1. Introduction
Vitamins of the B complex are water-soluble molecules with essential roles in humans. Our previous paper comprehensively summarised the biological properties of vitamins B1, B2, B3 and B5 (Reference Hrubša, Siatka, Nejmanová, Vopršalová, Kujovská Krčmová and Matoušová1). Although there is a recent practical paper on vitamin B12 (Reference Wolffenbuttel, Owen, Ward and Green2), a comprehensive paper on vitamin B12 is missing.
Vitamin B12, cobalamin, with a molecular weight of 1355.4 Da, is indispensable for humans as an integral part of two biochemical reactions: the conversion of l-methylmalonyl coenzyme A to succinyl coenzyme A, and the formation of methionine by methylation of homocysteine. Humans, as well as animals and plants, are unable to synthesise it. Therefore, we have to obtain vitamin B12 from food(Reference Flippo and Holder3–Reference Smith, Warren and Refsum6).
The compound was first identified as a nutrient or external factor in the 1920s thanks to the research efforts of Minot, Murphy and Whipple, who showed that the symptoms of pernicious anaemia can be overcome by adding liver to the diet. The structure of the compound was solved by Dorothy Hodgkin’s pioneering X-ray crystallography, which revealed that the vitamin was cyanolated, amidated, tetrapyrrole-containing cobalt. As a result, it was called cyanocobalamin(Reference Smith, Warren and Refsum6,Reference Martens, Barg, Warren and Jahn7) .
The structure of vitamin B12 is shown in Figure 1. The cobalt is located in the middle of a circular contraction of a modified tetrapyrrole macrocycle coordinated by four nitrogen atoms. This centre of the molecule is known as the corrin ring and is similar to, although quite different from, the tetrapyrrole-derived ring systems found in haem and chlorophylls. The lower nucleotide loop is bound to the corrin ring by a side chain attached to a macrocyclic ring that contains an unusual natural base 5,6-dimethylbenzimidazole, which also coordinates the cobalt ion. Thus, in cyanocobalamin, the cobalt ion is ligated not only by the four pyrrole nitrogens of the central ring, but also by the upper (β) and lower (α) ligands. The β-ligand in the molecule of B12 is a cyano group, while the α is nitrogen from the mentioned dimethylbenzimidazole. In biological systems, the upper cyano ligand is usually replaced by an adenosyl group to form adenosylcobalamin (more precisely, 5′-deoxyadenosylcobalamin, AdoCbl), or a methyl group to form methylcobalamin, or a hydroxyl group to form hydroxocobalamin. Similarly, some species use a different lower base where dimethylbenzimidazole is replaced by bases such as adenine, substituted benzimidazoles, which include hydroxy- or methoxybenzimidazole, or phenolic compounds, including phenol or cresol(Reference Smith, Warren and Refsum6,Reference Martens, Barg, Warren and Jahn7) .
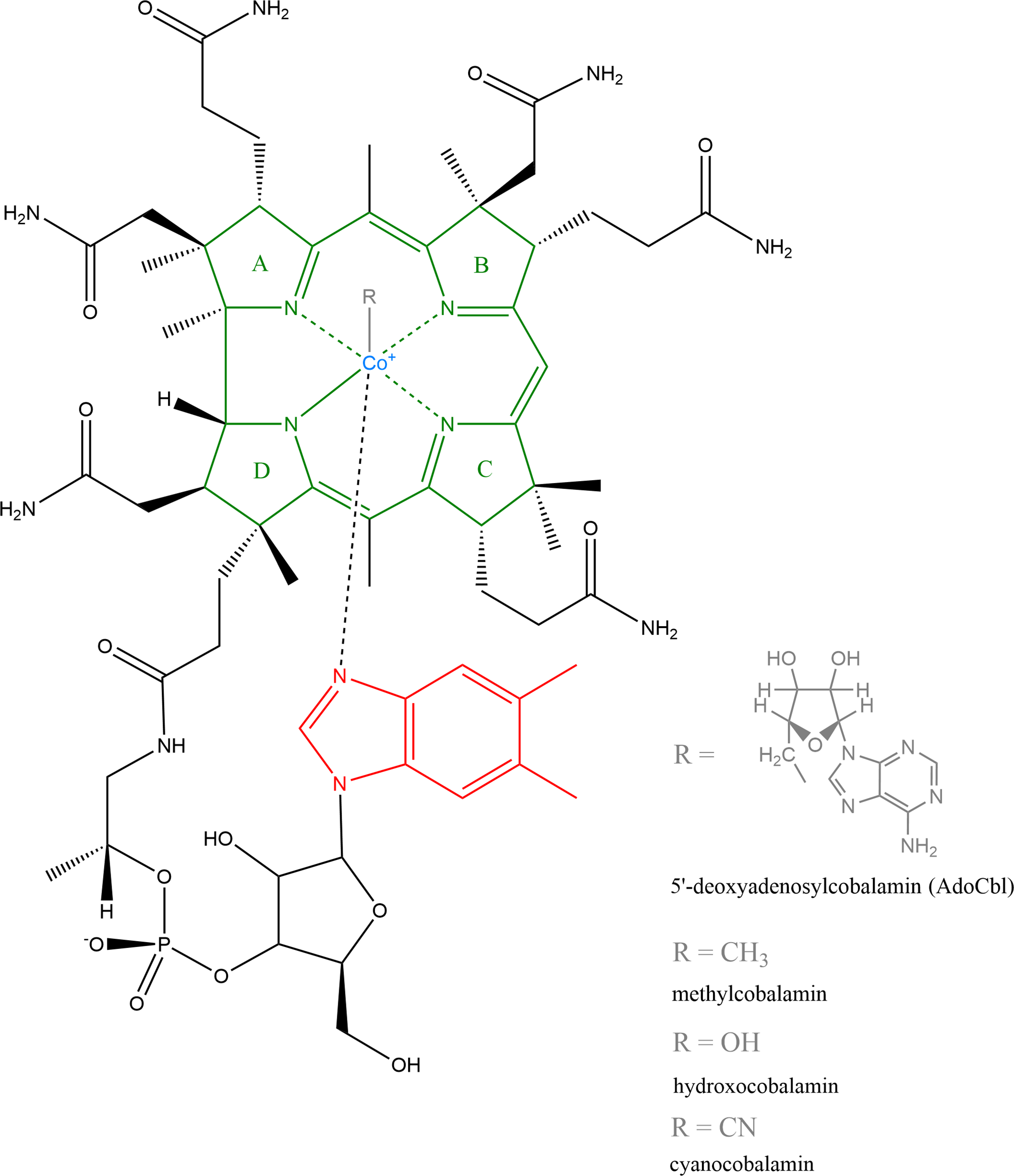
Fig. 1. Structure of vitamin B12: Natural forms include 5′-deoxyadenosylcobalamin (AdoCbI), methylcobalamin and hydroxocobalamin, industrially produced is cyanocobalamin(Reference Martens, Barg, Warren and Jahn7). Structure was created by ChemDraw, version 20.0.
