Single-Shot Three-Dimensional Electron Imaging
École Polytechnique Fédérale de Lausanne, Switzerland
Developers: Emad Oveisi and Cecile Hebert
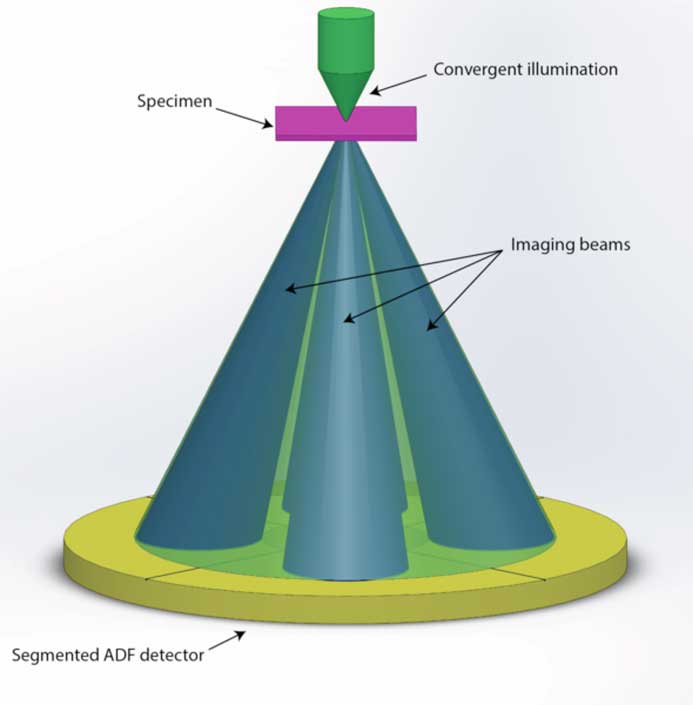
This new method for 3D imaging in the TEM promises to be more efficient in both acquisition time and electron dose compared to conventional TEM tomography techniques. The method is an integrated approach to stereo imaging in the scanning TEM (STEM) using segmented detectors and algorithmic feature reconstruction. By considering the solid cone of incident electrons that forms the STEM probe as consisting of parallel rays of different illumination angles, opposing sets of these convergent rays are selected within the direct beam of the diffraction pattern to image the sample stereographically. Both rays are recorded simultaneously using a new segmented STEM detector.
Compared to other 3D imaging methodologies, this simultaneous imaging technique has the advantages of faster acquisition speed and limited electron beam exposure for studying beam-sensitive materials. In addition, it eases the 3D imaging of crystalline defects under well-defined diffraction contrast conditions. In fact, for a crystal orientation where a systematic row of reflections is excited, choice of opposing incidence angles perpendicular to the direction of the diffracted beams corresponds to the same excitation condition. By selecting the intensities of two counterparts on opposing sides of the (000) direct beam disc for image formation, a pair of stereo images may be recorded with exactly the same deviation from the Bragg diffraction condition—an important criterion for imaging a 3D network of crystal dislocations. The reconstruction of the 3D morphology takes place via a proprietary image-processing algorithm. The algorithm uses the prior knowledge of line-shaped objects to bypass the missing wedge limitation of conventional tilt-series tomography, yielding reliable 3D reconstructions even with the limited stereographic tilt angle governed by the convergence angle of the electron probe. Since the specimen remains un-tilted, this method is effectively “tilt-less.” The efficiency of data acquisition and reconstruction is increased by two orders of magnitude compared to standard TEM tomography techniques. By exploiting ongoing instrumental developments in both hardware (new segmented and pixelated detectors, in situ holders, and cryo-STEM), software (faster acquisition), and image processing, this methodology has the potential to transform certain research areas, perhaps making possible real-time 3D imaging of in situ dynamics.
High Numerical Aperture, High Efficiency X-ray Lenses
Deutsches Elekronen-Synchrotron (DESY), Germany
Developers: Saša Bajt, Andrzej Andrejczuk, Sabrina Bolmer, Henry Chapman, Andrew Morgan, and Mauro Prasciolu

