Introduction
The apicomplexan parasite Toxoplasma gondii (T. gondii) infects one-third of the world's population, disproportionately affects socio-economically disadvantaged groups, and ranks among five neglected parasitic infections targeted in the USA by the Centers for Disease Control (CDC) for public health action [Reference Jones, Parise and Fiore1, 2]. Some estimates indicate that in the USA, ~170 000 new toxoplasmosis cases occur annually and that 1.1 million are currently infected, amounting to infection among 13.2% (age-adjusted: 12.4%) of the population > 6 years of age [Reference Jones3]. Other sources note that although >40 million men, women and children in the USA may harbour the parasite without symptoms because the immune system prevents the occurrence of the full-blown illness, consequences of infection can be severe for the immunocompromised and for women infected during or shortly before pregnancy [2].
The burden of T. gondii infection by foodborne routes, which is estimated to account for about half of all US cases, exceeds $3.6 billion and equates to the loss of 459 481 quality-adjusted life days [Reference Minor4]. According to Scallan et al. (2011), 2.6% of US-acquired foodborne T. gondii infections will result in hospitalisations, and 0.02% will result in death, leading to an estimated 327 deaths annually, making toxoplasmosis the second leading cause of foodborne illness fatality in the USA [Reference Scallan5].
Sexual reproduction of T. gondii occurs in its definitive felid hosts, including cats, while asexual reproduction occurs in intermediate hosts, including humans and nearly all warm-blooded vertebrates. The parasite has three infectious stages [Reference Dubey, Lindsay and Speer6–Reference Torrey15]: sporulated oocysts that contain sporozoites, tachyzoites that invade and multiply rapidly within non-intestinal epithelial cells of the definitive hosts and in any cell of intermediate hosts , and bradyzoites that slowly replicate in tissue cysts (life cycle detailed in Fig. 1). Tissue cysts are most often found in the central nervous system; the eyes; and smooth, skeletal or cardiac muscles. While bradyzoites in tissue cysts characterise chronic infection, tachyzoites characterise primary acute or reactivated infections, which can trigger adaptive IgG immune responses [Reference Hill, Chirukandoth and Dubey7, Reference Montoya and Liesenfeld8]. In reactivation, tissue cysts rupture and release bradyzoites that transform back into destructive tachyzoites [Reference Robert-Gangneux and Darde11, Reference Sutterland13]. Toxoplasmosis may present in immunocompetent individuals with painless lymphadenopathy; mononucleosis-like symptoms, including fever, malaise, sore throat and maculopapular rash; ocular infections in some older populations; or no symptoms in pregnant women [Reference Montoya, Boothroyd, Kovacs, Bennett, Dolin and Blaser9, Reference Dubey and Jones12]. Severe clinical manifestations, such as pneumonitis, chorioretinitis or multi-organ involvement, occur most often in reactivated and primary infections among immunocompromised individuals and in congenital infections [Reference Montoya, Boothroyd, Kovacs, Bennett, Dolin and Blaser9, Reference Derouin and Pelloux10]. HIV-positive individuals often present with life-threatening toxoplasmic encephalitis, characterised by headache, confusion, weakness, focal neurologic involvement and seizures [Reference Robert-Gangneux and Darde11, Reference Dubey and Jones12]. Previously, chronic infections were not associated with clinical manifestations [Reference Montoya, Boothroyd, Kovacs, Bennett, Dolin and Blaser9], but recent studies have linked chronic infection to schizophrenia [Reference Sutterland13–Reference Torrey15] and suicidal behaviour [Reference Arling16, Reference Pedersen17].
