Introduction
Recurrent implantation failure (RIF) is defined as the failure to achieve clinical pregnancy in adult women under 40 years of age after the transfer of at least three high-quality embryos within three fresh or frozen cycles, including day 3 embryos (≥8 cells, uniform blastomere size, fragmentation rate < 10%), and blastocyst (≥3BB) (Chinese Association of Reproductive Medicine and Professional Committee of Reproductive Medicine, China Medical Women’s Association, 2023). A diverse range of etiological factors may lead to RIF, including unhealthy lifestyle (smoking and obesity), poor embryo quality (especially in older women), thrombosis status, uterine factors (such as congenital uterine malformations, endometrial polyps, submucosal fibroids, uterine adhesions), and adjuvant factors (e.g., hydrocele in the fallopian tube). Patients with RIF typically fail to conceive despite extensive clinical examinations and all possible treatments, which poses a clinical challenge.
In a previous study, repeat implantation failure was found to be an independent factor affecting the implantation rate; the implantation rate decreased as the number of implantation failures increased (Wang et al., Reference Wang, Tian, Liu, Li, Tong, Zhu and Zhang2021c). Therefore, more attention should be paid to embryo transfer (ET) strategy for patients with RIF. The strategy should aim to reduce the number of ET cycles to improve pregnancy outcomes and shorten the time to conceive (Walsh et al., Reference Walsh, Shkrobot, Coull, Peirce, Walsh, Salma and Sills2009; Wang et al., Reference Wang, Sun, Wang, Zhang and Hu2010).
Unlike preimplantation genetic testing, selecting an embryo at the cleavage stage according to the morphological grading alone cannot screen out embryos with genetic and chromosomal abnormalities (Fragouli et al., Reference Fragouli, Alfarawati, Spath and Wells2014). Preimplantation embryo development is a sequential and complex process; the key events during this process include mitotic cell division, compaction, and formation of the blastocyst cavity. In addition, the embryonic gene activation at the 4-cell to 8-cell stage is a marked transition during embryo development (Nothias et al., Reference Nothias, Majumder, Kaneko and DePamphilis1995; Sozen et al., Reference Sozen, Can and Demir2014). Studies have shown that 50–80% of embryos at the cleavage stage have one or more blastomeres that are aneuploid (Vanneste et al., Reference Vanneste, Voet, Le Caignec, Ampe, Konings, Melotte, Debrock, Amyere, Vikkula, Schuit, Fryns, Verbeke, D’Hooghe, Moreau and Vermeesch2009). Furthermore, embryos with abnormal chromosomes and poor developmental potential are highly likely to experience developmental stagnation (Braude et al., Reference Braude, Bolton and Moore1988; Sfakianoudis et al., Reference Sfakianoudis, Maziotis, Karantzali, Kokkini, Grigoriadis, Pantou, Giannelou, Petroutsou, Markomichali, Fakiridou, Koutsilieris, Asimakopoulos, Pantos and Simopoulou2021). Available evidence suggests that blastocyst transfer is the more desirable choice; extending embryo culture allows the natural selection of embryos, therefore resulting in significantly better pregnancy outcomes than cleavage ET (Glujovsky et al., Reference Glujovsky, Farquhar, Quinteiro Retamar, Alvarez Sedo and Blake2016; Practice Committee of the American Society for Reproductive Medicine, 2017).
Embryo cryopreservation, a well established technique, enables the preservation of embryos not selected for immediate transfer and preserves biopsied embryos for genetic testing before pre-ET (Bosch et al., Reference Bosch, De Vos and Humaidan2020). In addition, the freeze-all strategy can prevent ovarian hyperstimulation syndrome, which is useful for fertility preservation (Stern and Agresta, Reference Stern and Agresta2019). Furthermore, several studies have shown a notable enhancement in clinical pregnancy rates through frozen ET (FET) compared with fresh ET (Sik et al., Reference Sik, Oral, Aba, Ozolcay, Koc and Sismanoglu2020). This divergence can be linked to the credible influences of ovarian stimulation and the subsequent elevation of supraphysiological sex steroid levels within fresh ET cycles. These factors can potentially induce modifications in endometrial receptivity, thereby compromising trophoblast invasion and the process of placental formation. Contrastingly, the endometrial preparation in the FET cycle more closely mimics the natural pregnancy cycle (Fauser and Devroey, Reference Fauser and Devroey2003; Chen et al., Reference Chen, Shi, Sun, Zhang, Liang, Cao, Yang, Liu, Wei, Weng, Tian, Hao, Yang, Zhou, Shi, Xu, Li, Yan and Qin2016). Some studies have reported that frozen–thawed cleavage-stage embryos, which did not undergo further division in the first 24 h after thawing, are not recommended for transfer because most of these embryos may have chromosome aberrations (Laverge et al., Reference Laverge, Van der Elst, De Sutter, Verschraegen-Spae, De Paepe and Dhont1998; Maurer et al., Reference Maurer, Ebner, Puchner, Mayer, Shebl, Oppelt and Duba2015). However, the pregnancy outcome of thawed blastocyst transfer was found to be superior to that of the cleavage ET in patients with repeated transfer failures; this distinction is likely to be attributable to a synergy between the process of natural selection during blastocyst development and an enhanced synchronization between the blastocyst and the endometrium (Wang et al., Reference Wang, Zhao, Ma, Zhu and Wang2021b; Ozturk, Reference Ozturk2023).
