Introduction
Parasitic nematodes are a group of multicellular organisms infecting and occupying a diverse range of hosts and ecosystems (Chung & Boeri Reference Chung and Boeri2012; Aleuy & Kutz Reference Aleuy and Kutz2020). They are a cause of animal disease (Charlier et al. Reference Charlier, Höglund, von Samson-Himmelstjerna, Dorny and Vercruysse2009; Mehlhorn Reference Mehlhorn and Mehlhorn2016) and can potentially impact the dynamics of wild populations (Tompkins et al. Reference Tompkins, Dobson, Arneberg, Begon, Cattadori, Greenman, Heesterbeek, Hudson, Newborn, Pugliese, Rizzoli, Rosa, Rosso, Wilson, Hudson, Rizzoli, Grenfell, Heesterbeek and Dobson2002), food webs (Lafferty et al. Reference Lafferty, Dobson and Kuris2006), and prey–predator interactions (Hudson et al. Reference Hudson, Dobson and Newborn1992; Hatcher et al. Reference Hatcher, Dick and Dunn2006). Parasitic nematodes and their development, survival, and distribution/migration in and on the herbage are sensitive to abiotic factors such as moisture and temperature (Crofton Reference Crofton1965; Stromberg Reference Stromberg1997; O’ Connor et al. Reference O’ Connor, Walkden-Brown and Kahn2006; Gyeltshen et al. Reference Gyeltshen, Kahn and Laurenson2022). Thus, changing environmental factors associated with climate change are expected to affect parasitic nematodes, with possible consequences for parasite–host dynamics (Kutz et al. Reference Kutz, Jenkins, Veitch, Ducrocq, Polley, Elkin and Lair2009; Molnár, Kutz et al. Reference Molnár, Kutz, Hoar and Dobson2013; Dobson et al. Reference Dobson, Molnár and Kutz2015). However, there has been no recent review of the knowledge gaps about the effect of temperature and moisture on direct life cycle parasitic nematodes in birds and mammals. Highlighting the knowledge gaps about abiotic effects, and particularly the interaction of moisture and temperature (O’ Connor et al. Reference O’ Connor, Walkden-Brown and Kahn2006) on parasitic nematodes, could guide future research and provide a better understanding of the implications for livestock health management, wildlife conservation, and ecosystem functioning in a rapidly warming climate.
In this review, we focus on parasitic nematodes with a direct life cycle. This group of parasites has one definitive host and a free-living stage outside the host where they occupy highly diverse habitats (e.g., faeces and herbage; Figure 1) and may be vulnerable to changes in abiotic conditions (Molnár, Dobson et al. Reference Molnár, Dobson and Kutz2013; Molnár, Kutz et al. Reference Molnár, Kutz, Hoar and Dobson2013). In an attempt to understand environmental effects on parasitic nematodes with a direct life cycle, many studies have focused on the seasonal correlation between environmental factors and nematode abundance (Chaudary et al. Reference Chaudary, Qayyum and Miller2008; van Dijk et al. Reference van Dijk, Sargison, Kenyon and Skuce2010; Halvorsen Reference Halvorsen2012; Holand et al. Reference Holand, Jensen, Kvalnes, Tufto, Pärn, Sæther and Ringsby2019) and behaviour (Langrová et al. Reference Langrová, Jankovská, Borovský and and Fiala2003; Kuzmina et al. Reference Kuzmina, Kuzmin and Kharchenko2006; Santos et al. Reference Santos, Silva and Amarante2012). Although seasonality studies are important in shedding light into the role of annual environmental change, the underlying mechanisms influencing the parasite dynamics often remain unknown (Altizer et al. Reference Altizer, Dobson, Hosseini, Hudson and Pascual2006) and need to be disentangled with experimental studies. Therefore, to tease apart the diverse factors and mechanisms that affect parasite–host dynamics, we have focused on reviewing experimental field and laboratory studies on the effect of abiotic conditions on parasitic nematodes with a direct life cycle in birds and mammals. Our primary aim is to identify the knowledge gaps by reviewing the literature describing studies that manipulated the effects of temperature, moisture, and precipitation on hatching, development, survival, and behaviour of parasitic nematodes in the free-living stage.

Figure 1. Schematic figure of the life cycle of parasitic nematodes with a direct life cycle that occupy highly diverse habitats (faeces, herbage, and inside the host) during the different life stages. 1) Parasitic nematodes with a direct life cycle have one definite host, in which exsheathment, reproduction, survival and egg production occurs. 2) In the free-living stage outside the host, eggs develop into infective larvae. 3) Infective larvae move out of the faeces and 4) are ingested by the host again.
Methods
For this review, we researched articles in Web of Science (© Copyright Clarivate 2021) on the effects of temperature, precipitation, and moisture on parasitic nematodes, with the last search date on 5 December 2022. The search string employed was (Parasit*) AND (nematod* OR roundworm) AND (temperature OR moisture OR humidity OR precipitation). This search resulted in 1,391 papers. From this total, only studies that fulfilled all the following criteria were included in the subsequent analysis: a) parasite with direct life cycle, b) parasite of terrestrial mammal or bird, c) effect of temperature, moisture, precipitation, or humidity on life-history traits (hatching, development, or survival) or behaviour of parasitic nematodes in the free-living stage, and d) experimental study under laboratory or field conditions. We specifically excluded studies on the effect of abiotic factors on the soil nematode community and studies not written in English. Most of the excluded studies were on plant and insect parasitic nematodes, soil communities, or observational studies without experimental manipulation. Finally, we added one relevant study (PhD thesis) that was missed by the literature search but that was previously known (Hoar Reference Hoar2012) to give a total of 57 relevant papers to review. In the next section, we synthesize the results of the reviewed papers. When findings are based on evidence from three or more papers, we only refer to the oldest (first documentation) and most recent relevant paper in the text here. In parentheses, we indicate the number of papers on the specific topic with np, while nr refers to the total number of results described in the different papers (as some papers describe results of multiple species or life stages). We refer the reader to the references of the reviewed studies provided in the Supplementary Material.