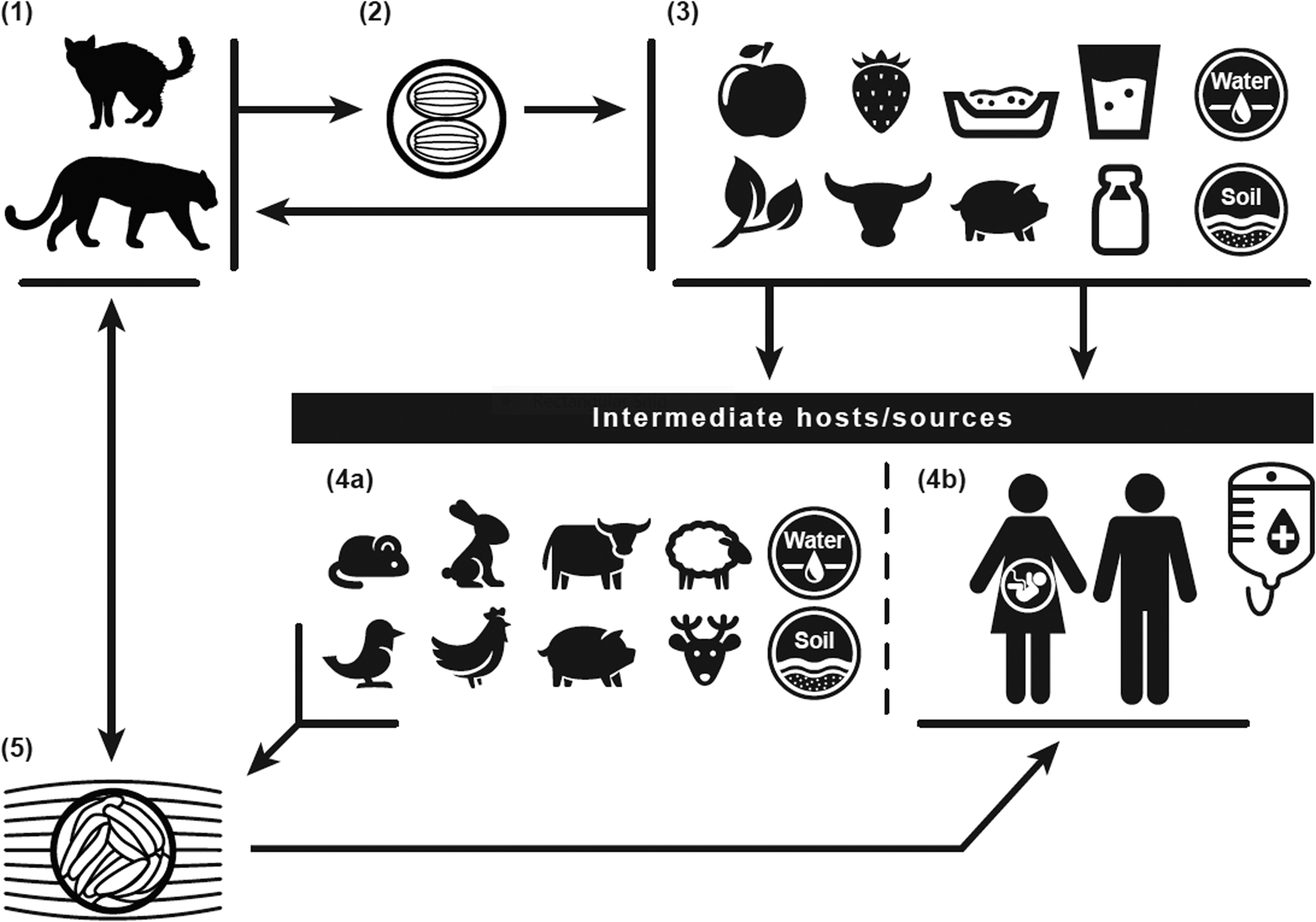
Fig. 1. Toxoplasma gondii life cycle: The definitive felid hosts, including house cats (1), uptake the Toxoplasma gondii parasite through ingestion of tissue oocysts (5) from infected intermediates, such as rodent and bird prey, or other sources, including water (4a). Following uptake, the definitive felid hosts shed unsporulated oocysts for up to 3 weeks through feces (2), contaminating water, soil, kitty litter, and food sources (3) of intermediate hosts/sources (4a/4b). The unsporulated oocysts distributed through the definitive felid host feces take 1 to 5 days to become infective. Intermediates include the definitive felid host prey, human food sources, water, and soil (4b). Humans can contract infection through intake of contaminated food or water; contact with contaminated soil or kitty litter; blood transfusions or organ transplants; or maternal to fetal (vertical/placental) transmission.