The penetrating nature of hard X-rays, photons with wavelengths below 0.1 nm, make them attractive for imaging of objects such as biological cells at resolutions beyond that of visible light and without the need for sectioning or thinning the sample as is the case for transmission electron microscopy. This property of hard X-rays also makes them difficult to focus. Soft X-rays, with wavelengths longer than 0.1 nm, can be focused with thin diffractive Fresnel zone plates (similar to a circular transmission grating), but high aspect ratio diffracting structures are required to focus hard X-rays. This was achieved by forming these structures layer by layer using magnetron sputtering. The lens is sliced from the multilayer structure in a direction perpendicular to the layers to produce what is known as a multilayer Laue lens (MLL). Several improvements were made to the fabrication process to achieve high efficiency and to extend the numerical aperture (NA) beyond what was previously possible. By scanning a small focused spot of hard X-rays, imaging at spatial resolutions below 10 nm is possible with diffraction efficiencies exceeding 80%.
Two MLLs with slightly different focal lengths are used to produce a focused spot, similar to two cylindrical lenses. The lenses are typically 10–100 µm tall (aperture size), 100 µm wide and several µm thick (optical depth) with focal lengths of 1–10 mm. They are fabricated from many tens of thousands of layers. Sharp interfaces between layers were obtained using new materials that do not inter-diffuse. Focusing and imaging with 8.4 × 6.4 nm resolution has been demonstrated. The larger NA and higher efficiency of these lenses allow rapid imaging of thick samples, as needed for tomographic imaging.
Imaging with hard X-rays offers advantages for the study of whole biological cells and of devices such as batteries under operating conditions. The increased penetration of the X-rays can image thicker objects. Even with improved NA, by operating at short X-ray wavelengths, a depth of field larger than the thickness of such objects is achieved, enabling high-resolution three-dimensional tomographic imaging. Shorter wavelengths also reduce the dose to the specimen and allow chemical analysis by X-ray fluorescence of all elements in the periodic table.
Phase Retrieval from the Differential Phase Contrast Signal
HREM Research, Inc
Developers: Akimitsu Ishizuka, Masaaki Oka, and Kazuo Ishizuka

Differential phase contrast (DPC) imaging in scanning transmission electron microscopy (STEM) was proposed by Dekker and de Lang in 1974. A few years later Waddell and Chapman showed that the center of mass (COM) of the diffraction pattern is proportional to the gradient of the phase distribution of a phase object and that a split semicircular electron detector will give an approximate estimate of the COM perpendicular to a bisector of the detector. Recently, the possibility of DPC imaging at atomic resolution was demonstrated using a quadrant detector from which two perpendicular DPC signals may be obtained.
Since the DPC signal is proportional to the gradient of the phase distribution, the phase distribution of the sample can be retrieved by integrating the DPC signal. The new method here uses numerical procedures to obtain the object phase from the two DPC signals. Before the present method, procedures to retrieve a phase distribution from the DPC signal used the Fast Fourier Transform (FFT), which inevitably introduced periodic continuation at the boundaries of its solution (creating an image artifact). The present method uses the discrete cosine transform (DCT) to integrate the DPC signal since the Neumann boundary condition can be directly specified by the DPC signals. Recently a high-speed camera (a pixel detector) became available, and a diffraction pattern now can be obtained at each scan position.
Previous methods to retrieve the phase distribution by integrating the DPC signal used the FFT, which inevitably introduces a slowly varying background (artifact) due to its implicit assumption of the periodic continuation at the image boundaries. The new method uses the Neumann boundary condition, which does not introduce such an artifact.
For many applications, STEM recently has become more popular than conventional transmission electron microscopy because of its versatility. Using STEM we can acquire chemical information simultaneously with structural information from an image. DPC imaging adds to STEM another capability: observation of a phase object using a segmented detector or a pixel detector. The new method gives a boundary-artifact-free phase distribution retrieved from the DPC signal. A DigitalMicrograph plug-in is in development, called qDPC (quantitative DPC), based on the above method.
Relativity ® Compressive Sensing and Multiplexed TEM Video
Integrated Dynamic Electron Solutions, Inc.
Developer: Bryan Reed