Furthermore, the vitrification and thawing processes can also cause mechanical damage, radical oxygen damage, and abnormalities in gene methylation and acetylation, therefore reducing the embryo’s potential to develop into blastocysts (Truong and Gardner, Reference Truong and Gardner2020). At our Fertility Center, the blastocyst culture technique was initially not widely used or used only upon patient request, and only cleavage embryos were used for cryopreservation. Most of the patients achieved pregnancy through the embryo freezing–thawing technique, but some of the patients were still infertile after more than three transfers of cleavage embryos. Based on our experience, we found that in this group of patients, thawing the remaining embryos and prolonging the culture to blastocysts resulted in better pregnancy outcomes.
In this study, we investigated the benefit of extending the culture time of vitrified/thawed embryos in RIF patients by comparing three different FET strategies: thawed D3 embryos, thawed D5 blastocysts, and D5 blastocysts resulting from thawed D3 embryos culture. We found that culturing thawed D3 embryos to D5 blastocysts reduced the number of transfer cycles, shortened the time to live birth, and was a promising FET strategy for RIF patients for whom only cryopreserved cleavage stage embryos were available.
Materials and methods
Data collection
This was a retrospective study of infertile couples who had undergone vitrified–thawed ET cycles at the Maternal and Child Health Care Center of Yulin between January 2018 and August 2020. This study was approved by the Ethics Committee of the Yulin Maternal and Child Health Care Hospital. All participants were informed about the study details and their written consent was obtained before the study.
Data pertaining to the following variables were collected: age, body mass index (BMI), duration of infertility, type of infertility, infertility factors, number of repeat implantation failures, medication regimen, endometrial thickness, and number of embryos transferred. The number of embryos thawed, number of intact embryos, number of surviving embryos, implantation rate, hCG-positivity rate, clinical pregnancy rate, miscarriage rate, multiple-birth rate, and live-birth rate were also calculated.
The inclusion criteria were: (i) maternal age < 38 years; (ii) failure to conceive after more than three consecutive in vitro fertilization (IVF) treatments with good quality embryo transfer; and (iii) endometrial thickness ≥ 8 mm on the day of ET. Patients who had fewer than two cryopreserved embryos, or those who had been diagnosed with hydrosalpinx, endometriosis, or uterine fibroids were excluded from this study.
Patient group
During this study period, two ET strategies were used at our centre: the cleavage stage ET and blastocyst ET. However, women who failed to conceive after three cleavage-stage ETs were advised to try a prolonged culture FET cycle (D3–D5), in which vitrified day 3 embryos were thawed and cultured in vitro for 48 h, and the resulting day 5 blastocysts were then transferred.
Overall, 276 FET cycles were performed on patients who qualified the above criteria. There were 58 cycles of prolonged culture of vitrified day 3 embryos, but 10 cycles were cancelled for ET because of failure of development of vitrified embryos into blastocysts. Finally, FET cycles were classified into three groups according to the transferred embryos: vitrified–warmed D3 cleavage-stage ET (D3 FET) group (n = 52 cycles); vitrified–warmed D5 blastocysts transfer (D5 FET) group (n = 166 cycles); and the prolonged culture FET (D3–D5 FET) group (n = 48 cycles).
Embryo vitrification and warming protocols
Vitrification was used for embryo cryopreservation of day 3 cleavage embryos (<25% fragmentation) and day 5 blastocysts. The vitrification device used was Cryotop (Kitazato, Tokyo, Japan), combined with the commercial vitrification medium (VT601, Kitazato, Tokyo, Japan). The vitrified embryos were warmed using the Kitazato Warming Kit (VT602, Kitazato, Tokyo, Japan). The vitrification and warming protocols were as per the instructions provided by the manufacturer, which have been described in a previous study (Le et al., Reference Le, Nguyen, Nguyen, Dang and Nguyen2021).
No more than two embryos could be warmed at each FET cycle for the routine day 3 embryo or day 5 blastocyst warming procedure. For the prolonged culture group (days 3–5), the previously vitrified cleavage embryos were warmed and cultured in vitro for 48 h. However, no more than two resulting day 5 blastocysts could be transferred, and the remaining day 5 blastocysts were preserved by vitrification (Kitazato, Tokyo, Japan). In addition, embryos that developed into blastocysts on day 6 were vitrified, and the FET cycles were cancelled. The results of extended embryo culture were recorded for each individually cultured embryo.