Multiple behavioural, socio-economic and demographic factors may contribute to the risk of toxoplasmosis. Studies focusing on behavioural risk factors often use a combination of immunoassays to determine infection and questionnaires to conduct an epidemiologic assessment of potential exposures. While the questionnaires themselves are often tailored to specific populations, many ask similar questions regarding behaviour-related exposures: most notably, eating different meats [Reference Said18–Reference Jones22] (porcine, bovine, lamb/mutton, other); handling or eating raw or undercooked meat [Reference Said18, Reference Jones22]; consuming dairy/dairy products [Reference Jones22–Reference Retmanasari24]; eating unwashed or improperly washed fruits and/or vegetables [Reference Jones22, Reference Wam25, Reference Zhang26]; gardening and/or engaging in contact with soil [Reference Said18, Reference Nash21, Reference Jones22, Reference Zhang26]; living with pets, such as cats and/or dogs at home [Reference Said18, Reference Bamba19, Reference Zhang26]; drinking water (treatment, source, other characteristics) [Reference Bamba19, Reference Jones22, Reference Messier27]; and even hunting/consuming wild game (venison, boar, other) [Reference Said18, Reference Messier27]. In the USA, those below the federal poverty threshold have higher T. gondii seropositivity [Reference Jones3], while higher early-life socio-economic status has been shown to be related to seronegativity [Reference Meier28]. Other risk factors for higher seroprevalence, specifically in the USA, include foreign country of birth, Hispanic and non-Hispanic Black race/ethnicity, lower than high school education levels, crowded housing conditions, rurality/urbanicity and employment type [Reference Jones3, Reference Jones22].
The Old Order Amish (OOA) population in the USA, by virtue of its homogeneous characteristics, provides a unique opportunity to assess relationships between T. gondii seropositivity and specific behavioural and environmental (including food-related) risk factors. T. gondii seropositivity prevalence levels among the OOA exceed those found among the general US population [Reference Hill29], and the geographic, ethnic and socio-economic homogeneity of the OOA population [Reference Kraybill, Johnson-Weiner and Nolt30] helps to control the potential confounding that can otherwise complicate risk factor assessment. In addition, the Amish lifestyle presents the prospect of evaluating potential associations between T. gondii seropositivity and culturally specific meal-preparation practices, such as slaughtering, smoking, curing and canning meats [Reference Cuyun Carter31]. These distinctive characteristics, in conjunction with antibody testing and data collection using OOA lifestyle-specific questions, allowed this study to pursue the objective of advancing understanding of relationships between the prevalence of T. gondii seropositivity and behavioural and environmental (including food-related) risk factors of infection, while minimizing confounding by other potential socio-demographic and behavioural determinants.
Methods
Study population
The Amish Wellness Study
The Amish Wellness Study (AWS), an ongoing community-based investigation conducted by the University of Maryland Baltimore and approved by the University of Maryland Baltimore Institutional Review Board, was established in 2010 to offer health screening and collection of DNA, blood samples and basic medical information to address specific research questions. Criteria for participation included membership in the OOA community of Lancaster County, Pennsylvania; age of ⩾18 years; and informed consent. A registered nurse obtained multiple clinical measures of general health (e.g., weight, height, waist and hip circumference, blood pressure, measures of respiratory health). An Amish liaison facilitated administration of the study questionnaire in person to assess several aspects of each participant's health and well-being, including mood, sleep, and medical, personal and family histories; as well as to evaluate lifestyle factors. A University of Maryland Amish Research Clinic research nurse confirmed completion of each questionnaire. A fasting blood sample was drawn for the measurement of lipids and glucose, and from this sample, we also measured T. gondii IgG antibodies for this study.
For the present study, we sent the Amish Toxoplasma Risk Factor Questionnaire (ATRFQ) to a convenience sample from the AWS (n = 3551, non-randomly selected, based on availability to participate), between 30 December 2015 and 3 January 2016. We evaluated participant responses to the ATRFQ, along with their demographic information and T. gondii antibody results from the AWS.
Immunoglobulin G serointensity and seropositivity testing
For this study, the laboratory of Maureen Groer at the University of South Florida in Tampa determined T. gondii antibody seroprevalence using the enzyme-linked immunosorbent assay (IBL International, Männedorf, Switzerland) that tests for RH factor common to all T. gondii serotypes. Concentrations of ⩾12 IU/ml were defined as seropositive, and of 8–12 IU/m as equivocal. The equivocal samples were tested a second time, and if the second testing yielded samples that tested positive for concentrations of ⩾12 IU/ml on the second test, they were also considered positive as previously reported [Reference Duffy32].
