Introduction
Among Ti4+-, Sn4+-, VIGe4+-, VISi-, VIMn4+-, VIPb4+-, VITe4+-, Nb-, Ta-, Sb5+-, Mo6+- and W6+-oxide minerals with the stoichiometry MO2, there are numerous mineral species related structurally to columbite. Although they display substantial common features, these minerals differ from each other in many aspects, including different kinds of cation ordering, symmetry, unit-cell dimensions, and coordination numbers of cations. Attempts to elaborate a general crystal-chemical classification of columbite-type minerals and other related mineral species with the stoichiometry MO2 have been undertaken repeatedly (Graham and Thornber, Reference Graham and Thornber1974a; Sugitani et al., Reference Sugitani, Suzuki and Nagashima1985; Hanson et al., Reference Hanson, Simmons, Falster, Foord and Lichte1999). This paper summarises available data on minerals with the stoichiometry MO2 that are topologically related to columbite and constitute the columbite supergroup. The nomenclature and classification of the columbite supergroup has been approved by the Commission on New Minerals, Nomenclature and Classification of the International Mineralogical Association (IMA–CNMNC).
The root-name columbite is the oldest one among all of the names of mineral species that are discussed in this nomenclature report. Minerals belonging to the columbite group are important from petrological, geochemical and practical points of view.
The name is after the chemical composition: the mineral columbite was described originally as an iron and columbium oxide. Columbium is an old and today obsolete name for the chemical element that was later re-named niobium. The mineral, however, retained its name. The root columbium is also maintained in ‘coltan’, an acronym which refers to the niobium/tantalum oxides.
General definitions
The following criteria are applied to define the minerals of the columbite supergroup:
(1) The general stoichiometry MO2 is required.
(2) The crystal structure is based on the hexagonal close packing (hcp) of anions (or close to it).
(3) Only octahedral voids of hcp are occupied. As a result, the coordination number of M-type cations is 6 (sometimes augmented to 8 in the case of a slight distortion of hcp).
(4) The presence of zig-zag chains of edge-shared octahedra (the idealised symmetry described by the rod group þ2/c11; Fig. 1).

Fig. 1. The zig-zag and straight chains of edge-shared MO6 octahedra and their rod groups.
The application of these criteria obviously excludes compounds with rutile-related structures (e.g. tapiolite-group minerals) which are characterised by straight chains of edge-sharing octahedra with the idealised symmetry described by the rod group þ112/m (Fig. 1). Alumotantite (Ercit et al., Reference Ercit, Hawthorne and Černý1992d) only matches criteria I-III and is not considered a member of the columbite supergroup. A short outline of minerals with the general MO2 stoichiometry which do not belong to the columbite supergroup (and as such were not part of the IMA-approved report) has been deposited with the Principal Editor of Mineralogical Magazine and is available as Supplementary material (see below).
Using the approach applied for the perovskite supergroup (Mitchell et al., Reference Mitchell, Welch and Chakhmouradian2017), the ixiolite-type structure is considered as an aristotype with the space group Pbcn, the smallest unit cell volume and the basic vectors a0, b0 and c0. The following derivatives can be distinguished on the basis of the multiplying of the initial ixiolite-type unit cell (Fig. 2a): ixiolite type with a = a0, b = b0 and c = c0; space group Pbcn; wolframite type (an ordered analogue of the ixiolite type) with a = a0, b = b0 and c = c0; P2/c; samarskite type with a = 2a0, b = b0 and c = c0; P2/c; columbite type with a = 3a0, b = b0 and c = c0; Pbcn; and wodginite type with a = 2a0, b = 2b0 and c = c0; C2/c.
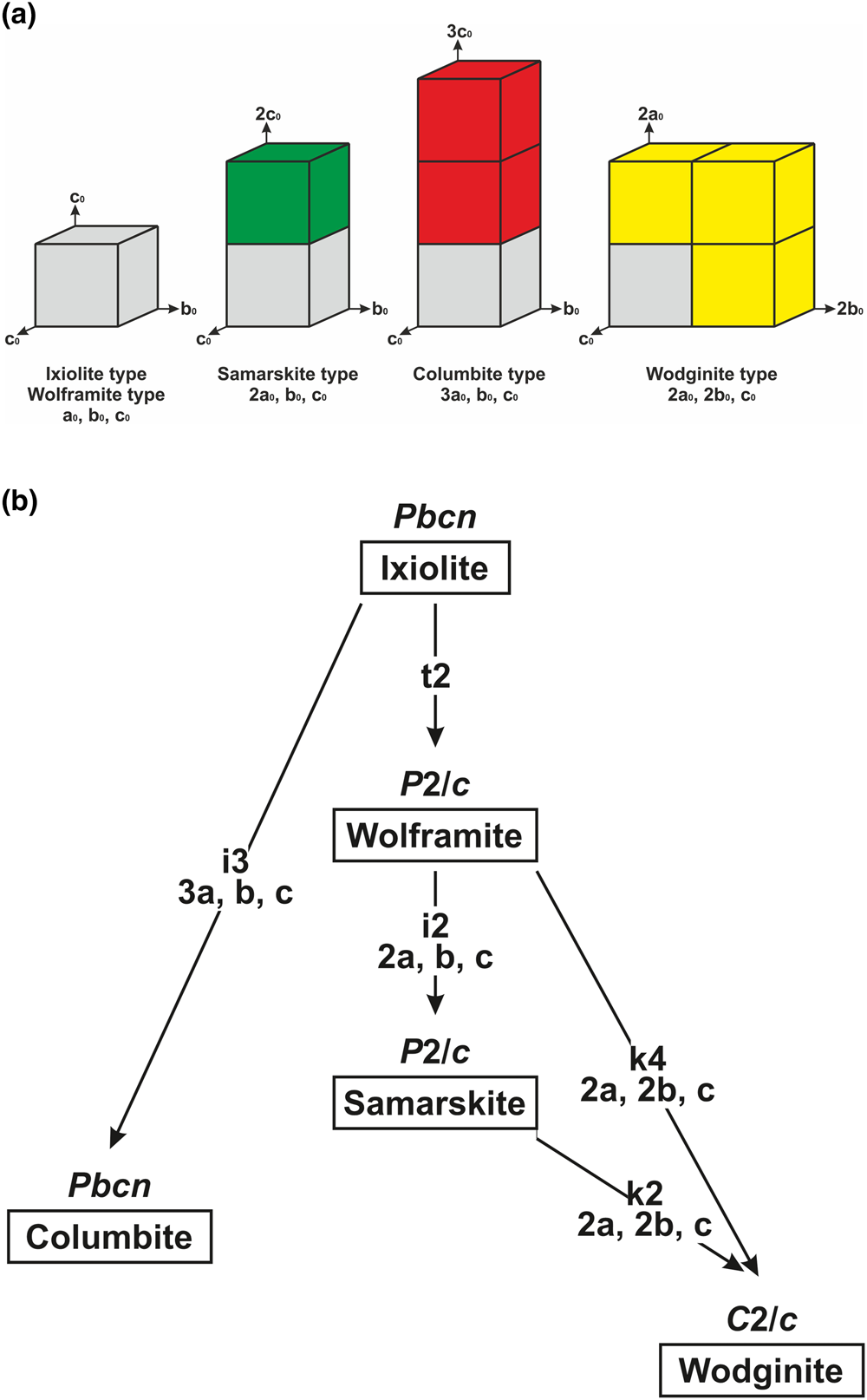
Fig. 2. General comparison of the unit cells (a), and symmetry reduction from the initial aristotype with the ixiolite-type unit cell and the space group Pbcn induced by the different kinds of ordering of cations (b).
Different schemes of ordering of M cations control both the symmetry lowering and multiplying of the basic ixiolite-type unit cell. The Bärnighausen tree (Müller, Reference Müller2004) shown in Fig. 2b illustrates the symmetry relations between different structures.
Minerals belonging to the columbite supergroup
Ixiolite group
Minerals belonging to the ixiolite group with the general formula M1O2 (orthorhombic, Pbcn, a = a0, b = b0, c = c0 and Z = 4) are characterised by a disordered distribution of the cations: in the crystal structure of ixiolite-group minerals (Fig. 3), all cations occupy a single M1 site. In these minerals, edge-sharing M1O6 octahedra form chains along the c direction. In the a direction, the chains are connected with each other via common vertices of the octahedra.