Embryo culture and embryo selection
All warmed embryos were cultured in a tri-gas incubator at 37°C in an environment of 6% CO2, 5% O2, and 90% N2. For D3 FET and D5 FET cycles, vitrified–warmed cleavage embryos and blastocysts were cultured in vitro for 2 h prior to the transfer. For D3–D5 FET cycles, cleavage embryos were cultured extensively for 48 h after warming, and the resulting day 5 blastocysts were transferred. If the blastocyst formed on day 6, it was preserved and the patient was classified as having not completed the transplant cycle. The warmed cleavage embryos without damaged blastomeres were considered intact, and only those embryos that developed into blastocysts were transferred. The surviving embryo, developed from a 6-cell cleavage embryo, was approved immediately after warming.
According to the Istanbul Consensus, the cleavage embryo grading system considered the number of embryos, number of fragments, uniformity, and arrangement of cell size, cytoplasmic colour, and pronuclei. The usable thawed cleavage stage embryos included both good and medium quality embryos. Good quality embryos contained 8–9 cells, had <10% fragmentation, an embryo size consistent with the developmental stage, and no multiple pronucleus phenomenon. Medium quality embryos contained >6 cleavage cells, had 10–25% fragmentation, most cells of a size consistent with the developmental stage, and no multiple pronucleus phenomenon. Poor embryonic characteristics included <6 cleavage cells, >25% fragmentation, cell size inconsistent with the developmental stage, and multiple pronucleus phenomena.
The viability of frozen–thawed blastocysts was predicted based on the expansion of the blastocyst cavity within a few hours (usually 2–6 h) after recovery. The quality of the thawed blastocyst was graded according to the Gardner scoring system (Gardner and Schoolcraft, Reference Gardner and Schoolcraft1999). A blastocyst with either the inner cell mass or trophoblast cells graded as B was defined as a usable blastocyst. In addition, good quality blastocysts were defined as inner cell mass and trophoblast cell grade ≥ B when they reached stage 3 or above. Good quality blastocyst rate was defined as the number of good quality blastocysts divided by the number of stage II and above blastocysts. The integrity rate was defined as the number of intact recovered embryos divided by the total recovered embryos for all the thawed embryos.
For the extended culture of thawed day 3 embryos, day 5 or day 6 blastocyst formation was observed and marked as D5 blastocyst and D6 blastocyst, according to the timing of blastocyst formation. If more than three embryos developed into blastocysts on day 5, the maximally developed two blastocysts were transferred, while the rest were vitrified again. To estimate the embryo development potential in the prolonged culture procedure, the utilization rate was defined as the number of usable blastocysts (including the number of transferred and re-vitrified embryos) divided by the number of thawed embryos.
Endometrium preparation and embryo transfer
Endometrial preparation for ET involves the utilization of three distinct regimens: hormone replacement cycles, spontaneous cycles, and pre-ovulatory cycle protocols. For spontaneous cycles, endometrial and follicular development was monitored by ultrasound starting on day 10 of menstruation. When large follicles reached 18–24 mm in diameter and endometrial thickness was ≥ 8 mm (day 0), luteal support was given (on day 0 and day 3 for D3 FET, and day 5 for D5 FET). For the hormone replacement regimen, oral estradiol valerate (Tonicare, Bayer Pharmaceuticals, Germany) was administered starting on days 2–3 of menstruation. When endometrial thickness was ≥8 mm, progesterone injection (20 mg/stem, Zhejiang Xianju) was administered for luteal support (on day 0 and day 3 for D3 FET, and day 5 for D5 FET). Ovulation stimulation was administrated on days 3–5 of menstruation: oral letrozole tablets were prescribed for 5 days, along with optional human menopausal gonadotropin (HMG) for combined ovulation stimulation. Progesterone was then used to convert the endometrium when the dominant follicle was ≥17 mm and the endometrial thickness was ≥8 mm. The chosen embryos were introduced into the uterine cavity with ultrasound guidance, employing the COOK-K-JETS-7019 catheter manufactured by COOK Medical based in Indiana. This standardized catheter was consistently used for all cycles, ensuring methodological uniformity and minimizing potential variations introduced using different catheters.
Clinical outcomes
Pregnancy outcomes included biochemical, clinical pregnancies, clinical pregnancy rate, implantation rate, multiple pregnancy rate, abortion rate, and live-birth rate. The level of beta-hCG was measured on the 14th day after cleavage-stage ET in the D3 FET group or on the 11th day after a blastocyst transfer in the D3–D5 FET and D5 FET groups. hCG positivity was defined as beta-hCG level > 50 mIU/ml. Clinical pregnancy was diagnosed when a gestational sac and fetal cardiac activity were present, 4 weeks after ET. The implantation rate was calculated as the number of gestational sacs per transferred embryo at 6 weeks of pregnancy.