Amish Toxoplasma Infection Risk Factor Questionnaire
The ATRFQ was developed by researchers at the University of Maryland Baltimore with input from epidemiologists at FDA's Center for Food Safety and Applied Nutrition (CFSAN) and co-authors based on current risk factor literature and subject matter expertise. The questionnaire was adapted after incorporating input from three sessions of review and feedback by a group of Amish liaisons, three sessions of feedback by nurses experienced in working with the Amish, and individual researcher comments and questions to maximise comprehension and cultural sensitivity. The questionnaire consisted of 30 questions focusing primarily on food consumption and preparation, as well as other environmental risk factors, such as water sources, pet ownership and contact, and contact with soil. The pet component specifically asked about cat/kitten ownership, cat breeding, cat litter cleaning, where the cats live (outdoors vs. indoors), where the cats/kittens eat, type of food they eat, visits of not-owned cats, type of cats seen in the garden or yard, cats for breeding, feral cats (domesticated and returned to the wild), and stray cats (lost or abandoned). It was mailed to study participants between 30 December 2015 and 3 January 2016 with a cover letter, response envelope and US$2 compensation. The cover letter informed participants that they could keep the $2 even if they decided not to participate. Responses from the returned questionnaire received by 11 April 2016 were entered into an Access database. Returned envelopes received after 11 April 2016 were not included in the analytical sample used for this study.
Statistical analyses
The analytical sample included those with both T. gondii serological measures and ATRFQ responses. Analyses were performed to describe the analytical sample (Table 1) and to assess the strength and significance of associations with examined behavioural and environmental (including food-related) risk factors through models estimating the odds of T. gondii seropositivity (Tables 2–4). In addition to age and sex derived from the parent AWS survey, the ATRFQ variables addressed contact with soil, pets (including cats and litter boxes) and shoes/feet before touching food; farm vs. other-than-farm residence and time at residence; drinking untreated or treated water and drinking-water source/filtration; working with animals/raw meat/horses; consuming locally-produced, cooked, cured, frozen, canned, raw and/or rare meats; consuming raw oysters; consuming unpasteurised goat and/or cow milk/products; washing produce; washing hands/utensils after raw meat contact; and eating outside the home.
Table 1. Demographic characteristics of Old Order Amish participants, Toxoplasma gondii serostatus and risk factor study, 2015–2016

s.d., Standard deviation; T. gondii, Toxoplasma gondii.
a P value from χ 2 test of independence for age and sex [Reference McDonald33]; from SAS 9.4 for t test; italics denote significance at P < 0.05.
b T. gondii titre concentration: seropositive ⩾12 IU/ml; seronegative <12 IU/ml.
Table 2. Bivariate analysis of unadjusted associations (unadjusted odds ratios) of serostatus and category-based behavioural risk and protective factors of Old Order Amish participants, Toxoplasma gondii serostatus and risk factor study, 2015–2016

Italics denote significance at P < 0.05.
a Only one respondent indicated raw ground pork intake.
b Only two respondents indicated consumption of unpasteurised goat milk products; 421 indicated intake of unpasteurised cow milk products.
Table 3. Bivariate analysis of associations (unadjusted odds ratios) of serostatus and frequency-based behavioural risk and protective factors of Old Order Amish participants, Toxoplasma gondii serostatus and risk factor study, 2015–2016

Italics denote significance at P < 0.05.
Table 4. Adjusted associations (adjusted odds ratios) of Toxoplasma gondii seropositivity and age and behavioural risk and protective factors by sex of Old Order Amish participants, Toxoplasma gondii serostatus and risk factor study, 2015–2016

Italics denote significance at P < 0.05.
a Only one respondent indicated raw ground pork intake; results, therefore, not shown.
b Only two respondents indicated consumption of unpasteurised goat milk products; 421 indicated intake of unpasteurised cow milk products.
Bivariate analyses, including χ 2 and t tests, compared seropositive to seronegative to identify significant or borderline significant (P value <0.05 and <0.20) variables. For those variables, we then specified comparison reference groups and estimated unadjusted odds ratios (UORs), 95% confidence intervals (CIs) and P values (Tables 2 and 3). We included the variables that exhibited significance in the bivariate UOR analysis (P value <0.05) in the final unconditional multiple logistic regression models by sex to estimate adjusted odds ratios, 95% CIs and P values, evaluating the associations between each of the predictor variables and the outcome of T. gondii seropositivity as defined above (Table 4). These analyses were performed using SAS 9.4 (Cary, NC, USA), as well as the χ 2 calculator in McDonald (2014) [Reference McDonald33]. We also adjusted the results for relatedness (heritability) and shared households by applying a variance component approach using SOLAR 6.6.2 software (San Antonio, TX, USA) to assess significance (P value <0.05) [Reference Almasy and Blangero34].