Results
The main findings are described below and summarized in Table 1. A complete overview of the results can be found in the Supplementary Materials available at the journal’s website (Tables S1–S13 in Appendix 1). In addition, we would like to point out that the majority of results described in the available studies use sheep and cattle as hosts (66%), and consequently, 32% of the results on environmental effects on parasitic nematodes are obtained from just three nematode species: Teladorsagia circumcincta, Trichostrongylus colubriformis, and Haemonchus contortus (Figures 2 and 3).
Table 1. The main findings of the reviewed literature on temperature and moisture effects on life-history traits and behaviour of parasitic nematodes of birds and mammals. For more detailed results, we refer the reader to the corresponding text and the supplementary Tables S1–S13 in Appendix 1


Figure 2. Bar graph of the parasitic nematode species that were used in the reviewed studies on the x-axis. On the y-axis, the number of published results on environmental effects on parasitic nematodes that were obtained from the corresponding parasitic nematode species, ordered from most studied to least studied species.

Figure 3. Bar graph of the host species that were used in the reviewed studies on the x-axis. On the y-axis, the number of published results on environmental effects on parasitic nematodes that were obtained from using the corresponding host species, ordered from most studied to least studied species.
1 What are the effects of temperature and moisture on life-history traits of free-living parasitic nematodes?
1.1 Temperature effects on parasitic nematode life-history traits
Temperature effects (studied in laboratory) on hatching (np = 8, nr = 18, Table S1) (Crofton Reference Crofton1965; Melville et al. Reference Melville, van Dijk, Mitchell, Innocent and Bartley2020), development (np = 14, nr = 29, Table S2) (Ciordia & Bizell Reference Ciordia and Bizell1963; Gyeltshen et al. Reference Gyeltshen, Kahn and Laurenson2022), and survival (np = 15, nr = 19, Table S3) (Gardner et al. Reference Gardner, Gems and Viney2004; Wang et al. Reference Wang, Vineer, Redman, Morosetti, Chen, McFarland, Colwell, Morgan and Gilleard2022) are generally well known. All three traits have an optimum temperature and a minimum and maximum temperature in which the trait functions. Moreover, the temperature effect is dependent on the trait (van Dijk & Morgan Reference van Dijk and Morgan2008; Gyeltshen et al. Reference Gyeltshen, Kahn and Laurenson2022), the species (Crofton, Reference Crofton1965; Gyeltshen et al. Reference Gyeltshen, Kahn and Laurenson2022), larval stage (Peacock et al. Reference Peacock, Kutz, Hoar and Molnár2022), and sometimes substrate (Knapp-Lawitzke et al. Reference Knapp-Lawitzke, von Samson-Himmelstjerna and Demeler2016; Wang et al. Reference Wang, Vineer, Redman, Morosetti, Chen, McFarland, Colwell, Morgan and Gilleard2022). Thus, conclusions on the effect of temperature cannot be drawn based on one trait or stage only, but it is important to consider the different life-history traits, larvae stages, substrates, and the corresponding temperature ranges, simultaneously. However, a minority of the studies (np = 5, nr = 14, Table S1–S3) (Ciordia & Bizell Reference Ciordia and Bizell1963; Gyeltshen et al. Reference Gyeltshen, Kahn and Laurenson2022) cover both the optimum temperature and the upper and lower temperature threshold in which the trait functions.
In addition, some species (e.g., Nematodirus follicollis and Nematodirus battus) require ‘chilling’ before hatching (van Dijk & Morgan Reference van Dijk and Morgan2009; Oliver et al. Reference Oliver, Pomroy, Ganesh and Leathwick2016) and/or show higher hatching rates after chilling (van Dijk & Morgan Reference van Dijk and Morgan2008). Some parasitic nematode species develop to infective larvae within the eggs before they hatch. Interestingly, within this group of nematodes there are species that need a minimum of ‘chilling units’ before hatching can occur, as, for example, in Nematodirus filicollis (Oliver et al. Reference Oliver, Pomroy, Ganesh and Leathwick2016). Chilling experiments aim to study these specific chilling requirements, (e.g., specific chilling temperature and chilling duration). Temperature could also impact developmental paths, for instance, to the infective larval stage or adult stage (np = 4, nr = 4, Table S4) (Viney Reference Viney1996; Aleuy et al. Reference Aleuy, Hoberg, Paquette, Ruckstuhl and Kutz2019).
Most laboratory studies keep each temperature treatment level constant over time (all above mentioned and Table S1–S4). However, the effects of fluctuating temperatures (which is more representative of the natural environment) on life-history traits yield different, but nonuniversal, outcomes compared to constant temperatures (np = 5, nr = 6, Table S5) (Saunders et al. Reference Saunders, Tompkins and Hudson2000b; Hernandez et al. Reference Hernandez, Poole and Cattadori2013) and thus require more attention. Also, only a limited number of studies were conducted in the field with contrasting climatic conditions (np = 2, nr = 3, Table S6) (Hoar Reference Hoar2012; Hernandez et al. Reference Hernandez, Poole and Cattadori2013). These studies confirmed a temperature threshold of development of a parasitic nematode in the lower central Canadian Arctic (Hoar Reference Hoar2012) and a positive effect of thermal energy accumulation on the recovery of infective L3 larvae from faeces in Scotland with a temperate climate (Hernandez et al. Reference Hernandez, Poole and Cattadori2013). Since recovery was not specified in this last study, we assume Hernandez et al. (Reference Hernandez, Poole and Cattadori2013) used recovery as a proxy for the result of hatching, development, and survival combined.