Relativity® is a post-specimen electrostatic deflector system that deflects transmission electron microscope (TEM) beams into sub-regions of the TEM camera with a 20 ns inter-frame switching time. This multiplexed image acquisition allows kHz-level frame rates, with nanosecond-precise single-frame exposure times, even using conventional TEM cameras. Each sub-image contains image data to reconstruct a video of the experiment. The deflector provides true compressive sensing of TEM video with effective frame rates 100× faster than the base camera frame rate. The sophisticated temporal sequencing software of Relativity® provides a high degree of control over the timing and duration of beam blanking, multiplexing of exposure patterns, and detailed nanosecond-precise control of up to 8 auxiliary devices including sample holders, lasers, and detectors. The deflector system projects images, diffraction patterns, or EELS spectra onto the camera. Relativity® has been designed to be configurable over a wide range of operating modes and gives users the ability to precisely define where, when, and how long the electron dose is delivered to each sub-region of the detector over the duration of an experiment. Combined with Acuity™ data analysis software (IDES, Inc.), Relativity® acts as the core of an integrated high-speed data acquisition and analysis tool. Relativity® can be installed into any standard 35 mm camera port and requires no significant modifications to the TEM.
The current approach to improving TEM camera frame rates involves incremental advances in image sensor technology. While there are cameras on the market that offer high-frame-rate imaging, the price of those products is often prohibitive and sometimes greater than the cost of the microscopes they are installed on. Relativity® allows similar high frame rates to be achieved with existing scintillator/CCD TEM cameras, while it also can synchronize with newer high-end camera systems potentially enabling multi-kilohertz sustained frame rates.
Key applications of Relativity® include in situ electron microscopy, cryo electron microscopy, and scanning transmission electron microscopy diffraction. The advantages of high-frame-rate imaging for in situ electron microscopy are obvious, however an equally valuable aspect of Relativity® is its ability to serve as the high-speed timing control system for triggering a variety of in situ sample holders and other TEM accessories.
Engineered Materials for Nanoscale Hyper-lensing
US Naval Research Laboratory and Vanderbilt University
Developers: Alexander J. Giles, Joshua Caldwell, and James Edgar

Hexagonal boron nitride (hBN) is a two-dimensional material, similar to graphene in that it consists of atomically thin, planar sheets that are vertically stacked upon each other. Using the imaging response of hBN, earlier work showed that it was possible to resolve objects 32× smaller than the incident imaging wavelength. This achievement was based on the concept of hyperlensing, which is a label-free method for imaging nanoscale objects, of a size below the usual diffraction limit of light. The method requires highly anisotropic materials with permittivities that are opposite in sign along orthogonal axes. The current innovation is a method to drastically improve the material quality through isotopic enrichment, effectively making an isotopically pure material. This affords both tunability and ultra-high-resolution imaging capabilities that were previously unavailable. By controlling the atomic (isotopic) masses in hBN, we observed up to an eight-fold increase in the quality factor of the phonon resonances in this material. This provides several benefits, most importantly higher transmission throughput (lower absorption), but also almost an order of magnitude enhancement in the spatial resolution (~30 nm minimum imaged feature size). With further advances such as coupling with active elements like graphene and expansion of the image to the far-field, this method could provide the first label-free imaging of nano-sized objects using mid-infrared light. This method would also allow electrical control of the depth of field. Further, by imaging with light that is tuned to or off the vibrational resonances of the specimen material, spectroscopic identification would also be possible.
This technique does not require fluorescent labels, vacuum, or cryogenic environments and can be fitted on conventional light optical microscopes. Thus, the isotopically pure material for the hyperlens creates tremendous opportunities in bioimaging since the capability of this system has improved image resolution from 300 nm to 30 nm. Contained within this length scale are viruses, phages, larger proteins, and cellular organelles. Perhaps most importantly, these features can be imaged at room temperature and atmospheric pressure. This innovation holds the promise of enabling the real-time imaging of biological processes.
Quartet™
Neurescence, Inc.
Developer: Yasaman Soudagar

Quartet™ is a miniature microscope system for observing neurons in several areas of the brain and spinal cord over extended periods of time. In operation the researcher tags particular types of neurons in regions of rodent brain and spinal cord with a protein called GCaMP6 that changes its fluorescence level as it binds to calcium 2+ ions flowing in and out of the neurons. A patent-pending connector, containing lenses that employ gradient-index optics, is then surgically implanted at the tagged regions. After a 3-week recovery time for the rodent, a fiber optic probe is connected to each implanted lens. Under software control an LED is turned on, and the illumination level is adjusted for the amount of expression of fluorescence in the imaged regions. The camera is focused by manually turning the screw on the lens-side of the lens-fiber connector. The animal is then released into the experimental setup, and the system will begin saving images at 20 fps in continuous mode. At the end of the imaging session, the fiber optic probes are disconnected from the lenses. When the fiber probe is disconnected, the initial setting of the microscope focus remains the same, assuring that the same plane of neurons is imaged as days go by. Thus, observable changes that come from changes in the neuronal micro-circuitry, and not from focusing on a different plane of neurons, are recorded. Neuronal activity of particular neurons is processed and analyzed by selecting the imaged neurons via the software. The software saves the evidence of neuronal activity, time tags the amount of activity, and labels the particular neuron in a spreadsheet file.
Quartet™ provides monitoring of neuronal activity in multiple imaging regions anywhere in the brain, including its hard-to-reach areas, as well as the spinal cord. The electronics of Quartet™ do not sit on the head of the animal, allowing connection and disconnection of the fibers in an awake mouse, with minimal stress to the animal.
Applications of Quartet™ include the study of neuronal micro-circuitries forming and changing under various conditions. For example, does a drug candidate for Alzheimer’s improve the formation of micro-circuitries that are responsible for memory formation and recall?
Reflective Imaging Improves Spatiotemporal Resolution and Collection Efficiency in Light Sheet Microscopy
National Institute of Biomedical Imaging and Bioengineering
Developers: Yicong Wu and Hari Shroff