Results
Of the 3551 questionnaires mailed to study participants, 1518 (43%) were returned. Toxoplasma gondii serology was assessed in 55.5% (n = 843) of those who returned questionnaires. We limited our analytical sample to the 843 Amish subjects with both serology test results and questionnaire response data (23.7% of the 3551 who were mailed the questionnaire also had serology results).
More than half (56.7%) of the 843 study participants tested positive for T. gondii IgG antibodies (Table 1). Nearly two-thirds of participants (60.1%) were female among both the seropositive and seronegative groups. The age distribution/n was similar among seropositive individuals, but not among seronegative individuals or the whole study sample. Mean and median ages were higher among seropositive than among seronegative individuals, and there were more seropositive than seronegative males.
In addition to sex, the χ 2 test results from the first step of the bivariate analysis indicated significant/borderline significant associations between T. gondii seropositivity (P values <0.20) and the category-based variables capturing farm vs. other-than-farm residence and time at residence; working with horses; consuming unpasteurised cow milk/products; consuming cured, rare and raw meats; pet contact; and washing hands/utensils after raw meat contact, as well as the frequency-based variables addressing how often unpasteurised cow milk/products, raw meats, rare meats and meals outside the home were consumed.
Of those variables, the UOR estimates from the second step of the bivariate analysis showed significance (P value <0.05) for pet contact, consumption of meat and unpasteurised milk/products, and handwashing behaviours (Table 2), as well as for the frequency of consumption of unpasteurised cow milk/yoghurt, rare meat, raw meat and meals outside the home (Table 3).
The meat-eating behaviours, as well as the two variables addressing the frequency of consumption, were all associated with T. gondii seropositivity. The odds of seropositivity from eating rare and raw meat compared to not eating rare and raw meat were 1.613 (95% CI 1.017–2.560) and 2.192 (95% CI 1.172–4.101), respectively. Only one individual reported eating raw ground pork and was seropositive. Odds of seropositivity comparing the consumption of unpasteurised milk to not consuming raw milk was 1.211 (OR 1.211, 95% CI 1.055–1.391). Among the behaviours related to pets, only cleaning a cat's litterbox was found to be statistically significantly associated with T. gondii seropositivity (OR 2.451, 95% CI 1.167–5.143).
Because of the significant association found between sex and seropositivity in the bivariate analysis, separate models by sex were used to estimate the adjusted associations between the other variables also identified as significantly associated with seropositivity (Table 4). Several of the associations between T. gondii seropositivity and behavioural and environmental (including food-related) risk factors did not retain significance in the adjusted models by sex. However, the separate adjusted models by sex showed that significance persisted nominally for cleaning the litterbox (among men only), eating raw meat (among women only) and washing hands after touching raw meat (protective in women only). Consuming raw goat or cow products, as well as unpasturised cow milk products, weresignificantly associated with seropositivity among women only (OR 1.863, 95% CI 1.086–3.197; OR 1.930, 95% CI 1.127–3.304, respectively, where only two respondents indicated consumption of unpasteurised goat milk products, and 421 indicated intake of unpasteurised cow milk products). Washing vs. not washing hands after touching raw meat was significantly negatively associated with seropositivity among women only (OR 0.462, 95% CI 0.260–0.823). These adjusted significant results were held, even after adjustment for heritability and shared household.