1.2 Precipitation and moisture effects on parasitic nematode life-history traits
The effect of moisture on life-history traits under laboratory conditions (np = 10, nr = 29, Table S7) (Rossanigo & Gruner Reference Rossanigo and Gruner1995; Gyeltshen et al. Reference Gyeltshen, Kahn and Laurenson2022) has been less extensively studied compared with the effect of temperature. Nonetheless, these studies showed positive effects of simulated rainfall (O’Connor et al. Reference O’Connor, Kahn and Walkden-Brown2007; Khadijah, Kahn, Walkden-Brown et al. Reference Khadijah, Kahn, Walkden-Brown, Bailey and Bowers2013), faecal moisture content (FMC) /soil moisture (O’Connor et al. Reference O’Connor, Kahn and Walkden-Brown2007; Gyeltshen et al. Reference Gyeltshen, Kahn and Laurenson2022), and the ratio of precipitation to evaporation rates (O’Connor et al. Reference O’Connor, Kahn and Walkden-Brown2007) on development and recovery rate (here used as proxy for developmental success) of L3 larvae from soil. FMC was also positively correlated with body length of L3 larvae (of four different nematode species; Rossanigo & Gruner, Reference Rossanigo and Gruner1996). Larvae recovery was also affected by simulated rainfall timing (Khadijah, Kahn, Walkden-Brown et al. Reference Khadijah, Kahn, Walkden-Brown, Bailey and Bowers2013a, Reference Khadijah, Kahn, Walkden-Brown, Bailey and Bowers2013b) but in opposite ways according to the distribution (split vs. single) of simulated rain (O’Connor et al. Reference O’Connor, Kahn and Walkden-Brown2007, Reference O’Connor, Kahn and Walkden-Brown2008). Low relative humidity resulted in high mortality of eggs of Nematodirus battus (van Dijk & Morgan Reference van Dijk and Morgan2012), but drought stress had no significant impact on the recovery of L3 larvae (Cooperia oncophora) from soil or grass (proxy for survival; Knapp-Lawitzke et al. Reference Knapp-Lawitzke, von Samson-Himmelstjerna and Demeler2016). As with temperature, only limited studies were conducted in the field (np = 3, nr = 4, Table S8) (Khadijah, Kahn, Walkden-Brown et al. Reference Khadijah, Kahn, Walkden-Brown, Bailey and Bowers2013c; May et al. Reference May, Raue, Blazejak, Jordan and Strube2022). These field studies showed differing results but were also conducted in the field under different climatic conditions. Soil moisture was identified as important for translation (a term used by the authors to describe the process of development and transition from egg to parasite stage within the host) of parasitic nematodes in sheep in New South Wales (NSW), Australia (Khadijah, Kahn, Walkden-Brown et al. Reference Khadijah, Kahn, Walkden-Brown, Bailey and Bowers2013c). Nonetheless, wetting of pasture did not have long-term effects on infection of endoparasites (including nematodes) in sheep and cattle (measured by larvae and egg count in faeces) in Germany (May et al. Reference May, Raue, Blazejak, Jordan and Strube2022). In addition, a field study in Texas showed that viability of eggs (of Baylisascaris procyonis that can have both direct and indirect life cycle with racoon as host) was not related to soil texture, moisture, and sun exposure (Ogdee et al. Reference Ogdee, Henke, Wester and Fedynich2016).
1.3 Interacting temperature and moisture effects on parasitic nematode life-history traits
Only two studies (np = 2, nr = 5, Table S9) (Rossanigo & Gruner Reference Rossanigo and Gruner1995; McCarthy et al. Reference McCarthy, Vineer, Morgan and van Dijk2022) tested for an explicit interaction effect between temperature and moisture, and neither found one. McCarthy et al. (Reference McCarthy, Vineer, Morgan and van Dijk2022) found that lungworm (Dictyocaulus viviparus) L3 larvae mortality was similar in paired wet and dry conditions measured at three different temperatures; so, by inference, the effect of moisture on L3 larvae mortality did not depend on temperature. A study of strongyles in sheep, cattle, and deer found that the optimal faecal moisture content for development was independent of temperature (Rossanigo & Gruner Reference Rossanigo and Gruner1995). Thus, a limited number of studies report a lack of interacting abiotic factors on life-history traits of parasitic nematodes. Three other studies focused on the combination or additive effects of abiotic factors on life-history traits without testing for possible interactions (Table S2, S3, S7; Rossanigo & Gruner Reference Rossanigo and Gruner1996; Knapp-Lawitzke et al. Reference Knapp-Lawitzke, von Samson-Himmelstjerna and Demeler2016; Gyeltshen et al. Reference Gyeltshen, Kahn and Laurenson2022). For instance, an extensive laboratory study on the effect of temperature and faecal water content (FWC) on development averaged FWC across the different temperatures, instead of considering the interaction between FWC and temperature, and merely suggested that temperature negatively affected FWC (Gyeltshen et al. Reference Gyeltshen, Kahn and Laurenson2022). Rossanigo & Gruner (Reference Rossanigo and Gruner1996) found that L3 larvae body size increased with faecal moisture content (FMC) in all three temperature regimes in four species of strongyles, but apparent interacting effects between temperature and moisture (visual assessment of their Figure 1) were not formally tested. Finally, a study of temperature (spring versus summer scenarios), drought (adequate water versus none), and exposure duration on the overall recovery of L3 larvae (proxy for survival) showed negative effects of temperature (on recovery from soil) and exposure duration (on recovery from grass), but possible interactions were not tested (Knapp-Lawitzke et al. Reference Knapp-Lawitzke, von Samson-Himmelstjerna and Demeler2016). Thus, more information on interacting factors might exist in the present literature, and this data could possibly be acquired to analyze interacting factors specifically.