This new method uses reflection to improve the spatiotemporal resolution and collection efficiency of light sheet microscopy. The improvement is a result of introducing additional specimen views that carry extra information about the sample. The sample is deposited on a reflective coverslip, instead of a conventional glass coverslip. The reflection (a) introduces an additional light sheet that increases the fluorescence emission rate, improving speed; (b) introduces multiple extra virtual views of the specimen that enhance axial resolution as the views are normally not “seen” by the microscope; and (c) increases collection efficiency since fluorescence that is usually “lost” to the coverslip can now be captured. In addition to this concept, new deconvolution methods were invented that combine the extra information gleaned from reflection and reduce out-of-focus fluorescence that would otherwise contaminate images.
Reflective coverslips are commercially available and are easily substituted for conventional coverslips, so the method can be straightforwardly applied to existing light sheet microscopes. The method can also be used to generate higher numerical aperture (NA) views that would normally be difficult to obtain.
The utility of the method has been demonstrated on a dual-view inverted selective plane illumination microscope (diSPIM), achieving an effective quadrupling of speed relative to single-view microscopy and a four-fold enhancement in collection efficiency. These advantages allowed us to image calcium flux at an unprecedented volumetric rate (~3 volumes/s), allowing us to “catch” transients in rapidly moving worm embryos. We also demonstrated the improvement in volumetric resolution and collection efficiency that would occur at higher NA, obtaining high-resolution datasets within single cells and worm embryos.
Fluorescence microscopy is an enabling method in cell biology because of the superb specificity it affords. Light sheet fluorescence microscopy is a further advance that much better uses the available photon budget compared to a confocal or widefield fluorescence microscope. However, the usefulness of any fluorescence microscope is limited by the available photon signal. By capturing more views of the specimen without introducing additional delay, the reflective cover slip fundamentally improves on light sheet fluorescence microscopy, extending the value of the limited photon budget by improving resolution and information content within the images.
MUSE Microscopy
University of California Davis Health
Developers: Richard Levenson, Farzad Fereidouni, and Stavros Demos

MUSE microscopy (microscopy with ultraviolet surface excitation) is based on two properties of 280 nm UV light: (1) UV light in this range penetrates tissue specimens to a depth of no more than about 10 microns, a thickness only slightly greater than that of a standard histology slide tissue section; and (2) UV light at this wavelength can excite, and cause to emit in the visible range, a large variety of inexpensive fluorescent stains that can mimic (and extend) the tissue specificity of hematoxylin and eosin (H&E) stain. The low penetration depth limits excitation to a thin layer and removes the usual requirement for tissue sectioning. Using color conversion algorithms, the resulting fluorescent images can be converted, in real time, to resemble that seen with conventional pathology microscopy.
The design is based on UV LEDs, conventional glass (not UV-transparent) objectives, and color cameras. There is no need for lasers, emission or excitation filters, or the computational input required by other methods. Sample preparation is straightforward, consisting of a brief (seconds) stain of tissue and a brief wash.
Light microscopy for tissue diagnostics, the industry standard, typically relies on thin-sectioned formalin-fixed specimens and absorbance-based stains (such as H&E) that are useful for general morphological visualizations. But those stains provide only a limited color gamut that reveals little about the molecular constitution of the sample. Moreover, thin-cut specimens on slides represent a narrow and flat planar slice of a complicated 3D sample. MUSE overcomes both of these limitations. First, a number of rapidly applied fluorescent tissue stains can generate multiple and potentially useful color signals that convey more information than is available with H&E. Secondly, since MUSE views the surfaces of tissues, it can capture 2.5-dimensional topographical information.
There is no substitute for tissue sampling (biopsies) followed by histopathology. The light microscopy methods used to examine these biopsies have not changed significantly in over a century. MUSE can replicate, and in some cases improve upon, conventional transmission light microscopy, but its ability to eliminate the need for histological slide-preparation is likely to be of greater significance. MUSE has the potential to fundamentally change how diagnostic microscopy is practiced.
Gradient Light Interference Microscopy (GLIM)
University of Illinois at Urbana-Champaign
Developer: Gabriel Popescu

