3). Higher protein contents was observed in infested leaves of the landrace Manteigado than in uninfested leaves (U = 0.00; df = 1, 6; P = 0.0286). Oppositely, lower total protein contents were produced in infested leaves of the landrace 90D Mouro than in uninfested leaves (U = 16.00; df = 1, 6; P = 0.0286). No differences were observed among bean genotypes concerning total proteins in infested (H = 9.28; df = 5, 15; P = 0.0984) or uninfested leaves (H = 11.63; df = 5, 15; P > 0.05) (Table 3).
Table 3. Protein contents, and levels of POD and SOD (mean ± SE) in leaves of Diabrotica speciosa infested and uninfested plants of different common bean genotypes
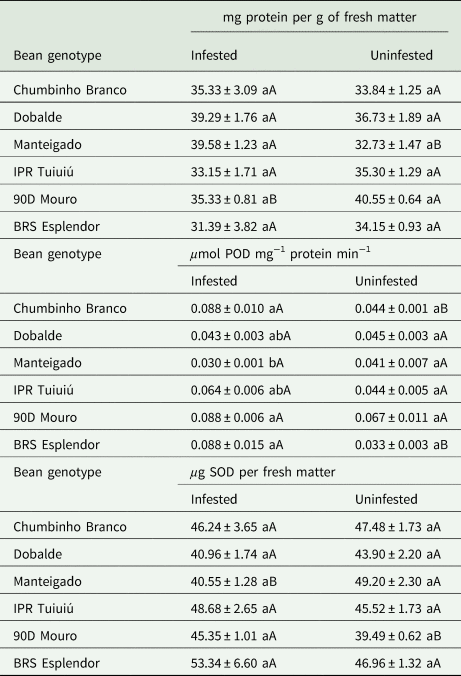
Evaluation performed at 15 days after infestation.
Means followed by different lowercase letters, in the column, are significantly different by the Kruskal–Wallis test, whilst means followed by different uppercase letters, in the row, are significantly different by the Wilcoxon–Mann–Whitney test (α = 0.05).
POD level
No statistical differences were obtained for POD level in infested and uninfested leaves of the genotypes Dobalde (U = 9.00; df = 1, 6; P = 0.8857), Manteigado (U = 12.00; df = 1, 6; P = 0.3065), IPR Tuiuiú (U = 1.00; df = 1, 6; P = 0.0571), and 90D Mouro (U = 4.00; df = 1, 6; P = 0.3429) (Table 3). However, higher POD levels were found in leaves of infested plants of the genotypes Chumbinho Branco (U = 0.00; df = 1, 6; P = 0.0286) and BRS Esplendor (U = 0.00; df = 1, 6; P = 0.0286) compared with leaves of uninfested plants (Table 3). Higher POD levels were noted in D. speciosa-infested leaves of the genotypes Chumbinho Branco, 90D Mouro, and BRS Esplendor compared to infested leaves of landrace Manteigado (H = 17.83; df = 5, 15; P = 0.0032). In contrast, no differences were found among bean genotypes for POD levels in uninfested leaves (H = 10.53; df = 5, 15; P = 0.0615) (Table 3).
SOD level
No significant differences were observed for SOD levels in D. speciosa-infested and uninfested leaves of the genotypes Chumbinho Branco (U = 7.50; df = 1, 6; P > 0.05), Dobalde (U = 12.00; df = 1, 6; P = 0.3429), IPR Tuiuiú (U = 5.00; df = 1, 6; P = 0.4857), and BRS Esplendor (U = 5.00; df = 1, 6; P = 0.4857) (Table 3). In contrast, a higher SOD level was found in leaves of infested plants of the landrace 90D Mouro than in uninfested leaves (U = 0.00; df = 1, 6; P = 0.0286). Conversely, a reduced SOD level was noted in leaves of infested plants of the landrace Manteigado compared with its uninfested leaves (U = 16.00; df = 1, 6; P = 0.0286) (Table 3). No significant differences for SOD levels were observed among bean genotypes in infested (H = 9.28; df = 5, 15; P = 0.0984) and uninfested leaves (H = 11.63; df = 5, 15; P > 0.05) (Table 3).