2. Sources of vitamin B12
Eukaryotes, encompassing plants, algae, protists, fungi, animals and humans, do not synthesise vitamin B12 (Reference Smith, Warren and Refsum6–Reference Orłowska, Steczkiewicz and Muszewska22). Vitamin B12 is biosynthesised exclusively in prokaryotes. However, only about a third of all bacteria and archaea species are able to synthesise it(Reference Yilmaz and Walhout8,Reference Warren, Raux, Schubert and Escalante-Semerena9,Reference Kennedy and Taga23–Reference Scott38) . These species provide it for other cobalamin non-producing bacteria and archaea(Reference Sokolovskaya, Shelton and Taga30,Reference Lauridsen, Matte and Lessard39–Reference Doxey, Kurtz and Lynch51) as well as for eukaryotes, except plants and fungi which do not use vitamin B12 as an enzyme cofactor(Reference Smith, Warren and Refsum6,Reference Martens, Barg, Warren and Jahn7,Reference Sanudo-Wilhelmy, Gomez-Consarnau, Suffridge and Webb17,Reference Roth, Lawrence and Bobik18,Reference Sokolovskaya, Shelton and Taga30,Reference Osman, Cooke and Young52–Reference Bertrand, Allen and Dupont58) .
Vitamin B12 is also produced by microbiota in the large intestine of humans, but it is not utilisable by the human body as it is not spatially bioavailable since the intrinsic factor-mediated absorption of cobalamin occurs in the upper part of the gastrointestinal tract (i.e. upstream of the location of its synthesis) as will be described in the following text related to pharmacokinetics(Reference Smith, Warren and Refsum6,Reference Sokolovskaya, Plessl and Bailey59–Reference Biesalski69) . Moreover, a major proportion of the microbially produced vitamin B12 is utilised by other non-vitamin-producing microbes, further limiting its possible availability for the human host(Reference Degnan, Taga and Goodman60,Reference Biesalski69–Reference Kundra, Geirnaert and Pugin76) . The latest observational study in adult humans reveals a slight colonic absorption of cobalamin (approximately 7%) and speculates about the potential involvement of the colonic microbiome in the body’s vitamin B12 homoeostasis(Reference Kurpad, Pasanna and Hegde77). However, the mechanism of vitamin B12 uptake from the colon and the overall contribution of the ‘endogenously produced’ colonic vitamin B12 to the maintenance of vitamin B12 status remain unknown(Reference Fedosov78). Based on the current knowledge, it seems that humans are mostly dependent on vitamin B12 from exogenous sources.
In animals, the vitamin B12 from prokaryotes is obtained and stored in tissues through microbial interactions in the natural food chain. A good example are ruminants, such as cattle and sheep. They are herbivores, i.e. they feed on pasture that does not contain cobalamin, but they obtain the essential vitamin B12 through symbiotic relationship with microbes inside their body. They have a specialised digestive organ positioned upstream of the small intestine – a rumen that is heavily colonised with various micro-organisms, including cobalamin-synthesising ones, and that allows fermentation of the ingested feed. The synthesised cobalamin is later absorbed in the small intestine, incorporated into organs and muscles, and secreted into milk(Reference Jiang, Lin, Xie, Jin, Zhu and Wang49,Reference Osman, Cooke and Young52,Reference Degnan, Taga and Goodman60,Reference Rowley and Kendall73,Reference Watanabe, Bito and Koseki79–Reference Sobczyńska-Malefora, Delvin and McCaddon81) . Monogastric, non-ruminant herbivores, e.g. rabbits, receive the benefit of microbial cobalamin production in their own large intestine by consuming their faeces. This coprophagy enables microbial cobalamin absorption in the upper part of the digestive tract(Reference Osman, Cooke and Young52,Reference Degnan, Taga and Goodman60,Reference Rowley and Kendall73,Reference Antony82) . Omnivores, such as pigs and poultry, as well as carnivores acquire cobalamin from feed of animal origin(Reference Watanabe and Bito80,Reference Sobczyńska-Malefora, Delvin and McCaddon81) . In aquatic environments, cobalamin is produced only by certain bacteria and archaea. It is taken up by cobalamin-requiring bacteria as well as eukaryotic plankton, transferred to fish bodies via plankton, and concentrated in the larger piscivorous fishes. Similarly, the vitamin concentrates in the bodies of shellfish feeding on plankton(Reference Watanabe and Bito80,Reference Bito, Tanioka and Watanabe83–Reference Watanabe, Yabuta, Tanioka and Bito85) .