The miscarriage rate was defined as the number of miscarriage cycles divided by clinical pregnancy cycles. The multiple-birth rate was calculated as the number of cycles of multiple pregnancies divided by the number of clinical pregnancy cycles. The live-birth rate was defined as the number of cycles divided by clinical pregnancy cycles.
Statistical analysis
Power analysis was conducted using G*Power version 3.1.9.7 to determine the appropriate sample sizes for our study. For the F-test using analysis of variance (ANOVA) repeated measures between factors, we set the effect size (f) to 2.5, the alpha error probability to 0.05, and the power (1-beta error probability) to 0.8. Based on the analysis, we determined that a sample size of 159 cycles would be sufficient. Additionally, we conducted a power analysis for the chi-squared test using goodness of fit. In this analysis, we set the effect size (w) to 0.3, the alpha error probability to 0.05, the power to 0.95, and the degrees of freedom (df) to 2. The analysis showed that a sample size of 172 was required to meet the statistical requirements of our study. All statistical analyses were performed using SPSS 25.0 (SPSS Inc., Chicago, IL). Categorical variables were described as the frequency (percentage)of the category; between-group differences were assessed using the chi-squared test. Continuous variables are presented as mean ± standard deviation (SD); Student’s t-test and one-way ANOVA were used to assess the differences between three groups, with the relevant post-hoc test for multiple comparisons. P-values < 0.05 were considered indicative of statistical significance.
Results
As shown in Table 1, there were no significant differences between the three groups with respect to the baseline characteristics, such as age, BMI, years of infertility, infertility factors, number of previous implantation failures, medication regimen, or endometrial thickness.
Table 1. Essential characteristics of the study population
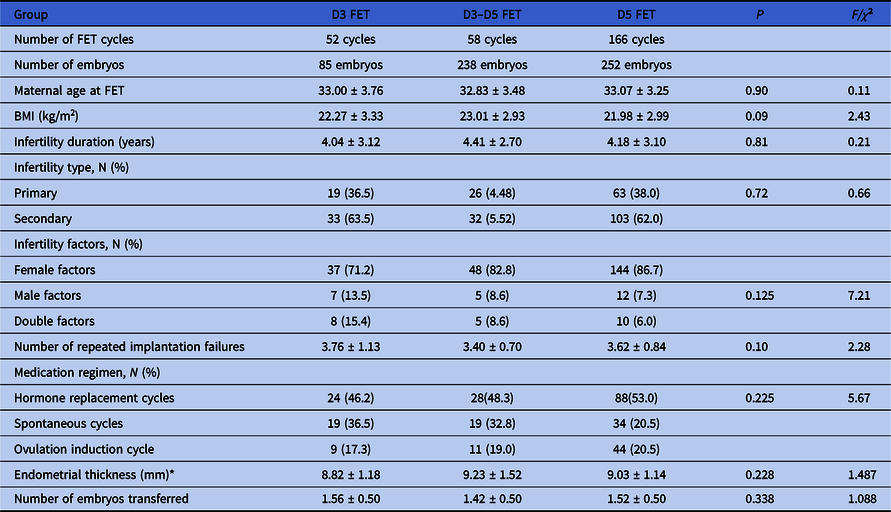
FET: Frozen embryo transfer. All continuous variables are expressed as mean ± standard deviation.
* The D3–D5 FET group excluded 10 cases having no D5 blastocyst transferred.
The embryo resuscitation rate (viable thawed embryos) and embryo integrity rate (intact thawed embryos) in the D5 FET groups were higher than those in the D3 and D3–D5 FET groups; however, there was no significant difference between D3 and D3–D5 groups in this respect. Interestingly, the good quality rates of the thawed embryo were comparable among the three groups. Notably, when considering the number of thawed embryos, the usable embryo rate and transferred embryo rate were lowest in the D3–D5 FET group. Only 44.5% of the thawed D3 embryos were usable after extending in vitro culture to D5 blastocysts, and only 28.6% of thawed embryos were transferred in this group. In contrast, the highest rates of usable thawed embryos (100%) and transferred embryos (100%) were in the D5 FET groups (Table 2).
Table 2. The quality of cryopreserved/thawed embryos among the three groups

FET: Frozen embryo transfer.
* The number of usable blastocysts following the extended in vitro culture of thawed day 3 embryos.
a P < 0.05: D3 FET vs. D3–D5 FET.
b P < 0.05: D3 FET vs. D5 FET.
c P < 0.05: D3–D5 FET vs. D5 FET.