Discussion
This study found that members of the OOA community of Lancaster, Pennsylvania have a high seroprevalence of T. gondii antibodies, with over 56% of study participants testing seropositive, exceeding the levels reported in many other US populations, although similar to levels reported in Java, Indonesia (62.5%) [Reference Retmanasari24] and among the Nunavik Inuit of Canada (59.8%) [Reference Messier27]. The Jones et al.'s (2014) US-based analysis of the 2009–2010 National Health and Nutrition Examination Survey (NHANES) that included a wave of participants aged ⩾6 years found a much lower seroprevalence of 13.2% (95% confidence limit (CL) 11.8–14.5%) prior to adjustment for age and an age-adjusted seroprevalence of 12.4% (95% CL 11.1–13.7%) [Reference Jones3]. As these studies vary in terms of recruitment and analytical methodologies, we suggest caution when interpreting these differences. The uniqueness of our study population may further complicate comparisons – as previously mentioned, the OOA community is an ethnically, behaviourally and culturally distinct group. We are not aware of any studies assessing the seroprevalence of T. gondii in other Amish populations and have found only one study focusing on a similar population. Alvarado-Esquivel et al. (2010) conducted a T. gondii risk factor and seroprevalence analysis among 152 Mennonites in Durango, Mexico, an isolated and mostly rural population of ethnic Germanic descent, with cultural and behavioural aspects that differ from the surrounding communities [Reference Alvarado-Esquivel35]. They found lower seroprevalence (30.3%) among the Mennonites of Durango [Reference Alvarado-Esquivel35], compared to the 56% seroprevalence rate that we have observed in the OOA community of Lancaster, Pennsylvania. Also, the seroprevalence of T. gondii IgG antibodies in the general population of Durango City, Mexico was reported to be 6.1%, which was remarkably less than the seroprevalence in the Mennonite community living in the same city [Reference Alvarado-Esquivel36]. This difference in seroprevalence may relate to diverse factors, including food sources, diet, unique group practices, geographic characteristics and other factors that differ between this Mennonite community and the OOA.
The rural location of residence may contribute to the higher seroprevalence among the OOA compared to other populations. Seroprevalence studies often consider living in a rural environment a risk factor because of the presence in rural settings of potentially infected animals that can transmit the parasite to humans in a variety of ways [Reference Dubey and Jones12]. The finding of higher seroprevalence among rural compared to urban populations is consistent with the results from the UK, Germany and Ireland [Reference Nash21, Reference Wilking37, Reference Taylor38]. In addition, a 2013 study by Muñoz-Zanzi et al. found that children residing on farms in Wisconsin were more likely to be seropositive than those who did not reside on farms [Reference Muñoz-Zanzi, Williams-Nguyen and Belongia39]. In their multivariate analysis of the 1988–94 wave of NHANES, Jones et al. (2001) reported significantly lower seropositivity among non-Hispanic Blacks living in metropolitan areas (>1 million population) (OR 0.74, 95% CI 0.59–0.93) than among non-Hispanic Blacks living in non-metropolitan areas [Reference Jones40]. Yet, the risk of T. gondii infection was not different among residents of metropolitan and non-metropolitan areas for populations of non-Hispanic Whites or Mexican-Americans [Reference Jones40]. Our study population lacked a proper urban counterpart to assess the impact of rurality/urbanicity, so we cannot exclude the possibility that there is simply high seroprevalence in the general study area.
Several of the food- and food safety-related risk factors found to be significantly associated with T. gondii seropositivity in this study are consistent with results from other studies. First, consumption and handling of raw and/or undercooked meats was associated with T. gondii seropositivity for both beef and pork, as previously seen in the literature [Reference Said18, Reference Nash21–Reference Zhang26]. While the practice of cleaning a cat's litterbox was also observed to be a risk factor among males in our study, our findings highlight the importance of the foodborne transmission route for T. gondii. In addition, washing hands after handling raw meat was found protective of T. gondii seropositivity in our study, especially among women. Our findings emphasise the importance of proper food safety behaviours, such as cooking meat to a safe temperature prior to eating/not consuming raw meat, which is effective at diminishing the risk of contracting the parasite. Also, people should avoid accidental contamination by thoroughly washing hands before and after handling meats, and properly washing preparation surfaces and utensils (such as knives) with soap and hot water after use. Washing of hands and/or use of gloves during gardening may also help prevent contamination [2]. Our study also found that consumption of unpasteurised milk products was associated with the risk of seropositivity. While intake of these products provides a possible route of exposure to the parasite [Reference Jones22–Reference Retmanasari24], consumption rates are low among the US population [Reference Jones22, Reference Costard41, Reference Boughattas42], and thus could explain, in part, the increased seropositivity among the Amish.