Accordingly, foods of animal origin are the principal sources of vitamin B12 for humans(63,Reference Watanabe and Bito80,Reference González-Montaña, Escalera-Valente and Alonso86,Reference Franco-Lopez, Duplessis and Bui87) . The most important ones include meat, milk and dairy products, fish, shellfish and eggs(Reference Basu and Donaldson64,Reference Gille and Schmid68,Reference Bito, Tanioka and Watanabe83,Reference Watanabe84,88–Reference Planells, Sanchez and Montellano136) . The highest levels of cobalamin are found in offal, especially the liver and kidney(Reference Basu and Donaldson64,Reference Gille and Schmid68,Reference Janice Marie Sych and Stevens70,Reference Allen, Miller and de Groot93,Reference Li111,Reference Truswell137–Reference Azzini, Raguzzini and Polito141) . Shellfish, such as mussels, oysters, clams and shrimps, are rich in cobalamin. However, certain types of edible shellfish (herbivorous sea snails such as abalone and turban shell) are not suitable as a source of vitamin B12 because they contain substantial amounts of pseudocobalamin(Reference Watanabe and Bito80,Reference Watanabe, Yabuta, Tanioka and Bito85,Reference Watanabe and Bito142–Reference Tanioka, Takenaka and Furusho147) . Pseudocobalamin (or pseudovitamin B12) is an analogue of cobalamin, in which adenine substitutes for 5,6-dimethylbenzimidazole as the lower axial ligand. It is biologically very likely inactive in humans because the intrinsic factor is very specific for binding cobalamin. Prevention of the absorption of cobalamin analogues might protect humans from their potential deleterious effects(Reference Smith, Warren and Refsum6,Reference Allen and Stabler74,Reference Watanabe, Bito and Koseki79,Reference Watanabe and Bito142,Reference Stupperich and NEXØ148–Reference Stabler, Bernadette, Marriott and Yates152) . Interestingly, edible insects, such as mealworms, grasshoppers and cockroaches, might also be a source of vitamin B12 for humans. Crickets, however, do not suit this purpose because they contain pseudocobalamin as the predominant corrinoid compound(Reference Schmidt, Call, Macheiner and Mayer153–Reference Bawa, Songsermpong, Kaewtapee and Chanput156).
Fungi, as mentioned above, neither produce nor utilise vitamin B12. Therefore, mushrooms and yeasts, e.g. black morels (Morchella conica), oyster mushrooms (Pleurotus ostreatus), parasol mushrooms (Macrolepiota procera), porcini mushrooms (Boletus sp.) and yeasts (Yarrowia lipolytica), generally contain none or very low amounts of this vitamin, which is presumably derived from the substrate on which they grow or from cobalamin-synthesising bacteria that live on the mushroom surface. Among edible mushrooms, the highest vitamin B12 contents were found in truffles (Tuber sp.), black trumpet (Craterellus cornucopioides), golden chanterelle (Cantharellus cibarius) and shiitake (Lentinula edodes)(Reference Bito, Teng and Ohishi14,Reference Watanabe and Bito80,Reference Watanabe, Yabuta, Tanioka and Bito85,Reference Watanabe, Schwarz and Takenaka157–Reference Jach and Malm167) .
Algae, like other eukaryotic organisms, are not capable of synthesising vitamin B12 de novo. Over one half of all algal species require vitamin B12. Those algae, as well as cobalamin-independent ones, which can, however, also accumulate exogenous cobalamin, acquire the vitamin from prokaryotic producers that are in symbiotic relationships with algae or reside on algal surfaces(Reference Croft, Lawrence and Raux-Deery11,Reference Tang, Koch and Gobler12,Reference Ramanan, Kim and Cho53,Reference Kazamia, Czesnick and Nguyen56,Reference Watanabe and Bito80,Reference Bito, Tanioka and Watanabe83,Reference de Brito, Campos and Menezes158,Reference Brawley, Blouin and Ficko-Blean168–Reference Kazamia, Helliwell, Purton and Smith179) . Changes in the character and magnitude of the epiphytic prokaryotic communities related to the region or algal physiological state (e.g. growing conditions and harvesting period) may contribute to variation in the vitamin content, but these factors are currently poorly quantified(Reference Wells, Potin and Craigie180). Contents of vitamin B12 vary interspecifically and intraspecifically in edible algae ranging from traces, e.g. in Irish moss (Chondrus crispus) and hijiki (Sargassum fusiforme), to substantial amounts, e.g. in green laver (Ulva spp.), purple laver or nori (Porphyra/Pyropia spp.) and Chlorella spp., especially the Chlorella grown non-aseptically under open culture conditions(Reference Watanabe and Bito80,Reference Bito, Tanioka and Watanabe83,Reference Kwak, Park and Cho144,Reference Bito, Teng and Watanabe150,Reference de Brito, Campos and Menezes158,Reference Bito, Bito and Asai172,Reference Kanazawa176,Reference Watanabe, Takenaka and Katsura181–Reference Cherry, O’Hara and Magee193) .