Pregnancy outcomes during the transplant cycle in the three groups are shown in Table 3. Miscarriage rates were comparable among the three groups; however, the D3–D5 FET group showed significantly improved pregnancy outcomes compared with the D3 FET group, in terms of higher hCG-positivity rates (P < 0.05), implantation rates (P < 0.05), clinical pregnancy rates (P < 0.05), and live-birth rates (P < 0.05). Moreover, the implantation rate and live-birth rate were doubled in the D3–D5 FET group compared with the D3 FET group. Compared with the D5 FET group, the D3–D5 FET group was found to have a better hCG-positivity rate and implantation rate, although the clinical pregnancy rates were similar. In addition, the multiple pregnancy rate was also higher in the D3–D5 FET group than in the D5 FET group (Table 3).
Table 3. Pregnancy outcomes of FET cycles in the three groups

FET: Frozen embryo transfer.
a P < 0.05: D3 FET vs. D3–D5 FET.
b P < 0.05: D3 FET vs. D5 FET.
c P < 0.05: D3–D5 FET vs. D5 FET.
The therapeutic effect analysis of the three transplantation strategies is shown in Table 4. Among the three methods, the transplantation cycle cancellation rate in the D3–D5 FET group was significantly higher than that in the other two groups (P < 0.05). Compared with the D3 FET group, the D3–D5 group had a significantly higher hCG-positivity rate and live-birth rate (P < 0.05 for both), indicating significant improvement in pregnancy outcomes. Pregnancy outcomes in the D3–D5 FET group and the D5 FET group were similar in terms of hCG-positivity rate, implantation rate, clinical pregnancy rate, and live-birth rate (Table 4).
Table 4. Analysis of the therapeutic efficiency of the three methods

FET: Frozen embryo transfer.
a P < 0.05: D3 FET vs. D3–D5 FET.
b P < 0.05: D3 FET vs. D5 FET.
c P < 0.05: D3–D5 FET vs. D5 FET.
Discussion
The purpose of this study was to investigate the optimal FET strategy for RIF patients who failed to conceive after three consecutive cleavage-stage ETs. We compared three different embryo thawing strategies for FET and found that compared with traditional D3 FET, the D3–D5 FET strategy significantly improved the hCG-positivity rate and live-birth rate. In contrast, the D3–D5 FET strategy had a similar hCG-positivity rate, implantation rate, clinical pregnancy rate, and live-birth rate compared with traditional D5 FET. Therefore, our results suggest that prolonged in vitro culture of post-thawed day 3 embryos is the optimal FET strategy for RIF patients with only cryopreserved cleaved embryos. Our findings are in line with previous studies. Le et al. (Reference Le, Nguyen, Nguyen, Dang and Nguyen2021) investigated the effectiveness of blastocyst transfer following extended culture from warmed cleavage-stage embryos for patients who had failed previous cleavage-stage ET cycles. They found that extended culture and blastocyst transfer significantly improved FET pregnancy outcomes compared with cleavage-stage embryo transfer. Gorrill et al. (Reference Gorrill, Kaplan, Patton and Burry1999) evaluated the viability and transfer efficiency of cryopreserved embryos that developed into blastocysts in extended culture for in vitro fertilization. They found that acceptable pregnancy rates are achievable by transferring these blastocysts with a significantly increased implantation rate. Holden et al. (Reference Holden, Kashani, Morelli, Alderson, Jindal, Ohman-Strickland and McGovern2018) and Aytac and Kilicdag (Reference Aytac and Kilicdag2022) compared the clinical outcomes of vitrified–thawed cycles during IVF by frozen and thawed blastocysts compared with cleavage-stage embryos that were frozen, thawed, and underwent extended culture to the blastocyst stage. They found that the use of extended blastocyst culture of cleavage-stage embryos was not inferior to frozen and thawed blastocysts. Freezing at the cleavage stage can yield similar cryo-survival rates when compared with blastocyst vitrification. Vitrifying surplus or all embryos for storage at the cleavage stage allows higher implantation and clinical pregnancy rates. However, after abortion, live-birth rates were similar in both groups (Aytac and Kilicdag, Reference Aytac and Kilicdag2022).