Fig. 3. The crystal structure of ixiolite-group minerals. The unit cell is outlined.
Ixiolite was first described by Nordenskiöld (Reference Nordenskiöld1857) as a tantalum oxide, with subordinate Fe and Mn and minor Sn. The sample originated from Skogsböle, Kimito Island, Finland. The chemical analysis of the sample from Skogsböle is incomplete and corresponds to the approximate formula Ta0.6(Fe,Mn)0.3Sn0.1O2. The Fe:Mn ratio was not determined. On the basis of goniometric measurements, the mineral was assumed to be orthorhombic with a:b:c = 1:0.5508:1.2460. D meas = 7.0–7.1; H(Mohs) = 6–6½.
In another ixiolite sample from Skogsböle, the Mn:Fe ratio is 1.04:1 in atomic units (Rose, Reference Rose1858). Mn-rich ixiolite (with 9.35 wt.% MnO) has been also discovered in pegmatites of the Kalbinskiy range, Russia (Chukhrov and Bonshtedt-Kupletskaya, Reference Chukhrov and Bonshtedt-Kupletskaya1967). The crystal structure of Mn-rich ixiolite with the charge-balanced empirical formula (Ta0.43Nb0.24)Mn2+0.23Mn3+0.07(Ti0.02Sn0.01)O2 from the Tanco pegmatite, Bernic Lake, Manitoba, Canada was solved by Grice et al. (Reference Grice, Ferguson and Hawthorne1976).
The chemical formula of ixiolite is currently given as (Ta,Mn,Nb)O2 which corresponds to an ixiolite-group mineral with Mn as the main charge-balancing component, but samples with Fe > Mn are also known. In most analyses of ixiolite from Skogsböle, Fe prevails over Mn, with Fe:Mn up to 13.8:1 (Rose, Reference Rose1858). Nickel et al. (Reference Nickel, Rowland and McAdam1963a) investigated the crystal structure of an ixiolite sample from Skogsböle with the charge-balanced empirical formula (Ta0.43Nb0.12)(Fe2+0.13Mn2+0.12)Fe3+0.05(Sn0.13Ti0.01Zr0.01)O2. The sample is deposited in the Royal Ontario Museum with the catalogue number M-6591. A synthetic compound with the formula NbFe3+O4 and ixiolite-type structure has been described by Harrison and Cheetham (Reference Harrison and Cheetham1989).
Scrutinyite, α-PbO2 was discovered in two natural occurrences situated in Bingham, New Mexico, USA and Mapimi, Mexico (Taggart et al., Reference Taggart, Foord, Rosenzweig and Hanson1988). The crystal structure of synthetic α-PbO2 was solved by Zaslavskij and Tolkachev (Reference Zaslavskij and Tolkachev1952).
Seifertite, SiO2, is an orthorhombic high-pressure silica polymorph with the ixiolite-type structure. The mineral is a constituent of high-pressure assemblages typical of shock-affected Martian meteorites belonging to the shergottite group (Dera et al., Reference Dera, Prewitt, Boctor and Hemley2002; El Goresy et al., Reference El Goresy, Dera, Sharp and Hemley2008; Zhang et al., Reference Zhang, Popov, Meng, Wang, Ji, Li and Mao2016).
Srilankite, TiO2, was described as a new mineral from Rakwana, Sabaragamuva province, Sri Lanka (Willgallis et al., Reference Willgallis, Siegmann and Hettiaratchi1983). The chemical composition was given originally as (Ti,Zr)O2, with Zr:Ti = 1:2. The ixiolite-type structure of srilankite has been confirmed by a single-crystal X-ray diffraction (XRD) study of a natural sample (Willgallis and Hartl, Reference Willgallis and Hartl1983) and its synthetic analogue (Troitzsch et al., Reference Troitzsch, Christy and Ellis2005). Similarly to transition metals in other ixiolite-group minerals, Ti and Zr in srilankite occupy the same crystallographic M1 site. Zirconium, having an ionic radius larger than titanium, plays an essential role in stabilising the ixiolite-type structure of srilankite at ambient pressure. Zirconium-free srilankite, pure TiO2, was described as a quenched ‘TiO2-II’ polymorph from the Ries impact structure (El Goresy et al., Reference El Goresy, Chen, Gillet, Dubrovinsky, Graup and Ahuja2001), the Xiuyan crater in China (Zhang et al., Reference Zhang, Liou and Ernst2009) and in the high-pressure mineral assemblages of subduction zones (Chen et al., Reference Chen, Gu, Xie and Yin2013).
The Nb-dominant analogue of ixiolite (with Nb > Ta) has been known for a long time (von Knorring and Sahama, Reference von Knorring and Sahama1969; Wise et al., Reference Wise, Černý and Falster1998; Zubkova et al., Reference Zubkova, Chukanov, Pekov, Ternes, Schüller and Pushcharovsky2020). This mineral was described as the new mineral species ‘ashanite’ with the formula (Nb, Ta,U, Fe, Mn)4O8 (Z = 1) (Zhan et al., Reference Zhan, Tian, Peng, Ma, Han and Jing1980). However, in 1998, ‘ashanite’ was discredited by the IMA–CNMNC. This decision was made based on unsatisfactory compositional data for this mineral, suggestive of a mixture of ixiolite, samarskite and uranmicrolite (Shen, Reference Shen1998).
Although there is only one cationic M1 site in the ixiolite-type structure, a charge-balanced end-member formulae of ixiolite and its Nb-dominant analogue cannot be written with a single cationic component. Thus, the dominant-charge-compensating cations (either a lower-valency cation or vacancy) should be taken into account, as discussed by Hatert and Burke (Reference Hatert and Burke2008).
Wolframite group
The wolframite-type structure (M1M2O4, monoclinic, P2/c, a = a0, b = b0, c = c0, β ≈ 91° and Z = 2) is a derivative of the ixiolite-type structure characterised by the ordering of the cations with lowering of the symmetry. It can be represented as a sequence of two kinds of structurally identical, but chemically different, octahedral layers of parallel zig-zag chains alternating along the a axis of the ixiolite quasi-framework (Fig. 4). The larger-radius cations occupy the octahedral M1 site, whereas the smaller-radius cations reside at the M2 octahedron. Consequently, members of the wolframite group are double oxides with the general formula M12+M26+O4 (M1 = Mg, Mn, Fe and Zn; M2 = W) or M13+M25+O4 (M1 = Sc and Fe; M2 = Nb and Ta). The Ti4+Ti4+O4 oxide, riesite, represents a slightly distorted variant of the wolframite structure. The layered ordering of different-sized cations in wolframites results in monoclinic distortion of the ixiolite framework, whereas the unit-cell dimensions of the parent ixiolite remain unchanged (Fig. 5).