Plants, like fungi, neither synthesise nor use vitamin B12 in their metabolism(Reference Roth, Lawrence and Bobik18,Reference Smith, Croft, Moulin and Webb20) . A few exceptions of plants containing some cobalamin have been reported: edible duckweed Wolffia globosa called mankai(Reference Kaplan, Zelicha and Tsaban194,Reference Sela, Yaskolka Meir and Brandis195) , sea buckthorn (Hippophae rhamnoides), elecampane (Inula helenium), couch grass (Elymus repens)(Reference Nakos, Pepelanova and Beutel21,Reference Kysil61) and tea plant (Camellia sinensis)(Reference Kittaka-Katsura, Watanabe and Nakano196). Cobalamin in those plants is, like in other eukaryotes, of prokaryotic origin and is presumably produced by symbiotic endophytic microbes(Reference Nakos, Pepelanova and Beutel21,Reference Kaplan, Zelicha and Tsaban194,Reference Sela, Yaskolka Meir and Brandis195) or taken up from soil containing some organic fertilisers such as fishmeal or manure(Reference Antony82,Reference Kittaka-Katsura, Watanabe and Nakano196,Reference Lawrence, Nemoto-Smith and Deery197) . Therefore, cobalamin is not a normal constituent of commonly eaten plant foods unless they are contaminated with cobalamin-producing microbes (e.g. from soil or manure), contain yeasts or have been exposed to microbial fermentation that have produced the vitamin, or have been fortified with cobalamin (e.g. fortified ready-to-eat breakfast cereals and bread)(Reference Smith, Warren and Refsum6,Reference Nakos, Pepelanova and Beutel21,Reference Sobczyńska-Malefora, Delvin and McCaddon81,Reference Antony82,Reference Watanabe84,88–Reference Tucker, Rich and Rosenberg90,Reference Lavriša, Hristov and Hribar104,Reference Truswell137,Reference Stabler, Bernadette, Marriott and Yates152,Reference Ofoedu, Iwouno and Ofoedu198–Reference Yajnik, Deshpande and Lubree202) .
Thus, individuals who consume diets completely free of animal products (vegans) and even lacto-ovo vegetarians are at risk of vitamin B12 deficiency compared with omnivores. Indeed, different national nutritional societies quote a need to ensure a reliable source of vitamin B12 in persons on plant-based diets(63,Reference Allen66,Reference Sobczyńska-Malefora, Delvin and McCaddon81,Reference Ströhle, Richter and González-Gross102,Reference Lavriša, Hristov and Hribar104,Reference Obeid, Heil and Verhoeven108,Reference Rizzo, Laganà and Rapisarda163,Reference Allen203–Reference Niklewicz, Smith and Smith244) . Some dietary ingredients or food supplements of non-animal origin may be useful for vegetarians to partly contribute to the supply of vitamin B12. Microalgae Chlorella and Spirulina (Arthrospira) are the commercially most produced microalgal genera as dietary supplements (Reference Pulz and Gross245,Reference Lafarga, Fernández-Sevilla, González-López and Acién-Fernández246) . In particular, the green alga Chlorella containing cobalamin is a relevant source of it. On the contrary, microalgae, e.g. Spirulina, Aphanizomenon and Nostoc, i.e. cyanobacteria, contain predominantly pseudocobalamin that they synthesise, and only minor amounts of cobalamin acquired from the environment; therefore, they are not suitable sources of vitamin B12. It should also be emphasised that nutrient labels on products often do not differentiate between forms of vitamin B12 – i.e. they do not specify that pseudocobalamin is present there instead of cobalamin(Reference Helliwell, Lawrence and Holzer13,Reference Kennedy and Taga23,Reference Heal, Qin and Ribalet24,Reference Watanabe84,Reference Watanabe, Yabuta, Tanioka and Bito85,Reference Ströhle, Richter and González-Gross102,Reference Watanabe and Bito142,Reference Rizzo, Laganà and Rapisarda163,Reference van den Oever and Mayer171,Reference Bito, Bito and Asai172,Reference Wells, Potin and Craigie180,Reference Watanabe, Takenaka and Kittaka-Katsura182,Reference Watanabe, Yabuta, Bito and Teng184,Reference Kittaka-Katsura, Fujita, Watanabe and Nakano189,Reference Edelmann, Aalto and Chamlagain191,Reference Merchant, Phillips and Udani192,Reference Watanabe, Katsura and Takenaka247–Reference Castillejo, Martínez-Hernández and Goffi253) . Macroalgae (seaweeds) green laver and purple laver, the most widely consumed edible algae, belong to the best non-animal sources of cobalamin; consumption of approximately 4 g of dried purple laver could supply the US recommended dietary allowance of 2.4 μg/d(Reference Watanabe and Bito80,Reference Watanabe, Yabuta, Tanioka and Bito85,Reference Kwak, Lee and Lee115,Reference Bito, Teng and Watanabe150,Reference de Brito, Campos and Menezes158,Reference Watanabe, Takenaka and Katsura181,Reference Watanabe, Takenaka and Kittaka-Katsura182,Reference Watanabe, Yabuta, Bito and Teng184,Reference Bito and Watanabe185,Reference Miyamoto, Yabuta and Kwak187,Reference Martínez–Hernández, Castillejo and Carrión–Monteagudo188,Reference Cherry, O’Hara