Morphological observation alone is not a good predictor of the subsequent embryo developmental potential as the embryos with chromosomal abnormalities are morphologically indistinguishable from euploid embryos. Chromosomal abnormalities have been reported in most cases of miscarriage in humans (Benkhalifa et al., Reference Benkhalifa, Kahraman, Biricik, Serteyl, Domez, Kumtepe and Qumsiyeh2004). A previous study showed that maternal effector genes have a critical role in the early embryogenesis of model organisms (Yu et al., Reference Yu, Yi, Gao, Qin, Zhai, Chen, Ou-Yang, Wang, Zheng, Zhu, Wang, Sun, Dean and Li2014). In addition, 90% of chromosomal abnormalities are maternal in origin, mainly attributed to female age-related chromosomal errors (Baltaci et al., Reference Baltaci, Satiroglu, Kabukçu, Unsal, Aydinuraz, Uner, Aktas, Cetinkaya, Turhan and Aktan2006; Chiang et al., Reference Chiang, Schultz and Lampson2012). Studies have shown that a high dose of gonadotropins (Baltaci et al., Reference Baltaci, Satiroglu, Kabukçu, Unsal, Aydinuraz, Uner, Aktas, Cetinkaya, Turhan and Aktan2006; Chiang et al., Reference Chiang, Schultz and Lampson2012) can result in an increased risk of aneuploidy rates in embryonic and granulosa cells (Bosch et al., Reference Bosch, Labarta, Kolibianakis, Rosen and Meldrum2016). Extending the in vitro culture from cleavage-stage embryos to blastocysts may be a natural selection, as the embryo’s development undergoes some key events such as activation of the embryonic genome, compaction, cavitation, and blastocyst formation (Kojima et al., Reference Kojima, Tam and Tam2014). Another previous study suggested that day 5 blastocysts are healthier and carry a lower risk of being aneuploid (Glujovsky et al., Reference Glujovsky, Farquhar, Quinteiro Retamar, Alvarez Sedo and Blake2016; Wang et al., Reference Wang, Zhao, Ma, Zhu and Wang2021b). This finding may explain the improved pregnancy outcomes following Day 3–5 FET compared with Day 3 FET in our study.
Under natural physiological conditions, the embryo divides and develops mainly in the fallopian tube, followed by its transport to the uterine cavity upon developing into a morula or blastocyst. The synchronization between embryo development and endometrial transformation enables embryo implantation (Chiang et al., Reference Chiang, Schultz and Lampson2012). Conversely, it is well known that the human endometrium is receptive to embryo implantation during a limited time window in the menstrual cycle, which is known as the window of implantation (WOI) (Matsumoto, Reference Matsumoto2017). According to a meta-analysis, single blastocyst transfers were associated with higher rates of sustained pregnancy and live birth than single cleavage-stage embryo transfers, but the evidence quality was deficient (Li et al., Reference Li, Liu and Lv2021b). Studies have also shown that blastocysts developed from poor quality embryos can improve the cumulative pregnancy rate of patients, obviating the need for patients to undergo repeat IVF–ICSI treatment due to poor quality embryos (Kaartinen et al., Reference Kaartinen, Das, Kananen, Huhtala and Tinkanen2015).
Moreover, another study showed that extending the bad quality cleavage-stage embryo culture to the blastocyst stage can result in successful implantation and clinical pregnancy, although the blastulation rate was lower (Hershko Klement et al., Reference Hershko Klement, Ovadia, Wiser, Berkovitz, Shavit, Nemerovsky, Ghetler, Cohen and Shulman2017). Similarly, extending the culture of the frozen–thawed embryos with low blastomere numbers to blastocysts was also shown to improve the pregnancy outcomes (Li et al., Reference Li, Huang, Li, He, Wang, Zhang, He, Kang, Shi, Chen and Wang2021a). Consistent with these findings, our study also indicated that blastocyst transfer, including D3–D5 FET and D5 FET, can result in better pregnancy outcomes when compared with the D3 FET.
Notably, our cohort exclusively comprised patients with RIF who had three failed consecutive cleavage embryo transfers. A recent study compared the pregnancy outcomes of cryopreserved day 3 embryos FET with cryopreserved blastocysts FET in RIF patients and suggested that cryopreserved/thawed single blastocyst transfer (SBT) may be the preferred strategy (Zhang et al., Reference Zhang, Gao, Liu, Liu, Wu, Xiong, Zhu, Han, Wang, Hao, Han and Huang2021). Similarly, we found that the day 5 FET group had better pregnancy outcomes in RIF patients than the day 3 FET group. Furthermore, in our study, although only 44.5% of the post-thawed day 3 embryos developed into available blastocysts on day 5, the resulting blastocysts caused significantly higher pregnancy rates than direct transfer post-thawed day 3 embryos. Interestingly, in a study by Rahav-Koren et al. (Reference Rahav-Koren, Inbar, Miller, Wiser, Yagur, Berkowitz, Farladansky-Gershnabel, Shulman and Berkowitz2021) when compared with day 5 blastocysts FET, culturing post-thaw cleavage embryos for 2 days and transferring the resulting blastocysts increased the chances of a viable pregnancy and delivery per transferred embryo. In contrast, Aytac and Kilicdag (Reference Aytac and Kilicdag2022) found that the extended culture of cleavage-stage embryos to blastocyst was not inferior to frozen and thawed blastocysts in terms of cryo-survival rate (96.7% versus 95.0%). However, implantation rates (28.9% versus 22.4%, P = 0.04) and clinical pregnancy rates (37.2% versus 27.9%, P = 0.03) were higher in cleavage-stage embryos with extended blastocyst culture (Aytac and Kilicdag, Reference Aytac and Kilicdag2022). Consequently, they suggested that vitrification of surplus or all embryos for storage at the cleavage stage and extending the culture to blastocysts after thawing may help achieve higher implantation and clinical pregnancy rates than the thawed blastocysts; however, live-birth rates were similar in both groups (Aytac and Kilicdag, Reference Aytac and