Discussion
Plant resistance is one of the most promising tactics to control D. speciosa in different crops, including common beans (Cabrera Walsh et al., Reference Cabrera Walsh, Ávila, Cabrera, Nava, Pinto and Weber2020). To date, few studies have been published on resistance of common bean genotypes to D. speciosa (Paron and Lara, Reference Paron and Lara2001, Reference Paron and Lara2005; Boiça Júnior et al., Reference Boiça Júnior, Costa, Souza, Ribeiro and Carbonell2015; Carneiro et al., Reference Carneiro, Kafer, Gobatto, Fedrigo, Andrade, Vargas and Finatto2022). However, none of them assessed (i) resistance of common bean landraces to this major pest, (ii) the constitutive and induced levels of trichome density, and (iii) seed production following D. speciosa attack.
In the present study, the resistance categories antixenosis and tolerance seemed to play a major role in common bean resistance to D. speciosa. For example, the landrace Dobalde exhibited a higher plant height, no changes in POD or SOD levels or total protein contents (apparently not stressed by D. speciosa feeding), and despite having a higher percentage of injured leaves, it showed no reduction in seed weight. Regarding the enzyme POD, it has multiple functions in plant defense, such as reduction in the nutritional composition of the leaf material (War et al., Reference War, Paulraj, Ahmad, Buhroo, Hussain, Ignacimuthu and Sharma2012), lignification, and wound healing (Chen, Reference Chen2008; War et al., Reference War, Paulraj, Ahmad, Buhroo, Hussain, Ignacimuthu and Sharma2012). The enzyme SOD has a major role in the scavenging of superoxide radicals (Zacheo and Bleve-Zacheo, Reference Zacheo and Bleve-Zacheo1988), thus contributing to plant tolerance by protecting plant cells from oxidative damage (Mallick and Mohn, Reference Mallick and Mohn2000).
Interestingly, the landrace Manteigado possessed an intermediate plant height and intermediate percentage of injured leaves and injury per leaf. In addition, its D. speciosa-infested leaves produced a higher protein content and a lower SOD level, referred to as susceptibility traits by Costa et al. (Reference Costa, Martins, Reis, Fernandes and Scalon2020). However, the landrace Manteigado had a higher trichome density, which may have avoided a greater leaf damage, thus preventing losses in seed production. Plants of the cultivar IPR Tuiuiú were characterized by low height and intermediate levels of leaf injury; nevertheless, feeding of D. speciosa did not change the POD or SOD levels or total protein contents. This means that an infestation with a D. speciosa pair caused no apparent stress to common bean plants of the cultivar IPR Tuiuiú, as evidenced in the weight of seeds produced by its infested plants. In general, infested plants of the common bean genotypes invested more resources for plant growth (height and number of leaves) than to increase the density of trichomes. This is an additional proof that common bean resistance to D. speciosa is mainly governed by tolerance-resistance category.
A classical example of antixenosis was shown by the landrace 90D Mouro. Plants of this landrace exhibited lower percentages of injured leaves and injury per leaf. Furthermore, leaves of the landrace 90D Mouro showed one of the highest trichome density, and D. speciosa-infested leaves contained a lower protein content and a higher SOD level than uninfested leaves, a plant resistance response similar to that reported by Costa et al. (Reference Costa, Martins, Reis, Fernandes and Scalon2020) in cowpea leaves infested with Spodoptera frugiperda (J.E. Smith) (Lepidoptera: Noctuidae) larvae. The resistance mechanisms expressed by the landrace 90D Mouro may have contributed to avoid a reduction in seed production.
It is clear in the current study that antibiosis did not play a role in the resistance to D. speciosa feeding, because beetle survival was not statistically different among the common bean genotypes. Furthermore, most resistant genotypes showed similar POD level (antinutritive factor) in their infested leaves compared to cultivar BRS Esplendor (susceptible standard). Therefore, we suggest that perhaps antibiosis is a resistance category more efficient to control immature than adult insect pests, since larvae and nymphs are more susceptible to plant toxins than full-grown insects (Vellau et al., Reference Vellau, Sandre and Tammaru2013).