This study provided novel insights into risk factors for infection with a highly prevalent parasite in a unique US population. Among several strengths, the study population is known for its ethnic and socio-economic homogeneity, which may have helped to minimise confounding by unmeasured variables. Second, response rates were high for both the AWS questionnaire and the ATRFQ. Third, to counteract the possibility that the participants' close family and household aggregation could have produced spurious associations, the study adjusted post-hoc for heritability and household [Reference Duffy32], without any loss of statistical significance for any of the positive findings reported in our study.
Among the study's important limitations, the findings may not be generalisable to other populations in the USA or elsewhere, given the unique characteristics of the culturally distinct OOA [Reference Kraybill, Johnson-Weiner and Nolt30]. In addition, we saw different distributions between age groups, which may be due to cumulative seropositivity and may have biased some of the observed associations. Our study also included only adults (age 18 years or older), which means that we are unable to assess whether infections were acquired during childhood. Consequently, we are unable to access certain risk factors and are also unable to provide recommendations to prevent these potential exposures. This study is also limited by a relatively small sample size given the number of risk factors analysed. Although the high seropositivity in the OOA may have mitigated sample size issues, the low incidence of certain behaviours may have masked the statistical significance of their potential association with seropositivity.
We presented our findings, both formally and informally, to the OOA community in Lancaster, PA. We discussed the different T. gondii risk factors identified in our analysis and specifically addressed the observed sex-based differences. Direct feedback from the OOA community and the findings themselves have and will continue to be used to develop content for future education efforts, including food safety. We plan on applying for grants to develop additional educational materials, train Amish educators and conduct future studies to assess the possible relationships between knowledge and practice among the OAA. For example, future research efforts include developing a study to assess Toxoplasma risk factors and foetal infection, morbidity and mortality, and possibly establishing a longitudinal study to look at a variety of other important genetic, epigenetic, proteomic, clinical and environmental factors, with sampling data for T. gondii in food, soil, water, and farm and domestic animals, as well as neuroimaging and other markers of physical and neuropsychiatric health and functioning.
Conclusion
This study found T. gondii seropositivity among more than half of the participants from a unique OOA community with culturally specific meal preparation practices. The study highlights significant behavioural and environmental (including food-related) risk factors associated with infection, such as consuming undercooked meats and unpasteurised cow milk products, as well as protective behaviours, such as eating meals outside of the home and washing hands after contact with raw meat, showing the importance of proper food safety practices for meal preparation, which is of growing relevance to the consumers in the current general US population as well. Future research will focus on characterizing specific microorganism traits, including serotypes, markers of infection, and genetic, physiological and clinical associations with T. gondii seropositivity, as well as advancing the understanding of education and outreach needed to promote protective behaviours and reduce the burden of toxoplasmosis from foodborne and other routes.
Acknowledgements
The authors thank Gursharon Nijjar, Christopher Chen, Phillip Miljanic, Hassan Yousufi, Nupur Hegde, Winny Mwaura, Hira Mohyuddin, Sonia Y. Postolache, Thea Postolache, Iqra Mohyuddin, Sunghee Flores, Hanna King, Christopher A. Lowry, Lisa A. Brenner, Andrew Hoisington, Jeffrey O'Connell, Amish Liaisons and nurses and staff of the Amish Research Clinic of the University of Maryland, Lancaster, PA, who went out of their way to support this project. The authors also thank the entire Amish community for collaborating in this project. We further would like to thank Dolores Hill, Dietmar Fuchs and Christopher Hunter for their expert advice on this project. We also thank Alexandra Dagdag for help with proofing the article This work was supported by the US Food and Drug Administration through the cooperative agreement FDU.001418 (TTP). Additional funding for this study was provided by the Mid-Atlantic Nutrition Obesity Research Center (NORC) Pilot & Feasibility Project (Postolache, PI), a sub-award of the parent grant P30DK072488 (Simeon I. Taylor, Program Director), and, in part, by the VA Merit Review CSR&D grant 1I01 CX001310-01A1 (Postolache, PI). The views, opinions and findings contained in this article belong to the authors and should not be construed as an official position of the US Food and Drug Administration, the US Department of Veterans Affairs, or the National Institutes of Health.
Data
The data cannot be shared because of the original protocol and Amish consent. A collaborative effort could be considered and interested individuals should contact the corresponding author.
Conflict of interest
None.
Disclaimer
The findings and conclusions in this study are those of the authors and do not necessarily represent the official position of the U.S. Food and Drug Administration (FDA), the US Department of Veterans Affairs, or the National Institutes of Health (NIH).