and Magee193,Reference Cornish, Critchley and Mouritsen254–Reference Green, Allen and Bjørke-Monsen260) . Shiitake can serve as a source of cobalamin. Nevertheless, although about 50 g of dried fruiting bodies could be adequate to achieve the daily cobalamin requirement, ingestion of such large amounts would not be feasible daily(Reference Bito, Teng and Ohishi14,Reference Watanabe and Bito80,Reference de Brito, Campos and Menezes158,Reference Marczykowski and Breidenassel259) . Sea buckthorn berries as well as mankai contain acceptable quantities of cobalamin; approximately 18 g of sea buckthorn jam (about 1.5 tablespoons), 6.5 g of sea buckthorn dried berries or circa 100 g of dried mankai (equivalent to 500 g of frozen mankai for making five cups of green shakes) may cover the daily US recommended amount of the vitamin specified above(Reference Nakos, Pepelanova and Beutel21,Reference Sela, Yaskolka Meir and Brandis195,Reference Jedut, Szwajgier, Glibowski and Iłowiecka261) . However, the cobalamin contents in all these alternative sources fluctuate greatly(Reference Bito, Teng and Ohishi14,Reference Nakos, Pepelanova and Beutel21,Reference Kysil61,Reference Watanabe and Bito80,Reference de Brito, Campos and Menezes158,Reference Teng, Bito and Takenaka160,Reference Rizzo, Laganà and Rapisarda163,Reference Bito, Bito and Asai172,Reference Kittaka-Katsura, Fujita, Watanabe and Nakano189) . Moreover, safety hazards posed by some constituents of those dietary products should not be underestimated. Continuous intake of large amounts of those products might adversely affect human health owing to the presence of potentially harmful substances, whose content should be monitored during the quality control(Reference Sánchez-Parra, Boutarfa and Aboal262–Reference Van Hassel, Ahn and Huybrechts267). High consumption of algae is associated with higher risks of deleterious effects due to excessive intake of some minerals that algae naturally accumulate, e.g. iodine, cancerogenic arsenic and toxic heavy metals(Reference Bito, Teng and Watanabe150,Reference Wells, Potin and Craigie180,Reference Cherry, O’Hara and Magee193,Reference Rogerson218,Reference Kristensen, Madsen and Hansen228,Reference Sandgruber, Gielsdorf and Baur249,Reference Sánchez-Parra, Boutarfa and Aboal262,Reference Rzymski, Niedzielski and Kaczmarek263,Reference Grosshagauer, Kraemer and Somoza265,Reference Muñoz, Díaz and Nelson268–Reference Lähteenmäki-Uutela, Rahikainen and Camarena-Gómez284) . In addition, these products might be detrimental to human health because they may be contaminated with toxic cyanotoxins produced by some cyanobacteria(Reference Sánchez-Parra, Boutarfa and Aboal262,Reference Rzymski and Jaśkiewicz264–Reference Van Hassel, Ahn and Huybrechts267,Reference Mendes, Navalho and Ferreira270,Reference Marles, Barrett and Barnes285) and with cancerogenic polycyclic aromatic hydrocarbons from the environment(Reference Grosshagauer, Kraemer and Somoza265,Reference Ampofo and Abbey266,Reference Muys, Sui and Schwaiger280) . Similarly to algae, excessive ingestion of mushrooms may threaten human health as a consequence of exceeding dietary exposure limits of heavy metals, arsenic and radionuclides(Reference Li, Wang and Wang286–Reference Guillén and Baeza299). Consumption of Wolffia globosa (mankai) is of safety concern because it leads to an increase in manganese intake which could represent a risk of adverse health effects(300). Fermented plant-based foods, such as kimchi, sauerkraut, injera, kombucha, tempeh and miso, are generally poor dietary sources of vitamin B12 for vegetarians mainly because there is simply not enough vitamin B12 produced by these fermentations(Reference Nakos, Pepelanova and Beutel21,Reference Kennedy and Taga23,Reference Herbert65,Reference Watanabe, Yabuta, Tanioka and Bito85,Reference Kwak, Lee, Oh and Park114,Reference Kwak, Lee and Lee115,Reference de Brito, Campos and Menezes158,Reference Rizzo, Laganà and Rapisarda163,Reference Watanabe, Yabuta, Bito and Teng184,Reference Melina, Craig and Levin205,Reference Stabler and Allen214,Reference Kwak, Hwang, Watanabe and Park255,Reference Jedut, Szwajgier, Glibowski and Iłowiecka261,Reference Liem, Steinkraus and Cronk301–Reference Masuda, Ide and Utsumi303) . Taking everything into account, all these alternative sources of cobalamin for vegetarians are unreliable, insufficient or impractical to meet cobalamin needs of the human body in the long term. They may improve vitamin B12 status in vegetarians but cannot replenish the total body store of the vitamin(Reference Watanabe, Yabuta, Tanioka and Bito85,Reference de Brito, Campos and Menezes158,Reference Watanabe, Yabuta, Bito and Teng184,Reference Merchant, Phillips and Udani192–Reference Sela, Yaskolka Meir and Brandis195,Reference Melina, Craig and Levin205,Reference Zugravu, Macri, Belc and Bohiltea304–309) .