Fig. 4. General view of the wolframite-type structure.
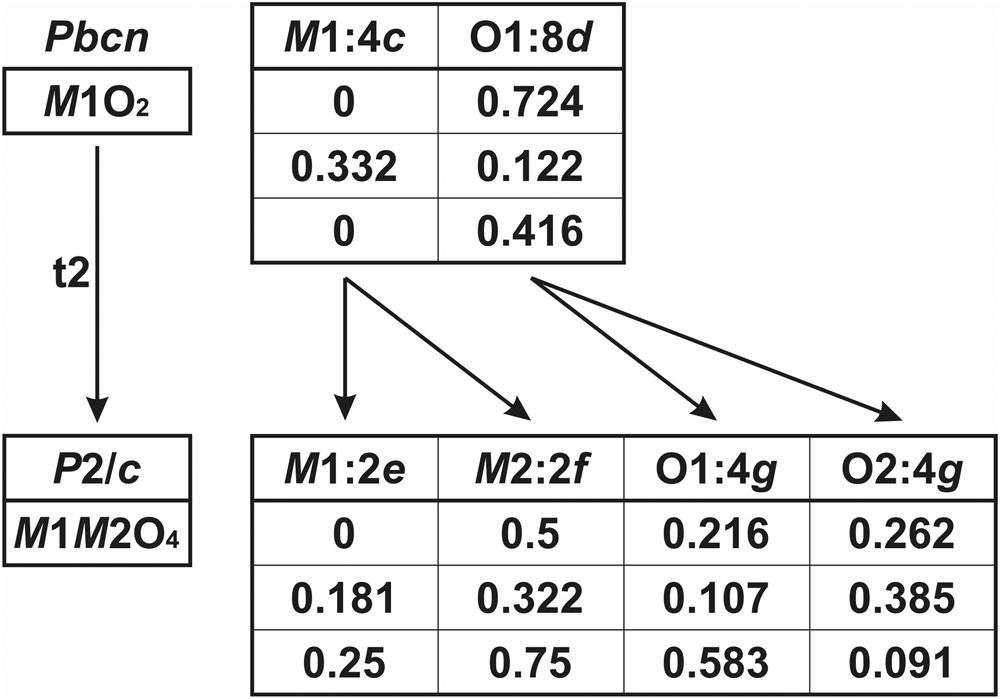
Fig. 5. The scheme of splitting of atomic sites (the upper row) and their coordinates in the ixiolite- and wolframite-type structures in accordance with the relations between the mineral groups (see Fig. 2b). One cationic M1 site and one oxygen O1 site in the ixiolite-type structure split into two symmetrically non-equivalent M1 and M2 as well as O1 and O2 sites in the wolframite-type structure due to the cation ordering and reducing of the symmetry from the space group Pbcn to P2/c.
The wolframite group inherits its name from ‘wolframite’, which is now considered to be an obsolete mineral species. The first scientific description of this mineral with the name ‘Wolfram’ (‘wolf-cream’, from German Wolfram or Wolfrahm) was made by Henckel (Reference Henckel1725).
Historically, wolframites represent intermediate members of the solid solution between pure Fe2+WO4 and pure Mn2+WO4. In particular, the term wolframite indicated minerals with compositions ranging between (Fe0.8Mn0.2)WO4 and (Fe0.2Mn0.8)WO4. The species having Fe > 0.8 and Mn > 0.8 atoms per formula unit (apfu) were called ferberite and hübnerite, respectively. Subsequently, compositional fields of ferberite and hübnerite have been expanded according to the 50% rule and the term ‘wolframite’ has been abandoned. For historical reasons, however, it seems convenient to keep wolframite as the name for the group of ordered structures with an ixiolite-type unit cell, but with the space group P2/c.
Ferberite was first described by Liebe (Reference Liebe1863). The type locality is the Niña mine, Sierra Almagrera, Andalusia, Spain. The crystal structure of ferberite has been refined by Cid-Dresdner and Escobar (Reference Cid-Dresdner and Escobar1968).
Hübnerite was first described by Credner (Reference Credner1865). The type locality is the Ellsworth mine, Nevada, USA. The crystal structure of ferberite has been refined by Dachs et al. (Reference Dachs, Stoll and Weitzel1967).
Huanzalaite is the Mg-dominant analogue of ferberite and hübnerite. It was first described by Miyawaki et al. (Reference Miyawaki, Yokoyama, Matsubara, Furuta, Gomi and Murakami2010). The type locality is the Huanzala mine, Ancash Department, Peru. The crystal structure of its synthetic analogue has been refined by Macavei and Schulz (Reference Macavei and Schulz1993).
Sanmartinite, ideally ZnWO4, was first described by Angelelli and Gordon (Reference Angelelli and Gordon1948). The type locality is the Department of San Martín, San Luis province, Argentina. The crystal structure of sanmartinite has been refined by Redfern et al. (Reference Redfern, Bell, Henderson and Schofield1995).
Heftetjernite, ScTaO4, was first described by Kolitsch et al. (Reference Kolitsch, Kristiansen, Raade and Tillmanns2010), who also refined its crystal structure. The type locality is the Heftetjern pegmatite, Tørdal, Telemark, Norway.
Nioboheftetjernite, ScNbO4, was first described by Lykova et al. (Reference Lykova, Rowe, Poirier, McDonald and Giester2021), who also refined its crystal structure. The type locality is the Befanamo pegmatite, Madagascar.
Rossovskyite was first described by Konovalenko et al. (Reference Konovalenko, Ananyev, Chukanov, Rastsvetaeva, Aksenov, Baeva, Gainov, Vagizov, Lopatin and Nebera2015), who also refined its crystal structure. The type locality is Bulgut, Altai Mountains, Mongolia. The chemical formula of the mineral is given as (Fe3+,Ta)(Nb,Ti)O4. According to the dominant-valency rule and the site-total-charge approach (Bosi et al., Reference Bosi, Hatert, Hålenius, Pasero, Miyawaki and Mills2019), the end-member formula is Fe3+NbO4.
Riesite was reported as a new TiO2 polymorph from impact-affected rocks (suevites) at the Ries impact crater, Germany (Tschauner et al., Reference Tschauner, Ma, Lanzirotti and Newville2020). Similarly to formerly described Zr-free srilankite, riesite was formed by shock-induced transformation of rutile at pressures of 20–25 GPa. In the crystal structure of riesite, the M1 and M2 sites are insignificantly displaced from the general positions of the wolframite-type framework, becoming statistically half-occupied. By analogy with other wolframite-group minerals, the ideal formula of riesite can be written as TiTiO4.
Samarskite group
The samarskite group includes three valid species, namely, samarskite-(Y), ekebergite and shakhdaraite-(Y). These minerals are monoclinic (space group P2/c, a = 2a0, b = b0, c = c0, β ≈ 93° and Z = 2), cation-ordered double niobates and tantalates with the general formula AM1M22O8 (A = Y and Th; M1 = Fe2+, Fe3+ and Sc3+; M2 = Nb and Ta) and unit-cell parameters a = 9.8–9.9, b = 5.6–5.7, c ≈ 5.2 Å, and β = 92–94° (Z = 2). Unlike other columbite-supergroup minerals, members of the samarskite group contain a relatively large cation at the A site with 6 + 2-fold coordination (Fig. 6) due to the slight distortion of the hcp (Lima-de-Faria, Reference Lima-de-Faria2012). Such insertion of a large cation transforms parallel zig-zag chains into a rigid layer of edge-sharing AO8 polyhedra with the preservation of the cation distribution between the ‘octahedral’ voids of hcp (Fig. 7). There are also three insufficiently studied metamict minerals, namely, samarskite-(Yb), ishikawaite and calciosamarskite, that are tentatively assigned to the samarskite group based on their stoichiometry and the powder XRD patterns of annealed samples.
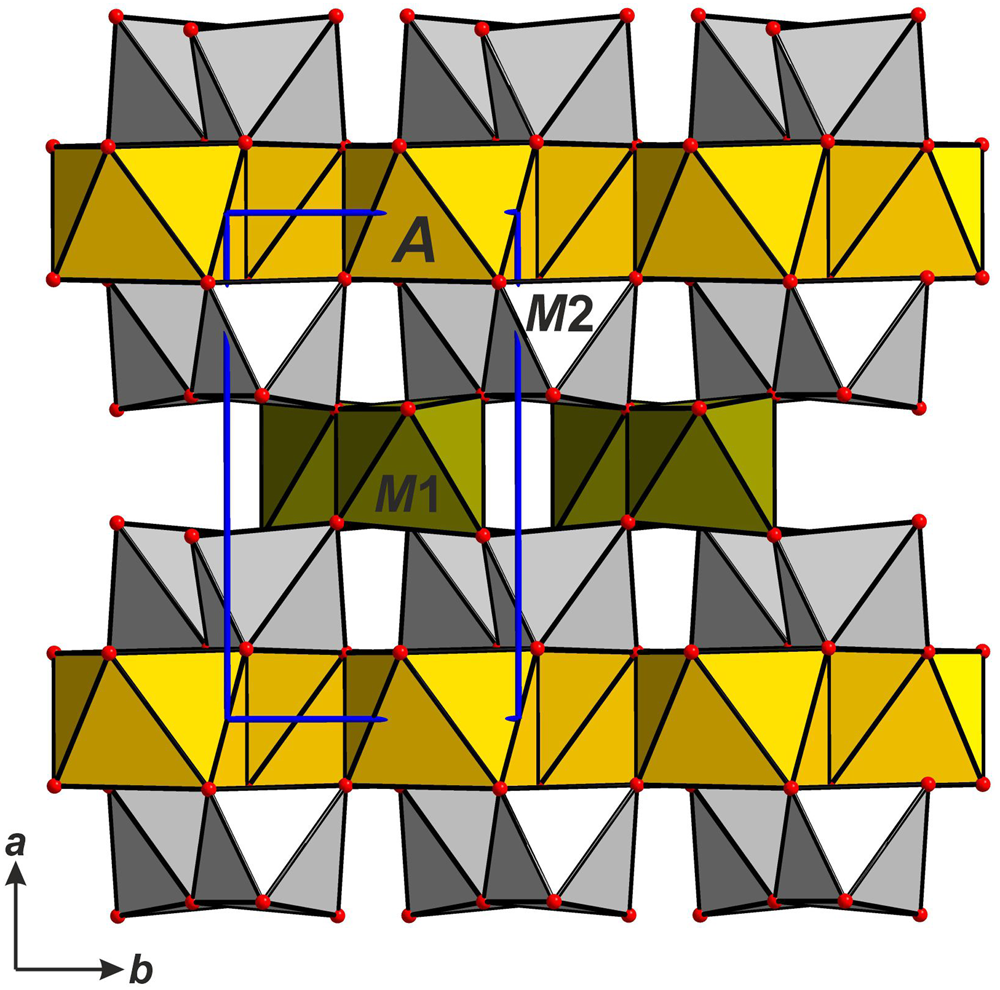
Fig. 6. General view of the samarskite-type structures.