Gradient light interference microscopy (GLIM) exploits a special case of low-coherence interferometry to extract phase information from the specimen, which in turn can be used to measure cell mass, volume, surface area, and their evolutions in time. Because it combines multiple images that correspond to controlled phase shifts between two interfering waves, gradient light interference microscopy is capable of suppressing the incoherent background due to multiple scattering.
The GLIM module is designed as an add-on connected to the output port of an inverted light microscope. A Wolllaston prism generates two replicas of the image field. The module shifts the phase of one component four times using a spatial light modulator (SLM) while keeping the other component unmodified. Interference patterns generated by these two components are recorded and transferred to a computer for phase-gradient extraction. Four frames are acquired by the GLIM module, one for each phase shift applied by the SLM. Using these images, a phase-gradient map is obtained, which then is integrated along the direction of the shift to recover a quantitative phase map.
GLIM provides a label-free and quantitative method to map the refractive index of live biological cells and tissues. Compared to other methods, GLIM uses a low-coherence white-light source instead of a laser, producing a speckle-free background, and works at a much lower irradiance, reducing light-induced damage to live cells. The method also can render cells in 3D. Furthermore, this method can be overlaid with other imaging modalities, such as fluorescence or bright-field microscopy.
In an early application, GLIM has been used to measure the growth of embryonic bodies in 3D without adding any fluorescent dyes or tags to the cells. GLIM can show a clear high-resolution reconstruction of embryonic bodies by using the phase delay as the (intrinsic) contrast agent, without damaging or disturbing the sample. Moreover, due to its imaging speed, the method can study both fast 3D dynamics, such as transport and diffusion in cells, and also slow 3D dynamics, such as cell growth or migration. GLIM can potentially become a valuable tool for in vitro fertilization, where contrast agents and fluorophores may impact the viability of the embryo.
Mochii S
Voxa
Developer: Christopher Own

The Mochii S is a miniature portable scanning electron microscope (SEM) with an integrated energy-dispersive X-ray spectrometer (EDS); it is the size of a coffee maker and weighs about 6 pounds. The wireless user interface allows Mochii S to be remotely operated from across the room or from distant locations. An Apple iPad is employed to control the instrument, process data, and send results by email. Connecting via the cellular network, the internet, or satellite uplink, several users can translate the sample and capture images and EDS spectra. In the future, researchers traveling to Antarctica or the Sahara to search for meteorites, for example, could bring Mochii S along to image and analyze samples immediately upon discovery. After sample loading, such deep-field researchers could turn over operating control to colleagues elsewhere in the world to continue analysis from thousands of miles away.
When the Mochii S electron source needs replacement, the user replaces the entire electron column in a single quick-change operation. Its 10 kV accelerating voltage and array detector for backscattered electrons provide useful imaging up to 5,000×. This SEM runs on 110/240 VAC, consumes under 85 W, and pumps down in as little as 3 minutes. The automated 2-axis specimen stage can handle specimens up to 20 mm × 20 mm × 15 mm. The Mochii S is low in cost; and, an optional integrated metal coater further reduces costs by eliminating the need for a typical stand-alone EM accessory.
Mochii S is scheduled to launch to the International Space Station in 2019 as a microgravity research platform. However, NASA found that SEM/EDS analysis on the ground has been critical in understanding equipment failures aboard ISS, and the use of Mochii S onboard ISS would reduce decision-making time and increase ISS efficiency. For example, in 2013 a crew member detected a water leak in his helmet during an extravehicular activity (EVA). NASA suspended all EVAs until, more than 6 months later, ground-based SEM/EDS analysis found the cause of the leak to be aluminum silicate debris in the helmet’s fan pump separator. With Mochii S onboard ISS, such analyses could be done as soon as a failure is detected, enhancing crew and vehicle safety.