Kilicdag2022). Although the vitrification protocol can eliminate ice crystal formation, the potential damage of ice crystal formation is due to insufficient permeation of cryoprotectant inside the blastocoele, and the potential osmotic shock caused by exposure to the high concentration of cryoprotectant can also affect the embryo survival and consequent implantation rate (De Vos et al., Reference De Vos, Van Landuyt, Santos-Ribeiro, Camus, Van de Velde, Tournaye and Verheyen2016; Boyard et al., Reference Boyard, Reignier, Chtourou, Lefebvre, Barrière and Fréour2022). Therefore, the process of cryopreservation and thawing at a cleavage stage, followed by 2 days of additional culture in fresh medium, can avoid potential vitrification damage on expanded blastocysts, which could be a better FET strategy compared with D5 FET. However, in our study, pregnancy rates and live-birth rates were similar in the D3–D5 FET and the D5 FET groups. Previous studies have suggested that blastocysts, although mechanically damaged by ice crystals during the freeze–thaw process, have artificial crinkling techniques applied to reduce the size of the blastocyst cavity and decrease the extent of damage, therefore keeping their pregnancy and live-birth rates from decreasing. Similarly, with an extended culture process, embryos differentiated to blastocyst period to repair the damage during freezing and thawing, and also maintained high pregnancy and live-birth rates. The similar pregnancy and live-birth rates for blastocyst transfer may be explained by the interaction of endometrium with blastocysts, which are composed of inner cell mass and trophectoderm cells. During this process, a small amount of damage may be repaired during further development of blastocysts after implantation.
However, we found that a subset of patients in the D3–D5 FET group had to cancel their FET cycle because of lack of development of blastocysts from post-thawed Day 3 embryos, while none of the patients in the D3 FET or D5 FET groups cancelled their cycle. Nevertheless, previous studies have shown that the number of failed implantations is an independent predictor of the success of assisted pregnancy, with pregnancy rates decreasing with an increase in the number of transplantations (Quinn and Kerin, Reference Quinn and Kerin1986; Wang et al., Reference Wang, Tian, Liu, Li, Tong, Zhu and Zhang2021c). Furthermore, in our study, D3–D5 FET groups showed higher implantation, pregnancy, and live-birth rates despite cancelled cycles. Therefore, we considered that discarding the bad quality embryos that did not develop into blastocysts would not adversely affect the final pregnancy outcome of patients with RIF. However, some patients had a larger number of blastocysts developed from post-thaw day 3 embryos, and we then performed re-freezing of the excess blastocysts by vitrification. Wang et al. showed that repeated cryopreservation is harmful to embryos and suggested that repeated freezing and thawing should be avoided (Wang et al., Reference Wang, Jiang, Xi, Li, Ren, Li, Zhu and Jin2021a).
In contrast, Hallamaa et al. (Reference Hallamaa, Seikkula, Willman, Ollila and Jokimaa2021) found that the transfer of twice-cryopreserved embryos did not compromise the live-birth rates and neonatal outcomes. To avoid embryo wastage, good quality surplus embryos from FET may be cryopreserved again by vitrification. However, more attention should be paid to the effect of repeated freezing and thawing processes on embryo quality, and future studies should investigate the value of repeated cryopreservation on embryo quality and pregnancy outcomes, especially in RIF patients.
At the same time, it should be noted that reduced transfer cycles and shorter live-birth times can be achieved by extended in vitro culture after resuscitation for blastocysts in RIF patients for whom only cryopreserved cleavage-stage embryos are available. Although 43.8% of vitrified–thawed cleavage embryos developed into blastocysts in the D3–D5 FET group, an additional 56.2% of D3 embryos that did not successfully develop into blastocysts were discarded, which may be considered to have resulted in patient embryo waste. However, as we described previously, due to the selection of embryos based on morphology, the arrest of embryo development to blastocyst, and the damage caused by freeze–thaw process, these embryos may have developed defects that were not known and may already have lost their potential to continue to develop themselves, which if not eliminated may become an obstacle to achieving good pregnancy outcome. While it is true that freezing the embryos introduces a delay in transferring them to a subsequent cycle, we observed that this initial delay was outweighed by the subsequent benefits of culturing the thawed D3 embryos to D5 blastocysts. Culturing the embryos to the blastocyst stage allows for better embryo selection, as only the embryos that successfully develop to the blastocyst stage are transferred. Blastocyst transfer also allows for more accurate timing of embryo development and uterine receptivity. These factors collectively contribute to an increased likelihood of achieving a successful pregnancy and ultimately lead to a shorter time to live birth. Our results show that blastocyst transfer obtained by extended in vitro culture can achieve similar pregnancy outcomes as with D5 blastocyst transfer, which is significantly higher than that in repeatedly failed patients who continue to receive D3 embryo transfer. These results also suggest that extended in vitro culture to obtain blastocyst transfer is effective in improving pregnancy outcomes in RIF patients for whom only cleaved embryos are available, with reduced transfer cycles and shorter time to conceive. In contrast, continuation of the transfer of the remaining cleavage embryos in RIF patients is liable to lead to delayed treatment, increased treatment costs, and wastage of resources due to repeated patient attempts.