Fig. 7. Transformation of parallel zig-zag chains of edge-sharing octahedra into a solid layer of edge-shared eight-vertex polyhedra with the increasing of the ionic radii of the cation in the samarskite-type structures.
The name samarskite was introduced into the mineralogical literature by Rose (Reference Rose1847) who described a sample from Ilmen Mountains, Chelyabinsk region, Russia. Subsequently, the mineral name was changed to samarskite-(Y) according to general nomenclature rules for the REE-bearing minerals (Levinson, Reference Levinson1966). According to Hanson et al. (Reference Hanson, Simmons, Falster, Foord and Lichte1999), the name samarskite-(Y) is attributed to the samarskite-group mineral in which the A site is dominated by REE cations, among which Y3+ prevails.
Samarskite-(Y) is the first member of the samarskite group whose crystal structure was published. A recent finding of non-metamict samarskite-(Y) allowed the refinement of its crystal structure, and the re-definition of the mineral as YFe3+Nb2O8 (Britvin et al., Reference Britvin, Pekov, Krzhizhanovskaya, Agakhanov, Ternes, Schüller and Chukanov2019). These authors confirmed that this new chemical formula, with Fe3+ as a species-forming constituent, corresponds to the formula of holotype samarskite-(Y).
Ekebergite, ideally ThFe2+Nb2O8, was approved as a new mineral species in 2018 (Kjellman et al., Reference Kjellman, Pay Gómez, Lazor, Majka, Stanley and Najorka2018). This mineral originates from the pumice quarry ‘In den Dellen’ (Bimsgrube Zieglowski), Mendig, Laacher See (Laach Lake) complex, Eifel, Rhineland-Palatinate, Germany. Ekebergite is isostructural with samarskite and forms a solid-solution series with samarskite. The full description of the mineral has not as yet been published.
Shakhdaraite-(Y), YScNb2O8, was described as a new mineral from Tajikistan (Pautov et al., Reference Pautov, Mirakov, Sokolova, Day, Hawthorne, Schodibekov, Karpenko, Makhmadsharif and Faiziev2022). It is the Sc-dominant analogue of samarskite-(Y).
Samarskite-(Yb), YbFe3+Nb2O8, was described as a new mineral by Simmons et al. (Reference Simmons, Hanson and Falster2006). It occurs as a metamict mineral at the Little Patsy pegmatite, South Platte district, Jefferson Co., Colorado, USA. The mineral recrystallised after heating at 1100°C for 12 h.
Ishikawaite was first described as an unnamed mineral from Ishikawa, Iwaki province, Japan, by Shimata and Kimura (Reference Shimata and Kimura1922a) and then named ishikawaite after the type locality (Shimata and Kimura, Reference Shimata and Kimura1922b). Its chemical formula is currently given as (U,Fe,Y)NbO4. According to Hanson et al. (Reference Hanson, Simmons, Falster, Foord and Lichte1999), the name ishikawaite should be attributed to the samarskite-group mineral in which the A site is dominated by U4+. Under this assumption, ishikawaite should be considered as the analogue of ekebergite with U4+ > Th and the end-member formula U4+Fe2+Nb2O8.
Calciosamarskite was first described by Ellsworth (Reference Ellsworth1928a, Reference Ellsworth1928b) as the Ca-dominant analogue of samarskite. Its chemical formula is currently given as (Ca,Fe,Y)(Nb,Ta,Ti)O4. The mineral was supposed to be discredited (see Hanson et al., Reference Hanson, Simmons, Falster, Foord and Lichte1999), but actually it is still considered a valid, grandfathered species. According to Hanson et al. (Reference Hanson, Simmons, Falster, Foord and Lichte1999), the name calciosamarskite should be attributed to the samarskite-group mineral in which the A site is dominated by Ca. However, the end-member formula CaFe3+Nb2O8, which would be expected for a Ca-dominant samarskite-group mineral, is not charge-balanced even with trivalent iron. The formula CaFe3+Nb2O7(OH) is neutral, but the presence of OH groups in calciosamarskite is questionable. Probably, this problem could be solved based on data for the synthetic analogue.
Columbite group
The columbite group includes double oxides with the general formula M12+M25+2O6 (orthorhombic, Pbcn, a = 3a0, b = b0, c = c0 and Z = 4; M1 = Mg, Ca, Mn and Fe; M2 = Nb and Ta). In the crystal structure of these minerals (Fig. 8), M1O6 octahedra share edges to form infinite zig-zag chains along the c axis. Similar chains are formed by the M2O6 octahedra. Thus, alternating [100] ‘layers’ are formed: a single ‘layer’ consisting of chains of M1O6 octahedra and double ‘layers’ comprising chains of M2O6 octahedra. The chains of the neighbouring layers are linked via common vertices.