Overall, the POD level in D. speciosa-infested leaves of the common bean genotypes was 3.29-fold lower compared with the levels of this enzyme found in S. frugiperda-infested leaves of cowpea genotypes (Costa et al., Reference Costa, Martins, Reis, Fernandes and Scalon2020). Differently from our common bean genotypes, some cowpea genotypes studied by Costa et al. (Reference Costa, Martins, Reis, Fernandes and Scalon2020) expressed high antibiosis levels to the herbivore. Thus, we suggest that POD activity in common bean plants is not related (at least directly) to the resistance against D. speciosa feeding. Another strong evidence supporting this lack of relationship was the higher POD level in D. speciosa-infested leaves of the cultivar BRS Esplendor (susceptible standard) compared with its uninfested leaves [similar results were found by Carneiro et al. (Reference Carneiro, Kafer, Gobatto, Fedrigo, Andrade, Vargas and Finatto2022)].; BRS Esplendor was the only genotype to show a reduced seed production as a result of the pest attack. Similarly, Han et al. (Reference Han, Wang, Bi, Yang, Huang, Zhao, Hu and Cai2009) showed that infestation with the aphid Sitobion avenae (F.) (Homoptera: Aphididae) increased the POD levels to a greater extent in leaves of susceptible than resistant wheat cultivars.
Four of the six common bean genotypes studied in the greenhouse presented lower trichome density following D. speciosa feeding, a phenomenon similarly observed by Bloomer et al. (Reference Bloomer, Lloyd and Symonds2014) in mechanically injured leaves of several genotypes of Arabidopsis thaliana (L.) Heynh. (Brassicales: Brassicaceae). However, in the current study, D. speciosa-infested leaves of the genotypes 90D Mouro (antixenosis resistant) and BRS Esplendor (susceptible standard) exhibited no decrease in trichome density compared with uninfested leaves. This seems to be an important resistance mechanism of the landrace 90D Mouro, that in addition to having a higher constitutive trichome density, showed remarkable stability (no inhibition in trichome formation) after D. speciosa feeding possibly mediated (at least partly) by a higher SOD level. Therefore, the landrace 90D Mouro seems to express two resistance categories: antixenosis and tolerance, which was confirmed by data on seed weight. Curiously, infested leaves of the cultivar BRS Esplendor also showed no decrease in trichome density, despite being the most susceptible genotype. Perhaps this was an unsuccessful attempt of the plant to avoid a greater loss in seed production, due to the lack of other resistance mechanisms. However, the cultivar BRS Esplendor had one of the lowest total numbers of trichomes. The genotypes Dobalde, Manteigado, and IPR Tuiuiú showed decreased numbers of trichomes in infested leaves; however, as discussed above, these genotypes invested their resources in plant growth and/or seed production.
The results obtained in the laboratory were confirmed in the greenhouse experiment, demonstrating that choice and no-choice feeding tests are really useful to select the most resistant genotypes, as stated by Smith (Reference Smith2005). The four genotypes classified as resistant in the laboratory tests (Dobalde, Manteigado, IPR Tuiuiú, and 90D Mouro) showed no decrease in seed production in the greenhouse, in contrast to the susceptible standard, cultivar BRS Esplendor.
Three of the four common bean genotypes deemed resistant to D. speciosa in the greenhouse are landraces (Dobalde, Manteigado, and 90D Mouro). Landraces are farmer-developed varieties commonly adapted to environmental conditions, considered key sources of genetic diversity that can be used in plant breeding programs aimed at controlling insect pests (Corrado and Rao, Reference Corrado and Rao2017). Hence, because the aforementioned landraces and cultivar IPR Tuiuiú were the most resistant to D. speciosa, they have potential to be used in breeding programs with the aim of developing D. speciosa-resistant cultivars. Although field studies are needed to confirm this, the perfect parallelism between laboratory and greenhouse experiments suggests that the resistant genotypes are indeed promising to control the pest. This may result in the development of cultivars more tolerant to D. speciosa attack, i.e., more independent of chemical insecticides.
Acknowledgements
We are thankful to Coordenação de Aperfeiçoamento de Pessoal de Nível Superior (CAPES) for granting a Post-Doctoral Scholarship (PNPD) to the first author.
Author contributions
E. N. C. and M. G. F. conceived and designed research. E. N. C., E. S. G., L. C. R., A. C. F. and S. P. Q. S. conducted experiments. E. N. C. analyzed data and wrote the manuscript. M. G. F. and E. S. G. critically revised the manuscript.
Conflict of interest
The authors declare none.