Embryo implantation entails a dynamic interplay between the embryo and the endometrial intima. In the context of assisted reproduction, diverse endometrial preparation schemes, including artificial cycle (AC), natural cycle (NC), and stimulation cycle (SC), are implemented, each offering distinct advantages. However, recent studies have revealed an increased risk of early miscarriage associated with the use of the AC for endometrial preparation before frozen ET (FET), a trend that is also observed with the natural and stimulation cycles. As a potential means of improving artificial cycles, researchers have explored individualized hormone replacement therapy (Vinsonneau et al., Reference Vinsonneau, Labrosse, Porcu-Buisson, Chevalier, Galey, Ahdad, Ayel, Rongières, Bouet, Mathieu d’Argent, Cédrin-Durnerin, Pessione and Massin2022). Of note, a comparative analysis of various endometrial preparation schemes showed limited effect on pregnancy outcomes (Chen et al., Reference Chen, He, Song, Li, Liu, Li and Xing2007). In this study, our specific focus was on the AC, NC, and SC protocols, with a criterion of endometrial thickness greater than 8 mm for transplantation. We hypothesize that greater intima thickness results in a more abundant blood flow, potentially benefiting embryo implantation. Nevertheless, gaining a comprehensive understanding of the intricate relationship between endometrial thickness, blood flow, and embryo implantation success necessitates further in-depth exploration, which we acknowledge as a limitation of this study.
The influence of ET catheters on both embryo implantation and patient pregnancy rates is a critical consideration. Several reports have identified potential influencing factors, such as endometrial damage, embryo retention, catheter occlusion, and inaccurate placement (Saravelos and Li, Reference Saravelos and Li2019). Although this study did not explicitly address the influence of catheter selection and manipulation on clinical outcomes, we acknowledge the significance of these factors. While our study did not reveal adverse outcomes linked to the COOK-K-JETS-7019 catheter, we recognize that a comprehensive analysis of these factors would enhance our understanding of overall success rates. These considerations will guide the focus of our forthcoming research.
Some other limitations of this study should also be acknowledged. First, this was a single-centre retrospective study. Prospective studies and randomized controlled trials (RCTs) are required to obtain more robust evidence of the value of D3–D5 FET. The second limitation is the small sample size compared with previous studies. Third, although we only focused on FET cycles, the different methods used for preparing the endometrium may have introduced an element of bias. Finally, we also noted that the formation of viable blastocysts in the D3–D5 group after extended culture was 44.5%, but only 28.6% of blastocysts were transferred, and the remaining blastocysts underwent re-freezing, with 16.0% of high-quality blastocysts transferred. In future studies, we should reduce the number of embryos in extended culture, reduce the number of re-frozen blastocysts, and optimize the culture process to obtain a high rate of good quality blastocysts.
In conclusion, our findings propose two potentially valuable transfer strategies for patients experiencing repeated implantation failures. The D3–D5 FET approach presents a more significant potential for selecting promising embryos in cases without blastocyst preservation; however, this strategy does entail the risk of cycle cancellation. Conversely, in instances in which blastocyst preservation is an option, prioritizing consideration of the D5 FET strategy is recommended.
Availability of data and material
The datasets used and/or analyzed during the current study are available from the corresponding author upon reasonable request.
Acknowledgements
We thank Medjaden Inc. for editing and proofreading this manuscript.
Author contribution
All authors contributed to the study’s conception and design. XL, YZ, and JH performed material preparation, data collection and analysis. XL, and all authors commented on previous versions of the manuscript. All authors read and approved the final manuscript.
Funding
This research received no specific grant from any funding agency, commercial or not-for-profit sectors.
Competing interests
Authors declare no Conflict of Interest for this article.
Ethics approval
This study was approved by the Ethics Committee of Yulin Maternal and Child Health Care Hospital.
Consent to participate
All participants were informed about the study details, and their written informed consent was obtained before the study.
Consent for publication
Not applicable.