Fig. 8. The general view of the columbite-type structure. The unit cell is outlined.
Columbite-(Fe), Fe2+Nb2O6, is the current name of the mineral originally described as ‘columbite’ and later named ferrocolumbite. Columbite was first described by Jameson (Reference Jameson1805). The type locality is likely to be either Haddam or Middletown, both in Connecticut, USA (cf. Dana, Reference Dana1892). The mineral was renamed to columbite-(Fe) after Burke (Reference Burke2008). The crystal structure of natural columbite-(Fe) from S. José de Safira, Minas Gerais, Brazil has been refined by Tarantino and Zema (Reference Tarantino and Zema2005).
Columbite-(Mn), Mn2+Nb2O6, was first described by Dana (Reference Dana1892) under the name manganocolumbite. This mineral was considered initially to be a Mn-dominant variety of columbite. The mineral was renamed to columbite-(Mn) after Burke (Reference Burke2008). The crystal structure of natural columbite-(Mn) from Kragero, Norway has been refined by Tarantino and Zema (Reference Tarantino and Zema2005).
Columbite-(Mg), MgNb2O6, the Mg-dominant member of the columbite solid-solution series, was first found in the Muzeinaya vein, Gorno-Badakhshan, Tajikistan (Mathias et al., Reference Mathias, Rossovskii, Shostatskii and Kumskova1963). The mineral was originally named magnocolumbite and then renamed to columbite-(Mg) after Burke (Reference Burke2008). The crystal structure of synthetic MgNb2O6 has been refined by Pagola et al. (Reference Pagola, Carbonio, Alonso and Fernandez-Diaz1997).
Tantalite-(Fe), Fe2+Ta2O6, is the current name of the mineral originally described as ‘tantalite’ and then named ‘ferrotantalite’. Tantalite was first described by Thomson (Reference Thomson1836). The type locality is Upper Bear Gulch, Tinton pegmatite district, Lawrence Co., South Dakota, USA. The mineral was renamed to tantalite-(Fe) after Burke (Reference Burke2008). An overwhelming majority of analysed tantalite-(Fe) samples contain significant amounts of Mn and/or Nb. Samples with compositions close to the Fe2+Ta2O6 end-member have the tapiolite structure (Ercit et al., Reference Ercit, Wise and Černý1995).
Tantalite-(Mn), Mn2+Ta2O6, was first described as ‘manganotantalite’, a Mn-dominant variety of tantalite by Nordenskiöld (Reference Nordenskiöld1877). The type locality is the Utö Mines, Stockholm Co., Sweden. The mineral was renamed to tantalite-(Mn) after Burke (Reference Burke2008). The crystal structure of natural tantalite-(Mn) from the Tanco pegmatite, Manitoba, Canada has been refined by Grice et al. (Reference Grice, Ferguson and Hawthorne1976).
Tantalite-(Mg), MgTa2O6, was described as a new mineral ‘magnesiotantalite’ from Lipovka, Central Urals, Russia by Pekov et al. (Reference Pekov, Yakubovich, Shcherbachev and Kononkova2003). The mineral was renamed to tantalite-(Mg) after Burke (Reference Burke2008).
Similarly to the samarskite-type structures (Fig. 7), the insertion of cations with large ionic radii into the columbite-type structure causes the parallel zig-zag chains to transform into a rigid layer. Such layers of edge-shared AO8-polyhedra (A = Ca and Y) have been found in the euxenite derivative of the columbite-type structure, where they alternate with double ‘layers’ containing zig-zag chains of M2O6 octahedra (Fig. 9). Despite the distortion of the initial hcp, the distribution of the cations over the ‘octahedral’ void in the euxenite derivative are exactly equal to those in the columbite-type structure (Lima-de-Faria, Reference Lima-de-Faria2012).

Fig. 9. General view of the euxenite derivative of the columbite-type structure, containing layers of edge-shared eight-vertex polyhedra.
Fersmite, CaNb2O6, was discovered in the pegmatites of the Vishnevye Mountains, Central Urals (Bohnstedt-Kupletskaya and Burova, Reference Bohnstedt-Kupletskaya and Burova1946). The crystal structure of fersmite was solved by Aleksandrov (Reference Aleksandrov1960). The presumed synthetic analogue of fersmite is orthorhombic in space group Pcan, with a = 5.75, b = 14.03 and c = 5.20 Å and Z = 4 (Cummings and Simonsen, Reference Cummings and Simonsen1970). Unlike other tantalite-group minerals, fersmite contains a rather large Ca cation having 8-fold coordination. Fersmite is dimorphous with the aeschynite-group mineral vigezzite.
Based on the stoichiometry, powder XRD patterns of annealed samples, and crystal structures of presumed synthetic analogues, four minerals whose natural samples are usually metamict [namely, euxenite-(Y), polycrase-(Y), tanteuxenite-(Y) and uranopolycrase] can be assigned tentatively to the columbite group (Palache et al., Reference Palache, Berman and Frondel1944; Weitzel and Schröcke, Reference Weitzel and Schröcke1980; Aurisicchio et al., Reference Aurisicchio, Orlandi, Pasero and Perchiazzi1993).
Euxenite-(Y) is orthorhombic, with the end-member formula YNbTiO6 and unit-cell parameters a ≈ 14.6, b ≈ 5.55 and c ≈ 5.2 Å. For example, the empirical formula of euxenite-(Y) from Lyndoch Township, Ontario, Canada (Ellsworth, Reference Ellsworth1927) calculated on 2(Nb + Ta + Ti + Fe3++Al) apfu is [(Ca0.31Fe2+0.04Mn0.02Pb0.01)Σ0.38(Y0.58Ce0.10)Σ0.68(Th0.07U0.01)Σ0.08][(Fe3+0.06Al0.01)Σ0.07Ti0.74(Nb1.13Ta0.06)Σ1.19]O6.34. Numerous chemical data of euxenite-(Y) are given in the reference book Minerals (Chukhrov and Bonshtedt-Kupletskaya, Reference Chukhrov and Bonshtedt-Kupletskaya1967). All of them correspond to the end-member formula YNbTiO6. The unit-cell parameters of a metamict euxenite-(Y) sample with the empirical formula (REE 0.92Ca0.08U0.11Th0.06Mn0.01)Σ1.18(Nb0.84Ta0.09Ti0.84Fe0.12)Σ1.89O6 from a rare-metal pegmatite, which was annealed at 900°C, are a ≈ 14.68, b ≈ 5.56 and c ≈ 5.18 Å (Sokolova, Reference Sokolova1959). The unit-cell parameters of synthetic YNbTiO6 (Weitzel and Schröcke, Reference Weitzel and Schröcke1980) are a = 14.64, b = 5.55 and c = 5.20 Å.
‘Polycrase-(Y)’, which was considered an analogue of euxenite-(Y) with Ti > Nb in atomic units (Johnsen et al., Reference Johnsen, Stahl, Petersen and Micheelsen1999), is rarer. The empirical formula of metamict polycrase-(Y) from Birkenes, Norway is (Y0.47Ln0.20Ca0.19U0.18Th0.06)Σ1.10(Ti1.19Nb0.71Ta0.07)Σ1.97O6 (Tomašić et al., Reference Tomašić, Galović, Bermanec and Rajić2004).
Non-metamict polycrase-(Y) with the unit-cell parameters a =14.82, b = 5.66 and c = 5.22 Å was described by Guastoni et al. (Reference Guastoni, Secco, Škoda, Nestola, Schiazza, Novák and Pennacchioni2019). It occurs in the Fiume pegmatite dyke, Vigezzo Valley, Central Alps, Italy. Its simplified empirical formula (analysis 9/1 in the cited paper) is (Ca,Mn,Fe2+)0.085REE 0.78(U,Th)0.19Ti1.14Si0.01(Nb,Ta)0.78W0.01.
Another sample described by Guastoni et al. (Reference Guastoni, Secco, Škoda, Nestola, Schiazza, Novák and Pennacchioni2019) originates from the Bosco dyke situated in the same region. It is an intermediate member of the euxenite-(Y)–polycrase-(Y) solid-solution series and has the simplified formula (Ca,Mn,Fe2+)0.165REE 0.84(U,Th)0.10Ti0.96Si0.01(Nb,Ta)0.96W0.01. This sample is also non-metamict and has the unit-cell parameters a = 14.736, b = 5.605 and c = 5.184 Å. All available analyses of polycrase-(Y) correspond to the end-member formula Y(NbTi)O6.
Thus, euxenite-(Y) and polycrase-(Y) (including those of annealed samples) are minerals with identical unit-cell parameters and the common end-member formula Y(NbTi)O6. Consequently, these minerals should be considered as the same mineral species. The name euxenite-(Y), as the older of the two, has priority.
Tanteuxenite-(Y), YTaTiO6, is a rare mineral first described from Western Australia (Simpson, Reference Simpson1928) and reported from a few other localities. The mineral is usually metamict.
Uranopolycrase, ideally UTi2O6, was described as a new mineral from Elba Island, Italy. Because the mineral is metamict, its crystal structure has been refined on a sample annealed at 900°C for 10 h (Aurisicchio et al., Reference Aurisicchio, Orlandi, Pasero and Perchiazzi1993).
Wodginite group
The wodginite group includes monoclinic minerals (space group C2/c; a = 2a0, b = 2b0, c = c0, β ≈ 91° and Z = 4) with the general formula M1M2M32O8. The dominant cations at the M sites are: M1 = Mn2+, Fe2+ and Li; M2 = Ti, Sn4+ and Ta; M3 = Ta. The structure of these minerals (Ercit et al., Reference Ercit, Hawthorne and Černý1992a) is based on alternating (100) ‘layers’ consisting of chains of edge-sharing MO6 octahedra running along the c axis (Fig. 10). The ‘layers’ of the first type contain chains of M3O6 octahedra, whereas the ‘layers’ of the second type contain chains of alternating M1O6 and M2O6 octahedra (Fig. 11). The chains of the neighbouring layers are linked via common vertices. The structures of wodginite-group minerals are characterised by a different degree of ordering of cations among the M sites; the heating of samples at 1000°C for 16 hours induces a full order of cations in wodginite-group minerals (Ercit et al., Reference Ercit, Hawthorne and Černý1992a, Reference Ercit, Černý, Hawthorne and McCammon1992b, Reference Ercit, Černý and Hawthorne1992c).