Plant-based diets, except those rich in ultra-processed plant-based food products(Reference Gehring, Touvier and Baudry310–Reference Orlich, Sabaté and Mashchak319), are considered potentially superior to a traditional omnivorous diet for reducing the risk of chronic diseases, such as metabolic syndrome with type 2 diabetes mellitus, hypertension, cardiovascular diseases in general, and several types of cancer(Reference Melina, Craig and Levin205,Reference Shaw, Zello and Rodgers217,Reference Phillips222,Reference Bakaloudi, Halloran and Rippin227,Reference Dawczynski, Weidauer and Richert241,Reference Craig, Mangels and Fresán320–Reference Nhan, Sgambat and Moudgil344) . Meanwhile, compared with omnivorous diets, plant-based ones are deficient in some nutrients, which could have detrimental health implications as well. Vitamin B12 is of particular relevance(Reference Craig204,Reference Phillips222,Reference Bakaloudi, Halloran and Rippin227,Reference Schüpbach, Wegmüller and Berguerand231,Reference Dawczynski, Weidauer and Richert241–Reference Niklewicz, Smith and Smith244,Reference Brown305,Reference Nebl, Schuchardt and Ströhle345,Reference Weikert, Trefflich and Menzel346) . The proposed benefit of plant-based-diets is conditioned by the balance and incorporation of all missing constituents so that nutritional quality is not compromised. Regular intake of cobalamin-fortified foods (mostly ready-to-eat-cereals, bread, nutritional yeast, meat analogues, such as tofu, and milk substitutes, e.g. soy, almond and rice milk) and/or cobalamin-containing supplements is recommended for people on plant-based diets to prevent vitamin B12 deficiency(Reference Janice Marie Sych and Stevens70,Reference Antony82,Reference Watanabe, Yabuta, Tanioka and Bito85,Reference Allen, Miller and de Groot93,Reference Azzini, Raguzzini and Polito141,Reference Rizzo, Laganà and Rapisarda163,Reference Watanabe, Yabuta, Bito and Teng184,Reference Melina, Craig and Levin205,Reference Rogerson218–Reference Phillips222,Reference Zeuschner, Hokin and Marsh224–Reference Woo, Kwok and Celermajer233,Reference White235,Reference Pawlak, Lester and Babatunde236,Reference Seves, Verkaik-Kloosterman, Biesbroek and Temme238,Reference Dawczynski, Weidauer and Richert241–Reference Neufingerl and Eilander243,Reference Chandra-Hioe, Lee and Arcot256,Reference Marczykowski and Breidenassel259,Reference Brown305–Reference Strain, Hughes, Pentieva, Biesalski, Drewnowski, Dwyer, Strain, Weber and Eggersdorfer308,Reference Craig, Mangels and Fresán320–Reference Mangels, Messina and Melina326,Reference Nebl, Schuchardt and Ströhle345–Reference Storz, Müller and Niederreiter373) . The vitamin B12 status should also be monitored regularly(Reference Ströhle, Richter and González-Gross102,Reference Azzini, Raguzzini and Polito141,Reference Rogerson218,Reference Herrmann, Schorr, Obeid and Geisel223,Reference Zeuschner, Hokin and Marsh224,Reference Bakaloudi, Halloran and Rippin227,Reference Woo, Kwok and Celermajer233,Reference White235,Reference Agnoli, Baroni and Bertini242,Reference Chandra-Hioe, Lee and Arcot256,Reference Richter, Boeing and Grünewald-Funk306,Reference Schwarz, Dschietzig and Schwarz307,Reference Craig, Mangels and Fresán320,Reference Jakše325,Reference Mangels, Messina and Melina326,Reference Donaldson347,Reference Rudloff, Bührer and Jochum360–Reference Baroni, Goggi and Battaglino362,Reference Kiely367,Reference Hunt, Harrington and Robinson374) .
Contents of cobalamin in some selected foodstuffs are presented in Table 1.
Table 1. Cobalamin contents in selected foodstuffs
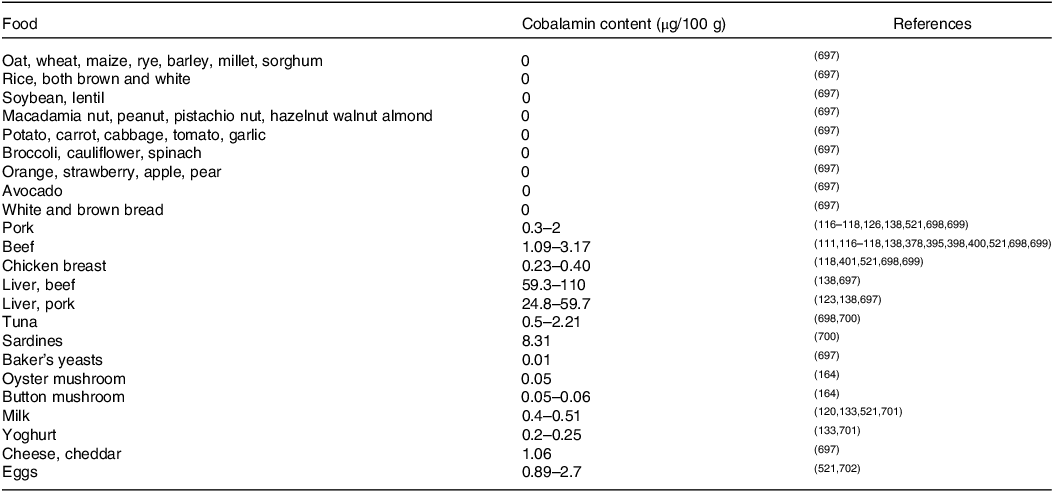
The stability of vitamin B12 in food products during processing, preparation and storage is an important parameter affecting the supply of the vitamin to consumers(Reference Janice Marie Sych and Stevens70,Reference Watanabe, Yabuta, Tanioka and Bito85,Reference Berry Ottaway, Skibsted, Risbo and Andersen375–Reference Bennink and Ono378) . Cyanocobalamin is chemically more stable than adenosylcobalamin, methylcobalamin and hydroxocobalamin. In neutral and weakly acid aqueous solutions, it is stable at room temperature with highest stability at pH 4.5–5 and it is relatively stable to the thermal processing. Loss of vitamin activity may occur due to heat treatments under alkaline and strong acid conditions or due to light or via contact with reducing agents, such as ascorbic acid, polyphenols, nitrous oxide, sulphite and iron(II) salts, but also with oxidising ones, such as atmospheric oxygen, hypochlorous acid and chloramine-T(Reference Bito, Teng and Ohishi14,Reference Nakos, Pepelanova and Beutel21,Reference Gille and Schmid68,Reference Janice Marie Sych and Stevens70,Reference Li, Gill, Grainger and Manley-Harris124,Reference Watanabe and Bito142,Reference Teng, Bito and Takenaka160,Reference Ofoedu, Iwouno and Ofoedu198,Reference Berry Ottaway, Skibsted, Risbo and Andersen375–Reference Bergström377,Reference Lee, Lee, Huh and Choi379–Reference Temova Rakuša, Roškar, Hickey and Geremia393) . On the one hand, degradation of vitamin B12 caused by ascorbic acid might have little practical importance because foods containing vitamin B12 generally do not contain significant amounts of vitamin C(Reference Godoy, Amaya-Farfan, Rodriguez-Amaya, Rodriguez-Amaya and Amaya-Farfan376), but on the other hand, it should be taken into account, e.g. in vitamin fortified beverages(Reference Bajaj and Singhal388,Reference Yamada, Shimodaira and Chida390) .