2 What are the effects of temperature and moisture on the behaviour of free-living parasitic nematodes?
2.1 Temperature effects on parasitic nematode behaviour
The effect of temperature on parasite behaviour and movement (out of faeces and in soil) is not conclusive (np = 6, nr = 8, Table S10) (Saunders et al. Reference Saunders, Tompkins and Hudson2000a; Gyeltshen et al. Reference Gyeltshen, Kahn and Laurenson2022). For example, some laboratory studies showed migration declined over time (days) stored at a constant high temperature of 30°C (Hamilton et al. Reference Hamilton, Waghorn, de Waal, Keane, Green and Leathwick2022) and an optimum temperature for L3 larvae migration out of faeces (Gyeltshen et al. Reference Gyeltshen, Kahn and Laurenson2022). However, temperature did not affect migration out of faeces in a greenhouse setting (Wang et al. Reference Wang, Vineer, Morrison, van Wyk, Bolajoko, Bartley and Morgan2018) and had no significant effect (but a positive trend) on vertical migration of L3 larvae in soil (Knapp-Lawitzke et al. Reference Knapp-Lawitzke, von Samson-Himmelstjerna and Demeler2016). Moreover, the effect of temperature on parasite behaviour is complex, as temperature might interact with light and vegetation type (Saunders et al. Reference Saunders, Tompkins and Hudson2000a). Studies under laboratory conditions conclusively indicated that temperature is important for thermokinetic behaviour and olfactory responses of skin-penetrating nematodes (np = 10, nr = 15, Table S11) (Stankiewicz Reference Stankiewicz1996; Dulovic et al. Reference Dulovic, Norman, Harbecke and Streit2022; and for a more extensive review, see Bryant & Hallem Reference Bryant and Hallem2018; Mendez et al. Reference Mendez, Walsh and Hallem2022; Takeishi Reference Takeishi2022). We could not find field studies on the effect of temperature on parasitic nematode behaviour.
2.2 Precipitation and moisture effects on parasitic nematode behaviour
Studies under laboratory or greenhouse conditions (np = 7, nr = 19, Table S12) (Rossanigo & Gruner Reference Rossanigo and Gruner1996; Gyeltshen et al. Reference Gyeltshen, Kahn and Laurenson2022) showed that water is required for infective larvae to migrate out of faeces (van Dijk & Morgan Reference van Dijk and Morgan2011; Wang et al. Reference Wang, van Wyk, Morrison and Morgan2014), with both a minimum and optimum faecal water content (FWC) value for migration of infective larvae out of faeces (Gyeltshen et al. Reference Gyeltshen, Kahn and Laurenson2022). Similarly, there was increased migration under increasing simulated rainfall, again with a required minimum for migration to occur (Wang et al. Reference Wang, Vineer, Redman, Morosetti, Chen, McFarland, Colwell, Morgan and Gilleard2022). In contrast to migration out of a faecal pat, it has been suggested that parasitic nematodes do not need water to move from the soil surface into the herbage (van Dijk & Morgan Reference van Dijk and Morgan2011). Simulated rainfall and evaporation also affected migration of L3 larvae to deeper soil layers. Recovery of L3 larvae in deeper soil layers increased with lower evaporation rates, with increasing simulated rainfall, and over time (O’Connor et al. Reference O’Connor, Kahn and Walkden-Brown2008). In contrast, drought stress had no significant effect (but a negative trend) on L3 larvae migration from grass to soil (Knapp-Lawitzke et al. Reference Knapp-Lawitzke, von Samson-Himmelstjerna and Demeler2016). Moreover, it was suggested that migration can be mediated by individual differences in body length. Short L3 larvae (collected from dry faeces) migrated more slowly than long L3 larvae collected from wet faeces (Rossanigo & Gruner Reference Rossanigo and Gruner1996). There is only one field study on the effect of rain and moisture on the behaviour of parasitic nematodes in Texas (np = 1, nr = 1, Table S13) (Ogdee et al. Reference Ogdee, Henke, Wester and Fedynich2016). This study indicated no (passive) movement of nematode eggs on dry soils and minor movement on wet soils (dependent on the soil type but remained within the top 10 cm in the soil column) over the course of two years (Ogdee et al. Reference Ogdee, Henke, Wester and Fedynich2016).
2.3 Interacting temperature and moisture effects on parasitic nematode behaviour
None of the reviewed studies explicitly tested for an interaction effect of temperature and moisture on parasitic nematode behaviour. In the discussion we will elaborate on three studies (also described in paragraph 1.3) that included a combined effect of moisture and temperature and thus had a study design capable of testing for possible interacting effects, but which were not explicitly done (Table S10, S12; Rossanigo & Gruner Reference Rossanigo and Gruner1996; Knapp-Lawitzke et al. Reference Knapp-Lawitzke, von Samson-Himmelstjerna and Demeler2016; Gyeltshen et al. Reference Gyeltshen, Kahn and Laurenson2022).
Discussion
In this review, we identified the knowledge gaps in research on abiotic effects on terrestrial parasitic nematodes in birds and mammals (Figure 4). While much is known about the separate effects of temperature and moisture on parasite life history (Figure 4, lines 1a, 1b), knowledge about the interactive effects of both temperature and moisture on parasitic nematode life-history traits is scarce (Figure 4, line 1c) even though this has long been recognised as important (see O’ Connor et al. Reference O’ Connor, Walkden-Brown and Kahn2006). We highlight this as the first major knowledge gap, especially given recent climate change (IPCC Reference Masson-Delmotte, Zhai, Pirani, Connors, Péan, Berger, Caud, Chen, Goldfarb, Gomis, Huang, Leitzell, Lonnoy, Matthews, Maycock, Waterfield, Yelekçi and Yu2021). Although positive moisture effects have been documented on parasite motility (Figure 4, line 2b), there is little known about temperature effects (Figure 4, line 2a) or the interaction of moisture and temperature (Figure 4, line 2c). This is the second major gap and is important because these interactions could influence transmission into the host (Silva et al. Reference Silva, Amarante, Kadri, Carrijo-Mauad and Amarante2008). Also, we advocate for more experimental field studies that test the effects of multiple environmental factors on life-history traits and behaviour under more natural and realistic circumstances. Moreover, we encourage studies to expand the range of used hosts and parasitic nematodes. Future studies targeting these knowledge gaps should improve our understanding of how environmental change will affect parasitic nematodes and, consequently, influence parasite–host dynamics. Such research has broader implications for identifying infection risks, which is highly relevant to livestock managers and wildlife conservationists, and for an improved understanding of ecosystem functioning in a rapidly warming climate.