Fig. 10. General view of the wodginite-type structure.

Fig. 11. Two types of layers containing zig-zag chains in the wodginite-type structure.
Wodginite, ideally MnSnTa2O8, was described as a new mineral from two localities, Wodgina, Western Australia and Bernic Lake, Manitoba, Canada (Nickel et al., Reference Nickel, Rowland and McAdam1963b). On the basis of powder XRD data, its crystal structure was recognised as a superstructure of ixiolite. The crystal-structure refinements have been carried out by Ercit et al. (Reference Ercit, Hawthorne and Černý1992a), who have shown that different samples have different degrees of Ta disorder. Partially ordered samples are structurally intermediate between wodginite and ixiolite. The crystal structure of wodginite from Wodgina was investigated by Graham and Thornber (Reference Graham and Thornber1974b). Later, the crystal structure of wodginite from Bernic Lake was solved by Ferguson et al. (Reference Ferguson, Hawthorne and Grice1976).
Ferrowodginite, FeSnTa2O8, was characterised as a new mineral species by Ercit et al. (Reference Ercit, Černý and Hawthorne1992c). In the type specimen, ferrowodginite occurs as 0.01 to 0.2 mm inclusions in cassiterite from a granitic pegmatite near Sukula, southwestern Finland.
Titanowodginite, MnTiTa2O8, holotype material occurs as euhedral crystals up to 1 cm across at the Tanco pegmatite, Bernic Lake, Manitoba, Canada. Its crystal structure was solved by Ercit et al. (Reference Ercit, Černý and Hawthorne1992c).
Ferrotitanowodginite, FeTiTa2O8, has been described from the San Elías pegmatite, Sierra de la Estanzuela, San Luis Province, Argentina (Galliski et al., Reference Galliski, Černý, Márquez-Zavalía and Chapman1999).
Tantalowodginite, (Mn0.5□0.5)TaTa2O8, was found in the Emmons granite pegmatite dyke in Oxford County, Maine, USA (Hanson et al., Reference Hanson, Falster, Simmons, Sprague, Vignola, Rotiroti, Andó and Hatert2018).
Lithiowodginite, LiTa3O8 or LiTaTa2O8, was discovered at the Ognevka and Yubileinoye tantalum deposits, Kalba Mountains, eastern Kazakhstan (Voloshin et al., Reference Voloshin, Pakhomovskii and Bakhchisaraytsev1990).
Achalaite, Fe2+TiNb2O8, is the first niobium-dominant member of the wodginite group and was described from the La Calandria granite pegmatite, Cañada del Puerto, Córdoba province, Argentina (Galliski et al., Reference Galliski, Márquez-Zavalía, Černý, Lira, Colombo, Roberts and Bernhardt2016).
Ungrouped columbite-supergroup mineral
Lithiotantite, LiTa3O8, with space group P21/c, a = 7.44 b = 5.04 c = 15.25 Å, β = 107.2° and Z = 4, is chemically and topologically identical to lithiowodginite (Fig. 12) (Voloshin et al., Reference Voloshin, Pakhomovskii and Bakhchisaraytsev1990; Ercit et al., Reference Ercit, Hawthorne and Černý1992a, Reference Ercit, Černý and Hawthorne1992c).