In food matrices, vitamin B12 is generally considered to be rather heat stable compared with other water-soluble vitamins. Reported losses depend on the type of food and processing conditions(Reference Gille and Schmid68,Reference Janice Marie Sych and Stevens70,Reference Bodwell and Anderson134,Reference Bennink and Ono378,Reference Campo, Muela and Olleta394–Reference Öhrvik, Carlsen, Källman and Martinsen406) . In milk, vitamin B12 is affected by heat processing; the more severe the process, the greater the loss is. Vitamin losses are generally less than 10% after pasteurisation, 10–20% after ultra-high temperature treatment, up to 20% following sterilisation and 20–35% during spray drying(Reference Watanabe84,Reference Berry Ottaway, Skibsted, Risbo and Andersen375,Reference Rolls and Porter396,Reference Oamen, Hansen and Swartzel407–Reference Graham411) . The vitamin B12 amount decreased three times more in heated chocolate milk (by about 33%) than in the unflavoured one because cocoa powder used for milk flavouring contains polyphenols, which are mainly responsible for the decomposition of the vitamin(Reference Johns, Das and Kuil383). In meat, vitamin B12 is stable during the cooking, if the vitamin content in the cooking liquids, gravy and drippings is taken into consideration(Reference Berry Ottaway, Skibsted, Risbo and Andersen375,Reference Bognár403) . Indeed, most vitamin B12 losses result from leaching into water, e.g. during cooking or freezing/thawing(Reference Nishioka, Kanosue, Yabuta and Watanabe399,Reference Bognár403,Reference Lešková, Kubíková and Kováčiková412–Reference Sato, Kudo and Muramatsu415) . This process is essentially similar to that of other water-soluble B vitamins(Reference Hrubša, Siatka, Nejmanová, Vopršalová, Kujovská Krčmová and Matoušová1). Boiling, stewing and frying lead to vitamin B12 losses of 20–40%, 10–40%, 30–50% and 10–20% in pork, beef, chicken and fish, respectively(Reference Bognár403). The highest reduction in the vitamin content occurs during boiling(Reference Bognár403–Reference Öhrvik, Carlsen, Källman and Martinsen406). The best method for cooking fish was vacuum-packed pouch cooking with no loss of vitamin B12, compared with steaming, boiling, grilling, frying and microwaving(Reference Nishioka, Kanosue, Yabuta and Watanabe399). Scrambled, fried and hard-cooked eggs lose during cooking 5%, 5–15% and 20% vitamin B12, respectively(Reference Bognár403,Reference Öhrvik, Carlsen, Källman and Martinsen406) .
Treatment of foods with penetrating waves such as microwaves was shown to promote the degradation of vitamin B12 (Reference Janice Marie Sych and Stevens70,Reference Nishioka, Kanosue, Yabuta and Watanabe399,Reference Watanabe, Abe and Fujita416–Reference Zheng, Xiang and Zhang418) . For instance, decreases by 17%, 14% and 48% were estimated in beef, pork and milk, respectively, treated with microwave heating for 6 min, which is a common time used for reheating of foods(Reference Watanabe, Abe and Fujita416).
Puffed rice extrudates could be used as a palatable vehicle for fortification with vitamin B12; losses of added vitamin ranged from 19% to 64% depending on extrusion processing parameters(Reference Bajaj and Singhal419). The stability of the added and in situ-produced vitamin B12 in breadmaking varied according to the chosen process (straight-, sponge- and sourdough processes)(Reference Edelmann, Chamlagain and Santin386,Reference Chamlagain420) . Converning baking from fortified whole wheat, the vitamin amounts were reduced by 9%, 20%, 66% and 76% in chapattis (unleavened flatbread), bread, cake and cookie, respectively. The lowest vitamin B12 retention was recorded in pooris prepared from fortified whole wheat flour by frying; the vitamin loss was 86%(Reference Bajaj and Singhal421).