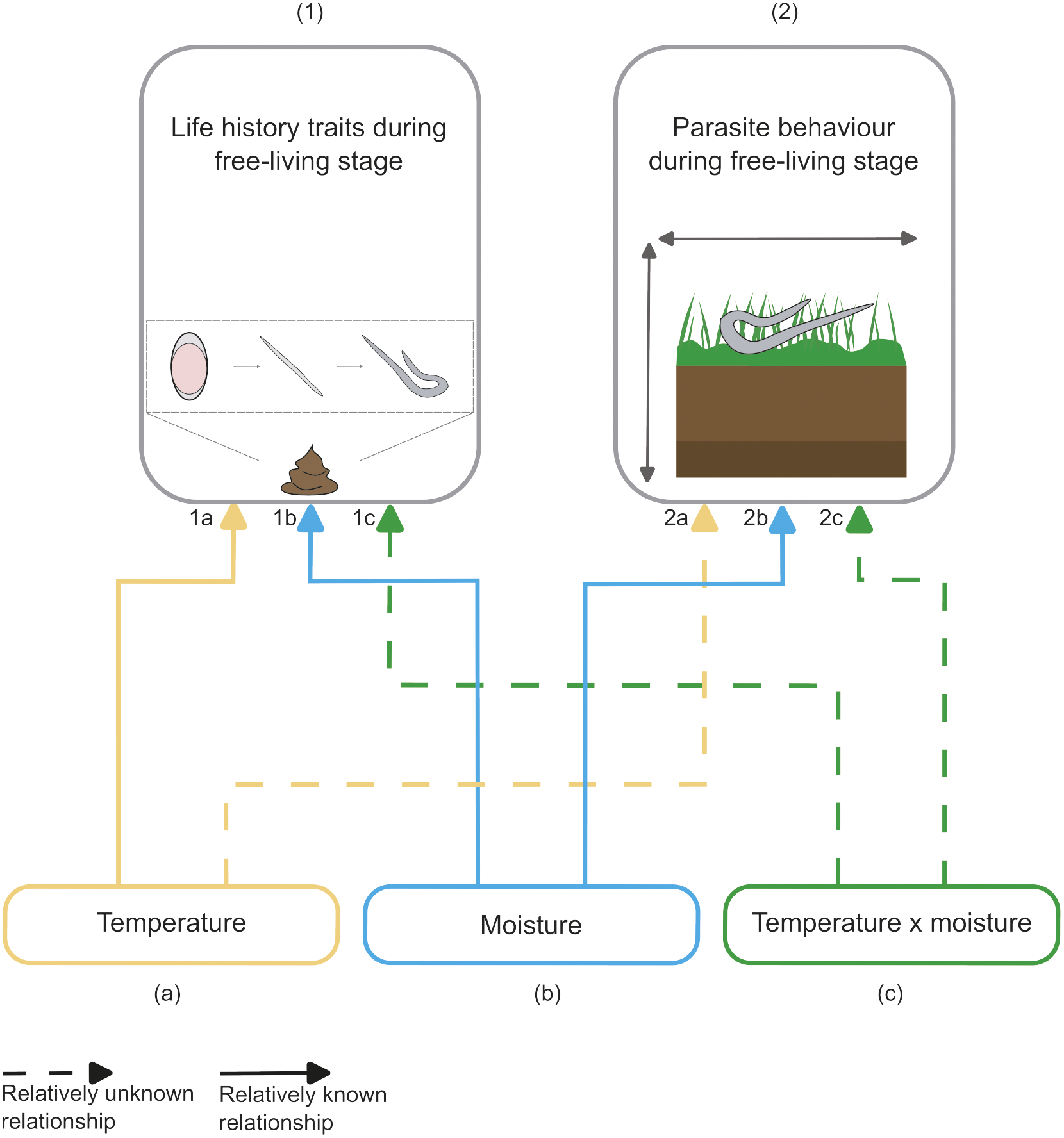
Figure 4. Visualization of the relatively known (solid arrows) and relatively unknown (dashed arrows) effects of temperature (yellow, a), moisture (blue, b), and temperature and moisture combined (green, c) on 1) life-history traits and 2) behaviour of parasitic nematodes of birds and mammals during the free-living stages.
1 Parasitic nematode life-history traits
Although temperature effects on hatching, development, and survival are generally well known (Figure 4, line 1a), we wish to encourage future research to focus on three issues. First, additional studies should calculate complete temperature dependency curves for all the different vital rates of a variety of species (see, for example, van Dijk & Morgan Reference van Dijk and Morgan2009). Insight into the full range of temperature dependency curves could be used for projecting parasite–host dynamics under changing climates with mathematical models (Fox et al. Reference Fox, Marion, Davidson, White and Hutchings2012; Molnár et al. Reference Molnár, Sckrabulis, Altman and Raffel2017). Determining temperature dependency curves of different life-history rates will also allow the search for general patterns within and across species (Vineer et al. Reference Vineer, Steiner, Knapp-Lawitzke, Bull, Fernex, Bosco, Hertzberg, Demeler, Rinaldi, Morrison, Skuce, Bartley and Morgan2016; Phillips et al. Reference Phillips, Vargas Soto, Pawar, Koprivnikar, Benesh and Molnár2022). Discovering general patterns (by using meta-analysis) would provide insight into the reliability of translating findings to different study systems. Second, future studies should investigate how fluctuating temperatures, more representative of the increased variance observed under climate warming (IPCC 2021), affect life-history traits – therefore better representing natural circumstances. Third, studies should test which species require pre-hatch chilling and how a warming climate will affect and possibly reduce hatching rate of species that require chilling. For example, warming could delay accumulation of ‘chilling units’, which could delay hatching by one year (Oliver et al. Reference Oliver, Pomroy, Ganesh and Leathwick2016), with possible consequences for infectivity patterns. Thus, although temperature effects on life-history traits are generally well known, specific knowledge is still needed.