Fig. 12. The crystal structure of lithiotantite (left) and the proposed structure of qitianlingite (right).
Insufficiently studied minerals
The minerals listed below are not currently included in the columbite supergroup, pending reliable data on their chemical composition and crystal structure.
Qitianlingite is a mineral related to the members of the columbite and tantalite solid-solution series. It was described as a new mineral species with the ideal formula Fe2+2Nb2W6+O10 (Yang et al., Reference Yang, Wang, Peng and Bu1985). Qitianlingite was named after the type locality (Qitianling granite, Hunan Province, China). The crystal structure of qitianlingite has been refined by Peng et al. (Reference Peng, Wang, Ma and Yang1988), who described it as a superstructure of ixiolite with ordered cation distribution and a unit cell with the a axis approximately 5 times larger than the a axis of ixiolite (Fig. 12). However, calculated powder diffraction data confirming the superstructure of qitianlingite are not given in these papers. Indexing of all assumed superstructure reflections in the measured powder data is not in accordance with the pattern calculated from the proposed structure; all observed reflections can be indexed using an ixiolite-type cell. The holotype material of this mineral needs additional investigation.
Yttrocolumbite-(Y), (Y,U,Fe2+)(Nb,Ta)(O,OH)4, is a questionable mineral described by Lepierre (Reference Lepierre1937). This mineral has been considered to be the Nb-dominant (with Nb > Ta) analogue of yttrotantalite-(Y). Natural yttrocolumbite-(Y) is metamict. The idealised formula of yttrocolumbite-(Y) coincides with those of fergusonite-(Y) and fergusonite-β-(Y).
Yttrotantalite-(Y) was described as a new mineral from Sweden (Ekeberg, Reference Ekeberg1802). Its chemical formula is currently given as (Y,U,Fe2+)(Ta,Nb)(O,OH)4. Actually, its ideal chemical formula should be reduced to YTaO4. Natural yttrotantalite-(Y) is metamict. It is considered to be a polymorph of formanite-(Y). Crystal structure refinements of yttrotantalite-(Y) have been carried out on presumed synthetic analogues; Keller (Reference Keller1962) described it with a samarskite-like unit cell, whereas Wolten (Reference Wolten1967) described it with a wolframite-like unit cell.
Yttrocrasite-(Y) is an ill-defined mineral described as an yttrium–thorium–uranium titanate from Burnet County, Texas, USA (Hidden and Warren, Reference Hidden and Warren1906). Its chemical formula is currently given as (Y,Th,Ca,U)(Ti,Fe)2(O,OH)6.
A mineral with the empirical formula [REE 0.52(U,Th)0.25(Fe2+,Mn,Ca)0.20]Σ0.97[(Nb,Ta)1.26Fe3+0.43(Ti,Zr,Sn,Hf)0.28W0.03]O6 and with Y as the predominant REE was described by Nakajima and Kurosawa (Reference Nakajima and Kurosawa2006) as ‘euxenite’. If this sample is isostructural with euxenite, its end-member formula should be Y(Nb1.5Fe3+0.5)O6. Unfortunately, no X-ray diffraction data have been provided for this mineral.
Ginzburg et al. (Reference Ginzburg, Gorzhevskaya, Sidorenko and Ukhina1969) described a so-called ‘wolframoixiolite’ from an unknown locality. The empirical formula of this sample is (Nb0.54W0.46Fe0.40Mn0.30Ta0.10Zr0.06U0.05Ca0.03Mg0.01Ti0.01)Σ1.96O4⋅0.84H2O. The powder diffraction data were indexed with a monoclinic cell P2/c, a = 4.750, b = 5.72, c = 5.06 Å and β = 90°. A monoclinic cell was required because not all lines could be indexed with the ixiolite cell. Wang et al. (Reference Wang, Ma and Peng1988) described a homogeneous material with the composition (Nb0.70Fe0.50W0.38Mn0.23Ta0.12Ti0.03Sn0.01)Σ1.97O4.00, monoclinic, space group Pc, with a = 4.674, b = 3.673, c = 5.050 Å and β = 90°. Borneman-Starynkevitch et al. (Reference Borneman-Starynkevitch, Rudnitskaya, Loseva and Amelina1974), during a reinvestigation of the type material by electron microprobe analysis, found a Nb–Ta–Mn mineral without W as the main phase. The authors discuss whether wolframoixiolite is really a homogenous mineral or a mixture of ferberite with columbite. Eventually Nickel and Mandarino (Reference Nickel and Mandarino1987) listed wolframoixiolite as a discredited mineral. Taking into account the relationships Nb > W, Fe + Mn > W, and Fe > Mn and under the assumption of a disordered cation distribution, the end-member formula of ‘wolframoixiolite’ could be (Nb2/3Fe2+1/3)O2. However, this mineral also needs additional investigation.
Summary of the approved report
Establishment of the supergroup
The columbite supergroup is established. It is divided into the ixiolite group, the wolframite group, the samarskite group, the columbite group and the wodginite group (Table 1).
Table 1. Minerals belonging to the columbite supergroup.
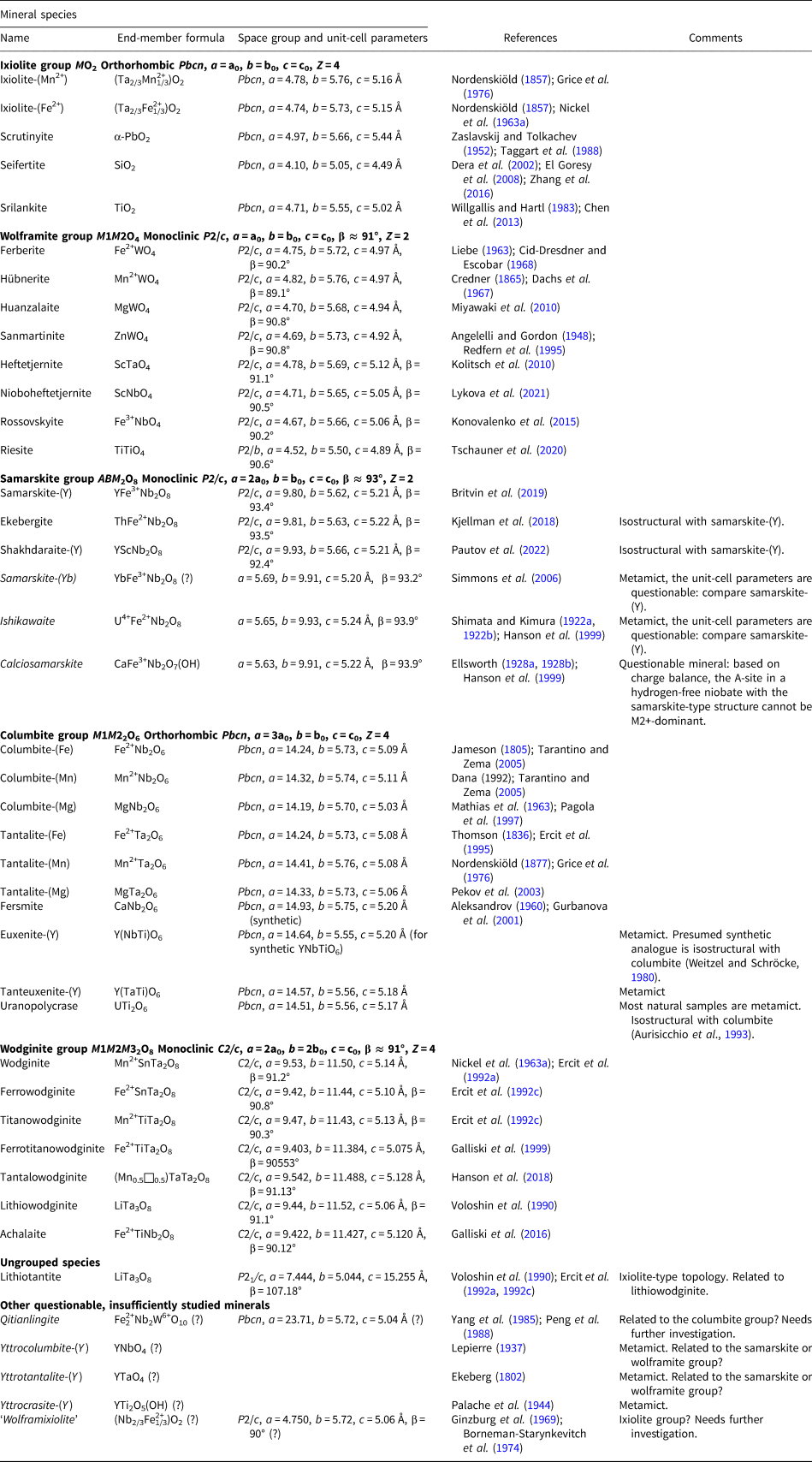
Note: Names of insufficiently studied minerals are italicised.
Redefined species
Currently, the IMA-accepted formulae of some mineral species belonging to the columbite supergroup do not correspond to their end-members. An introduction of end-member formulae for these minerals implies their redefinition. All these changes are summarised in Table 2.
Table 2. Changes in the formulae of columbite-supergroup minerals.

Discredited species
The currently IMA-accepted formula for polycrase-(Y) is Y(Ti,Nb)2(O,OH)6. Its end-member formula is Y(NbTi)O6, which is identical to the revised formula of euxenite-(Y) (cf. Table 2). As euxenite (Scheerer, Reference Scheerer1840) is older than polycrase (Scheerer, Reference Scheerer1844), polycrase-(Y) should be discredited.
New species within the ixiolite group
As noted above, Nb-dominant analogues of ixiolite with different schemes of charge balancing are known from numerous localities. In order to distinguish minerals with different kinds of dominant charge-compensating cations (DCCC), the end-member formula will depend on the dominant cation within the dominant valence state of the charge-compensating cation. Accordingly, formulae will have the form:




The DCCC will be appended to the root name ‘ixiolite’ (for Ta-dominant end-members) or ‘nioboixiolite’ (for Nb-dominant end-members). Accordingly:
(1) The current ‘ixiolite’ will become ixiolite-(Mn2+) with the formula (Ta2/3Mn2+1/3)O2.
(2) Because Fe2+-dominant ‘ixiolite’ is also known to occur at the same locality (Rose, Reference Rose1858; Nickel et al., Reference Nickel, Rowland and McAdam1963a), ixiolite-(Fe2+) is now considered a distinct mineral species, with the formula (Ta2/3Fe2+1/3)O2. The type locality for ixiolite-(Fe2+) is Skogsböle, Kimito, Finland. A similar procedure was adopted recently for the two grandfathered minerals ‘tetrahedrite’ and ‘tennantite’: both were redefined into two distinct species, after the IMA-approved report on the tetrahedrite group (Biagioni et al., Reference Biagioni, George, Cook, Makovicky, Moëlo, Pasero, Sejkora, Stanley, Welch and Bosi2020).
The names with no suffixes: ‘ixiolite’ and ‘nioboixiolite’, will not refer to any specific mineral species and will have the status of series names.
The status of the ixiolite-related mineral qitianlingite remains unclear until more reliable data on the crystal structure of the holotype sample is solved.
Change of status
The crystal structures of three metamict minerals tentatively assigned to the samarskite group [namely, samarskite-(Yb), approved with the current formula YbNbO4, ishikawaite, grandfathered with the current formula (U,Fe,Y)NbO4, and calciosamarskite, grandfathered with the current formula (Ca,Fe,Y) (Nb,Ta,Ti)O4] are unknown. Provided that these minerals are isostructural with samarskite-(Y), their end-member formulae could be written as YbFe3+Nb2O8, U4+Fe2+Nb2O8, and CaFe3+Nb2O7(OH), respectively. However, before making effective the changes in their end-member formulae, all these minerals need further study and so currently should be considered as questionable species; for instance, according to the type description of samarskite-(Yb) (Simmons et al., Reference Simmons, Hanson and Falster2006), the mineral is iron-depleted, with only 0.11 Fe apfu, and all iron tentatively given as Fe2+.
The status of yttrotantalite-(Y) is changed from Rn (renamed) to Q (questionable).
Acknowledgements
The members of the IMA–CNMNC are thanked for their constructive criticism during the two-step (comments + voting) procedure on the submitted proposal. We are grateful to Prof. Anthony R. Kampf and an anonymous reviewer for valuable comments. A part of this work related to the ixiolite group was carried out in with the financial support of the Russian Science Foundation (grant no. 19-17-00050 for N.V.Z.). Topological and modular analysis was performed with the financial support of the Russian Science Foundation (grant no. 20-77-10065 for SMA). The overview of the crystal chemistry of minerals related to the columbite supergroup was carried out in accordance with the state task of Russian Federation, state registration number ААAА-А19-119092390076-7.
Supplementary material
To view supplementary material for this article, please visit https://doi.org/10.1180/mgm.2022.105
Competing interests
The authors declare none.
Appendix I
Topological features of columbite-supergroup minerals and crystal chemical isotypism between the columbite-type structure and the euxenite-type derivative
Ixiolite-, columbite-, wolframite- and wodginite-group minerals as well as lithiotantite are characterised by the same topology of their atomic nets. Topological analysis of the octahedral frameworks in the columbite-supergroup members was performed based on a natural tiling (i.e. partition of the crystal space into the smallest cage-like units: Blatov et al., Reference Blatov2009) analysis of the 3D nets using ToposPro software (Blatov et al., Reference Blatov, Shevchenko and Proserpio2014). The atomic nets were simplified and the corresponding underlying nets, which characterise the connectivity of the primary structural units, were obtained. Topological analysis of the frameworks was performed based on a natural tiles analysis, where the tiles are the smallest clusters of the 3D nets, and are characterised by the following set of tiles (Blatov et al., Reference Blatov, O'Keeffe and Proserpio2010): [4.62]2[6.82]2[62.82] (Fig. A1). The further simplification of the 3D net using standard representation, where only the centres (M cations) of the primary building units (PBUs) are retained in the underlying net, while the 3-connected ligands are pulled into edges, acting as bridges between the PBUs (Shevchenko and Blatov, Reference Shevchenko and Blatov2021), gives the [32.42]2[34.42] set of tiles for the cationic 3D net (Fig. A1).

Fig. A1. Topological features of the ixiolite-type structures.
Analysis of the crystal-chemical similarity is a useful tool to evaluate the crystal-chemical relations between different compounds with the same symmetry and unit-cell parameters for their systematics (Aksenov et al., Reference Aksenov, Ryanskaya, Shchapova Yu, Chukanov, Vladykin, Votyakov and Rastsvetaeva2021a, Reference Aksenov, Antonov, Deyneko, Krivovichev and Merlino2022a). In accordance with the nomenclature of inorganic structure types, two structures are defined as configurationally isotypic if: (1) they are isopointal Footnote 1 and (2) for all corresponding Wyckoff positions, both the crystallographic point configurations and their geometrical interrelationships are similar (Lima-de-Faria et al., Reference Lima-de-Faria, Hellner, Liebau, Makovicky and Parthé1990). Comparison of the crystal structures of columbite-(Fe) (Balassone et al., Reference Balassone, Danisi, Armbruster, Altomare, Moliterni, Grazia, Petti, Mondillo, Ghiara and Saviano2015) with the columbite-type structure and fersmite (Gurbanova et al., Reference Gurbanova, Rastsvetaeva, Kashaev and Smolin2001) with the euxenite-type derivative structure was done using the program COMPSTRU (de la Flor et al., Reference De la Flor, Orobengoa, Tasci, Perez-Mato and Aroyo2016). In the crystal structures of both minerals, all the atoms fill the same Wyckoff positions. The calculated measure of similarity (Δ) (Bergerhoff et al., Reference Bergerhoff, Berndt, Brandenburg and Degen1999) is 0.134 (Table A1). Thus, despite the difference in coordination environments and coordination numbers of the M-sites, both minerals are configurationally isotypic. Similar crystal-chemical relations between structures characterised by different coordination environments of the cation have been described i.e. for the natural and synthetic compounds with the general formula A 2M 3(TO4)4 (Aksenov et al., Reference Aksenov, Antonov, Deyneko, Krivovichev and Merlino2022a).
Table A1. Evaluation of the structure similarities between the columbite-type structure and euxenite-type derivative.*

Appendix II
Ixiolite–euxenite (Eux)n(Ixi)m-polysomatic series
The crystal structures with euxenite- and samarskite-type structure minerals are characterised by the presence of cations with the ionic radii > 0.9 Å (Y3+, Th4+, etc.), which leads to considerable distortion of the initial hcp and with the formation of the layer of edge-shared eight-vertex polyhedra (Voloshin, Reference Voloshin1993; Capitani et al., Reference Capitani, Mugnaioli and Guastoni2016; Britvin et al., Reference Britvin, Pekov, Krzhizhanovskaya, Agakhanov, Ternes, Schüller and Chukanov2019). The increase of the coordination number from 6 to 8 is in good agreement with values of valence sums for two additional bonds. This results in a significant transformation of the parental ixiolite-type topology.
In this case, in accordance with the published data on natural fersmite (as well as other members of euxenite group) and members of the samarskite group, these minerals should be considered as modular structures [by analogy with högbomite-group minerals composed of spinel (S) and nolanite (N) modules; Armbruster, Reference Armbruster2002], in which the crystal structures are based on slightly distorted hcp and consist of two types of modules:
Euxenite (Eux) module: The ‘euxenite’ (Eux) module has the general formula [[8]AO2] and is represented by a central layer of edge-sharing AO8-polyhedra (screwed cubes).
Ixiolite (Ixi) module: The single-layered ‘ixiolite’ (Ixi) module with the general formula [[6]BO2] is represented by zig-zag chains of edge-sharing BO6-octahedra.
The occurrence of either of the above modules, or both, gives rise to the ixiolite–euxenite (Eux)n(Ixi)m-polysomatic series with the general formula [[8]AO2]n[[6]BO2]m or [[8]An [6]BmO2(n+m)]. The polysomes are (Fig A2): ixiolite type, with n = 0 and m = 1; euxenite type, with n = 1 and m = 2; and samarskite type, with n = 1 and m = 3.

Fig. A2. The crystal structures of the members of (Eux)n(Ixi)m-polysomatic series.
In general, the structure containing only Eux-modules (n = 1, m = 0) is characterised by a highly distorted fluorite-type topology (Sulyanova and Sobolev, Reference Sulyanova and Sobolev2022). However, a direct link between two Eux-modules seems unlikely because of the considerable distortion of the AO8 polyhedra (torsion angles in the polyhedra between the oxygen atoms of the adjacent hcp layers vary from 17° to 62°), accompanied by corresponding distortions of the oxygen layers. As a result, the distances and angles between the oxygen atoms of the same hcp layer become unsuitable for the formation of the square face of the AO8-polyhedron of the adjacent Eux-module (Fig. A3).

Fig. A3. The geometrical characteristics of the squares in the distorted hcp oxygen layers of the Eux-module. Irregularity in the angles of the blue square in comparison with the greenish one, which forms the face of the AO8-polyhedron, demonstrates the steric restriction of the direct linkage of two Eux-modules.
The influence of the local heteropolyhedral substitutions on the topological features of the parental crystal structures has been shown previously (Aksenov et al., Reference Aksenov, Yamnova, Kabanova, Volkov, Gurbanova, Deyneko, Dimitrova and Krivovichev2021b; Reference Aksenov, Kabanova, Chukanov, Panikorovskii, Blatov and Krivovichev2022b). In the case of ixiolite–euxenite polysomatic series, the euxenite- and samarskite-type structures are characterised by the following tile sequences of the cationic 3D nets: [34]2[32.42]4[34.42]2[38] and [34]2[32.42]6[34.42]3[38], respectively. The tiles [32.42] and [34.42] are common for the all members of the polysomatic series.