Most of the studies we reviewed that tested effects of moisture focused on the overall recovery of L3 larvae under different moisture conditions (Figure 4, line 1b). However, measuring total recovery of L3 larvae from faeces or soil is sometimes used as a proxy for developmental success (O’Connor et al. Reference O’Connor, Kahn and Walkden-Brown2007) or survival (Knapp-Lawitzke et al. Reference Knapp-Lawitzke, von Samson-Himmelstjerna and Demeler2016), or is not specified (Hernandez et al. Reference Hernandez, Poole and Cattadori2013). Nonetheless, true recovery is composed of hatching, development, larval survival, and possibly motility. This means that the effects of rain and moisture on the separate rates of hatching, development, and survival are still poorly known. To disentangle this, (laboratory) experiments are needed that sample frequently and differentiate between the different life stages and between dead and live larvae to separate survival and migration processes (for instance, by using extracting methods that are not based on motility and counting living and dead individuals). Studying the moisture response curve for separate instead of composite processes would also improve predictive models as advocated by Molnár and colleagues for thermal response curves (Molnár et al. Reference Molnár, Sckrabulis, Altman and Raffel2017).
The effect of interacting environmental factors on parasitic nematodes is identified as an important knowledge gap because it is more representative of variation in the natural environment than the effect of the separate abiotic factors in isolation (Figure 4, line 1c). In addition, under the current climate change projections, more extreme weather events are expected (IPCC 2021), and therefore, experiments that test interacting effects of moisture and temperature across large gradients, even beyond the current norm, are increasingly valuable. Only two studies formally investigated the possible interaction between temperature and moisture and indicated an absence of interactive effect of temperature and moisture on parasite life history (Rossanigo & Gruner Reference Rossanigo and Gruner1995; McCarthy et al. Reference McCarthy, Vineer, Morgan and van Dijk2022). Among three other studies which focused on additive effects of abiotic factors on life-history traits without testing for possible interactions (e.g., Rossanigo & Gruner Reference Rossanigo and Gruner1996; Knapp-Lawitzke et al. Reference Knapp-Lawitzke, von Samson-Himmelstjerna and Demeler2016; Gyeltshen et al. Reference Gyeltshen, Kahn and Laurenson2022), one presented a figure suggesting apparent interactions (Figure 1 in Rossanigo & Gruner Reference Rossanigo and Gruner1996). When reanalysing the data provided in their Table 2, there is a statistically significant interaction between moisture and temperature on L3 larvae body size in all four species studied: the temperature regime leading to the largest larvae differed as faecal moisture content varied from 30 to 80%, reflecting that optimal development temperatures are modulated by moisture (Supplementary Materials – Appendix 2). Testing for interactions among multiple environmental factors (Rossanigo & Gruner Reference Rossanigo and Gruner1995; Tarbiat et al. Reference Tarbiat, Rahimian, Jansson, Halvarsson and Höglund2018; Shifaw et al. Reference Shifaw, Feyera, Elliott, Sharpe, Ruhnke and Walkden-Brown2022) will advance our understanding of parasite–host interactions under increasingly variable conditions.
2 Parasitic nematode behaviour
Parasite motility influences contact rates between parasite and host, and hence potentially transmission rates. Also, parasite motility could modulate the effect of environmental change on life-history traits, by, for instance, the ability to ‘escape’ adverse environments. Nevertheless, there is limited knowledge on how temperature alone, or in combination with moisture, affect behaviour, especially the vertical migration of parasitic nematodes (Figure 4, lines 2a, c). Therefore, we advocate studies on temperature, moisture, and their combined effects on parasite behaviour and highlight two specific angles. First, how does temperature (Figure 4, line 2a) and soil moisture (Figure 4, line 2b), and their interaction (Figure 4, line 2c), affect vertical migration of parasitic nematodes in vegetation and in soil, especially under natural conditions? For instance, an entomopathogenic nematode (infecting insects) that poorly tolerates desiccation migrated towards the bottom soil layer with decreasing moisture content, while another species of nematode that is desiccation tolerant remained in the top layer (Salame & Glazer Reference Salame and Glazer2015). Whether parasitic nematodes of birds and mammals show similar migration behaviour is unknown. In addition, parasitic nematodes face a trade-off between avoiding adverse environmental conditions and opportunities enhancing the probability of being ingested by the host. For example, L3 larvae recovered from soil were considered fitter (measured with migration assay) than the individuals recovered from the grass (Knapp-Lawitzke et al. Reference Knapp-Lawitzke, von Samson-Himmelstjerna and Demeler2016). Migration behaviour might also vary between individuals with, for instance, longer nematodes (grown at optimal conditions), migrating more than shorter individuals and possibly increasing infection probability (Rossanigo & Gruner Reference Rossanigo and Gruner1996). In addition, insight is needed on how parasitic nematodes are distributed among different vegetation types and possible interactions with abiotic factors (Saunders et al. Reference Saunders, Tompkins and Hudson2000a). These insights could be provided by experiments that test vertical migration under different environmental factors on different vegetation types. Second, how do temperature and moisture levels affect the distance of horizontal dispersal (Figure 4, lines 2a, b, c), and do parasitic nematodes predominantly disperse actively or passively (for instance, rain splashes might be a major factor for translocation; Grønvold & Høgh-Schmidt Reference Grønvold and Høgh-Schmidt1989)? We advocate experiments that test dispersal range away from faeces under various environmental factors, including extreme temperature and moisture levels. Although rainfall is required for migration out of faeces (van Dijk & Morgan Reference van Dijk and Morgan2011; Wang et al. Reference Wang, van Wyk, Morrison and Morgan2014), heavy precipitation could also have negative effects. For instance, irrigation of the pasture facilitated migration of larvae, but mortality was high, and fewer larvae were recovered from the herbage on irrigated plots compared to dry plots, possibly due to mortality or dispersal to wider areas (Young & Anderson Reference Young and Anderson1981). Studying horizontal dispersal distances is especially relevant in relation to faecal avoidance by hosts (Hutchings et al. Reference Hutchings, Kyriazakis, Anderson, Gordon and Coop1998; van der Wal et al. Reference van der Wal, Irvine, Stien, Shepherd and Albon2000). Understanding the factors that affect both vertical and horizontal movement and linking this to the contact place with the host will help in understanding transmission patterns and infection risk of the host. In addition, the availability of vegetation (in relation to environmental conditions) is potentially an important factor in understanding the link between contact rates with the host and transmission. For example, vegetation growth (due to high levels of rainfall) could lead to a dilution effect with a decreased density of nematodes in the environment and possibly result in fewer encounters with parasites by the host (Grenfell Reference Grenfell1988; Grenfell Reference Grenfell1992; Shearer & Ezenwa Reference Shearer and Ezenwa2020).
3 Translation from laboratory to field conditions
We advocate more field studies, in addition to laboratory studies, because microclimate differences and soil structure might alter the relationships between environmental factors and parasites only documented under laboratory conditions. For example, solar radiation might cause mortality at temperatures lower than the threshold temperature determined in the laboratory (van Dijk et al. Reference van Dijk, de Louw, Kalis and Morgan2009), and snow layers could benefit parasite survival (Rossi et al. Reference Rossi, Interisano, Deksne and Pozio2019). Moreover, air temperature might be an insufficient proxy for temperature conditions near or in soil (Lembrechts et al. Reference Lembrechts, Hoogen, Aalto, Ashcroft, de Frenne, Kemppinen, Kopecký, Luoto, Maclean, Crowther, Bailey, Haesen, Klinges, Niittynen, Scheffers, van Meerbeek, Aartsma, Abdalaze, Abedi and Lenoir2022), especially during times with snow cover (Convey et al. Reference Convey, Abbandonato, Bergan, Beumer, Biersma, Bråthen, D’Imperio, Jensen, Nilsen, Paquin, Stenkewitz, Svoen, Winkler, Müller and Coulson2015). Surface or soil temperature might be more important and could have different effects on life-history traits of nematodes (Jenkins et al. Reference Jenkins, Veitch, Kutz, Hoberg and Polley2006; Kutz et al. Reference Kutz, Hoberg, Molnár, Dobson and Verocai2014). Also, in the field, biotic interactions may affect parasite life history and behaviour in several ways. For example, the presence of earthworms can reduce the number of recovered larvae in the field (Waghorn et al. Reference Waghorn, Reynecke, Oliver, Miller, Vlassoff, Koolaard and Leathwick2011). The presence of nemathophagous fungus was associated with a reduction in the number of infective parasite larvae (Kuzmina et al. Reference Kuzmina, Kuzmin and Kharchenko2006), but fungus can also play a role in and facilitate migration of parasitic larvae (McCarthy et al. Reference McCarthy, Vineer, Morgan and van Dijk2022). Moreover, in some species, contact between bacteria and embryonated eggs is required for hatching within the host (Hayes et al. Reference Hayes, Bancroft, Goldrick, Portsmouth, Roberts and Grencis2010). These biotic interactions add complexity beyond simple relationships between weather variables and parasite life-history traits and behaviour. Thus, field experiments and laboratory experiments are complementary. Lab experiments will continue to provide mechanistic links between the environment and parasite traits, but they need to be scrutinized in real-world settings to investigate if relationships hold. By doing so, we can more reliably draw conclusions on how changing climates affect parasites and hence their interaction with hosts.
4 Expanding the range of parasitic nematode and host species studies
In addition, we would like to stress that the majority of results described in the available studies use sheep and cattle as hosts (66%), and 32% of results describing environmental effects on parasitic nematodes are obtained from just the three nematode species: Teladorsagia circumcincta, Trichostrongylus colubriformis, and Haemonchus contortus (Figures 2 and 3). These species are the most abundant gastrointestinal nematodes in sheep and cause major production losses (O’Connor et al. Reference O’ Connor, Walkden-Brown and Kahn2006). However, we envisage that the nematode–host interaction could be different for livestock and free-living hosts. For example, wild animals generally live in lower population densities and do not receive (anthelmintic) treatments or supplemental food. Also, parasitic nematodes of livestock hosts might encounter different selection pressures due to rotational grazing or other treatments, which might result in differences in environmental effects on parasitic nematodes of wild compared to livestock animals. Because there is limited data on wildlife hosts and their parasitic nematodes, we encourage future studies on the parasitic nematodes of wild host species under natural conditions. Moreover, few of the reviewed studies involve the environmental effects on parasitic nematodes infecting humans. However, infection of soil-transmitted helminths in humans is a major cause of human disease (Brooker et al. Reference Brooker, Clements and Bundy2006; Weaver et al. Reference Weaver, Hawdon and Hoberg2010), especially in places where sanitation facilities are limited but population densities are high (Brooker et al. Reference Brooker, Clements and Bundy2006; Weaver et al. Reference Weaver, Hawdon and Hoberg2010). To limit infection by soil-transmitted helminths, insights are needed on how these parasites are affected by environmental changes (Weaver et al. Reference Weaver, Hawdon and Hoberg2010).
5 Link to life stages within the host and parasite host dynamics
The scope of this review was to identify the knowledge gaps in research about the abiotic factors impacting hatching, development, survival, and behaviour of direct life-cycle parasitic nematodes in their free-living stages. However, we have not considered how environmental factors experienced in these free-living stages might affect parasites once they are within the host – for example, when they need to exsheath, develop, survive, and reproduce (Figure 1) (Kutz et al. Reference Kutz, Jenkins, Veitch, Ducrocq, Polley, Elkin and Lair2009; Morley & Lewis Reference Morley and Lewis2014; Cizauskas et al. Reference Cizauskas, Carlson, Burgio, Clements, Dougherty, Harris and Phillips2017). Also, environmental factors can affect parasitic nematodes during the stages within the host through effects on homeostasis of the host, for instance (see for a review, but not specifically on parasitic nematodes, Morley & Lewis Reference Morley and Lewis2014). It is also suggested that rainfall could affect parasite infection levels through changes in host susceptibility, with low rainfall levels (compared to high rainfall levels) increasing susceptibility of the host to parasite infection (Shearer & Ezenwa Reference Shearer and Ezenwa2020). This could possibly be explained by reduced food availability and quality under low rainfall conditions, and hence changes and reduction in body condition of the host, but this mechanism needs to be tested (Shearer & Ezenwa Reference Shearer and Ezenwa2020). Also, we need to consider the abilities for adaptation to changing conditions by the host and by the parasites (reviewed by Aleuy & Kutz Reference Aleuy and Kutz2020) to get a full comprehension of the environmental effects on parasitic nematode.
6 Implications
In this review, we have focused attention on understudied abiotic impacts that could improve our insights into how environmental factors affect parasitic nematodes in their free-living stages, and hence the likely impact of climate change. Addressing these knowledge gaps will also have implications for identifying infection risks, of high relevance to livestock managers and wildlife conservationists, as well as improve our understanding of ecosystem functioning under a rapidly warming climate. First, experiments on the temperature and moisture (and their interaction) dependency curves of parasitic life-history traits and behaviour could better guide livestock managers about parasite dynamics and epidemiology (considered as an important knowledge gap according to the research community in livestock helminthology; Morgan et al. Reference Morgan, Aziz, Blanchard, Charlier, Charvet, Claerebout, Geldhof, Greer, Hertzberg, Hodgkinson, Höglund, Hoste, Kaplan, Martínez-Valladares, Mitchell, Ploeger, Rinaldi, von Samson-Himmelstjerna, Sotiraki and Vercruysse2019) and infection risks under changing climates. For instance, improved knowledge on how abiotic factors influence parasite life history and behaviour can help improve models that project infection pressure under various climate change scenarios (Rose et al. Reference Rose, Caminade, Bolajoko, Phelan, van Dijk, Baylis, Williams and Morgan2016). Moreover, data from these experiments could refine infection risk maps and calendars estimating when and where infection risks are high (Navarre Reference Navarre2020; McFarland et al. Reference McFarland, Rose Vineer, Chesney, Henry, Brown, Airs, Nicholson, Scollan, Lively, Kyriazakis and Morgan2022) and provide insights about pasture management strategies (Morgan et al. Reference Morgan, Aziz, Blanchard, Charlier, Charvet, Claerebout, Geldhof, Greer, Hertzberg, Hodgkinson, Höglund, Hoste, Kaplan, Martínez-Valladares, Mitchell, Ploeger, Rinaldi, von Samson-Himmelstjerna, Sotiraki and Vercruysse2019, Navarre Reference Navarre2020), such as fencing off risk areas and drainage (Beltrame et al. Reference Beltrame, Rose Vineer, Walker, Morgan, Vickerman and Wagener2021). For instance, experiments on the effect of temperature and moisture (and their interaction) on the vertical and horizontal distribution of parasitic nematodes could provide information on infection hotspots in the herbage and might guide management of forage heights (Navarre Reference Navarre2020). Second, knowledge (obtained by additional experiments on temperature and moisture effects on parasites) could provide insights benefiting wildlife conservation, such as disease risk frameworks and optimal timing of intervention, particularly in areas with a co-existence of livestock and wildlife (Khanyari et al. Reference Khanyari, Milner-Gulland, Oyanedel, Vineer, Singh, Robinson, Salemgareyev and Morgan2022). Moreover, the presence of parasites might be, in some cases, beneficial to population health (Thompson et al. Reference Thompson, Lymbery and Smith2010). For instance, parasites could possibly act on delayed density dependent mechanisms, which could dampen host population fluctuations and hinder population crashes resulting from rapid increases. Thus, understanding environmental effects on parasites and if and how parasite species should be conserved (Thomas et al. Reference Thomas, Bonsall, Dobson, Thomas, Renaud and Guegan2005) will have indirect benefits for wildlife and ecosystem conservation. Third, knowledge about environmental effects on parasitic nematodes (based on experiments that test the effect of a broad range of moisture and temperature regimes on life-history traits and behaviour of a wide range of parasites) might improve the understanding of ecosystem functioning. Parasites play a role in ecosystem engineering processes, either by modifying hosts that are ecosystem engineers or as engineers themselves (Thomas et al. Reference Thomas, Poulin, de Meeüs, Guégan and and Renaud1999). They can also impact intraspecific species interaction (Hudson et al. Reference Hudson, Cattadori, Boag and Dobson2006) such as predator–prey interactions (Hudson et al. Reference Hudson, Dobson and Newborn1992; Hatcher et al. Reference Hatcher, Dick and Dunn2006) and hence energy flow through the system (Hudson et al. Reference Hudson, Cattadori, Boag and Dobson2006). Understanding which functions in the ecosystem will be lost if a parasite species disappears might result in the realization that parasite species need to be conserved in order to maintain an ecosystem and its functions (Thomas et al. Reference Thomas, Bonsall, Dobson, Thomas, Renaud and Guegan2005). Parasites could also cause cascading effects on other (trophic) species in the ecosystem (Thomas et al. Reference Thomas, Bonsall, Dobson, Thomas, Renaud and Guegan2005). For instance, it was suggested that lethal and sub-lethal effects of a parasitic nematode on an herbivorous host could result in trophic cascades (in terms of lower herbivore host biomass and increased producer biomass) (Koltz et al. Reference Koltz, Civitello, Becker, Deem, Classen, Barton and Ezenwa2022). Thus, changes in the environmental effects on parasitic nematodes might have larger consequences on the ecosystem than just on the parasite and host. In conclusion, addressing and hence filling the knowledge gaps about abiotic impacts on parasitic nematodes could have broad implications for livestock managers and wildlife conservationists and improve our fundamental understanding of parasitic nematodes and ecosystem functioning under a rapidly warming climate.
Supplementary material
The supplementary material for this article can be found at http://doi.org/10.1017/S0022149X23000652.
Acknowledgements
The authors would like to acknowledge the NMBU library staff for their help developing appropriate search terms for this literature review.
Financial support
This work was supported by the Research Council of Norway (project number 315454) and a PhD grant to TMM provided by NMBU.
Competing interest
The authors declare none.
Ethical standard
This study did not involve human and/or animal experimentation.