Vitamin B12 is sensitive to light and ultraviolet (UV) radiation(Reference Janice Marie Sych and Stevens70,Reference Watanabe84,Reference Berry Ottaway, Skibsted, Risbo and Andersen375,Reference Godoy, Amaya-Farfan, Rodriguez-Amaya, Rodriguez-Amaya and Amaya-Farfan376,Reference Henry and Heppell381,Reference Yessaad, Bernard and Bourdeaux382,Reference Lie, Chandra-Hioe and Arcot384,Reference Juzeniene and Nizauskaite422,Reference Bito, Ohishi and Hatanaka423) . Sunlight at a brightness of 8000-foot candles (approximately 86 000 lux) caused a 10% loss of cyanocobalamin for each 30 min of exposure in neutral aqueous solutions, but exposures to levels of brightness below 300-foot candles (approximately 3200 lux) had little effect(Reference Berry Ottaway, Skibsted, Risbo and Andersen375). Photodegradation of cyanocobalamin in aqueous solutions may be accelerated by riboflavin, which acts as a sensitiser(Reference Godoy, Amaya-Farfan, Rodriguez-Amaya, Rodriguez-Amaya and Amaya-Farfan376,Reference Juzeniene and Nizauskaite422) . The vitamin B12 concentration in milk exposed to the light for 24 h decreased by 1–27%, depending on the type of milk tested(Reference Watanabe, Katsura, Abe and Nakano424), while no changes in the vitamin B12 content occurred in pasteurised milk packed in a clear polyethylene terephthalate bottle exposed to fluorescent light (1700 lux) for 10 d(Reference Saffert, Pieper and Jetten425). The photostability of vitamin B12 in foods may be increased due to matrix effects, such as binding to proteins(Reference Janice Marie Sych and Stevens70,Reference Zironi, Gazzotti and Barbarossa121,Reference Campos-Gimnez, Fontannaz and Trisconi426,Reference Wang, Shou and Zhu427) . Furthermore, light penetrates only slightly below the surface of foods, which would suggest that vitamin B12 photosensitivity is not a serious issue in most foods(Reference Janice Marie Sych and Stevens70).
Vitamin B12 content decreases in fermented milk products(Reference Scott and BISHOP397,Reference Arkbåge, Witthöft, Fondén and Jägerstad410) . Fermentation of milk resulted in vitamin B12 losses of 25% in yoghurt and 15% in Filmjölk. Storage of an unopened package of the final product at 4°C for 14 d, until the ‘use by date’, reduced the vitamin concentrations further by 33% and 26% for yoghurt and Filmjölk, respectively, so that they contained 40–60% of vitamin B12 originally present in the milk. This is most likely attributed to the consumption of the vitamin by starter cultures of lactic acid bacteria, which are metabolically active not only during fermentation but also at lower temperatures during storage(Reference Arkbåge, Witthöft, Fondén and Jägerstad410). During the cheese-making process, the whey fraction is removed, leading to a considerable loss of vitamin B12 (on average about 50% of the vitamin originally present in the milk) due to its water solubility. Meanwhile, the vitamin content in final products (especially in hard cheeses) is higher relative to the starting milk owing to the milk thickening during cheese production (for instance, about 10 litres of milk is required to produce 1 kg of hard cheese). Ripening and storage of cheeses do not alter the vitamin B12 content, except in mold cheeses, in which the content may decline(Reference Gille and Schmid68,Reference Arkbåge, Witthöft, Fondén and Jägerstad410,Reference Repossi, Zironi and Gazzotti428) . Swiss-type cheeses (e.g. Emmentaler and Gruyère) contain higher amounts of vitamin B12 than other ones owing to the application of propionibacteria as adjunct starter cultures for ripening (responsible for the characteristic flavor and opening formation) that are able to produce vitamin B12 (Reference Gille and Schmid68,Reference Matte, Britten and Girard99,Reference Arkbåge, Witthöft, Fondén and Jägerstad410,Reference Rabah, Carmo and Jan429,Reference Poonam and Tomar430) .
Maturation of meat between the time of slaughtering and consumption for up to 14 d does not affect the vitamin B12 content in beef(Reference Ortigues-Marty, Thomas and Prévéraud398).
Vitamin B12 is fairly stable to ionising radiation, which is used as a food preservation method to control foodborne pathogens and extend product shelf life; no losses of the vitamin were found in irradiated pork, chicken, clam and haddock(Reference Kilcast431–Reference Fox, Thayer and Jenkins433). Hypochlorous acid water (used to sanitise food products, e.g. vegetables, fruits and meat) as well as sodium metabisulfite and sodium sulfite (used to prevent black discoloration of shrimps) destroy vitamin B12 in aqueous solutions but do not reduce its content in shrimps. Similarly, no significant changes in vitamin B12 amounts occur in beef treated with hypochlorous acid water. This is explained by the fact that most vitamin B12 present in foods is in protein-bound form rather than free(Reference Okamoto, Bito and Hiura385).
Some food ingredients have been shown to influence positively the stability of vitamin B12. Sorbitol, a sweetener, protects cyanocobalamin from degradation by heat, ascorbic acid, thiamine, UV light, and low or high pH values (Reference Lee, Lee, Huh and Choi379,Reference Lie, Chandra-Hioe and Arcot384) . Whey proteins enhance thermal stability of vitamin B12 by 20% and could be useful as protective agents against the physical destruction of the vitamin during food processing(Reference Wang, Shou and Zhu427). Carnosine, a dipeptide naturally present in meat, prevents the destruction of cyanocobalamin by vitamin C in the presence of copper ions and may be useful as an additive to multivitamin–mineral food supplements. Carnosine has been shown to possess antioxidant and metal chelating activity, which could be responsible for the observed protection(Reference Nakos, Pepelanova and Beutel21,Reference Takenaka, Sugiyama and Watanabe434) .
Storage may influence the rate of vitamin B12 decomposition(Reference Godoy, Amaya-Farfan, Rodriguez-Amaya, Rodriguez-Amaya and Amaya-Farfan376,Reference Bajaj and Singhal388,Reference Yamada, Shimodaira and Chida390,Reference Ford, Hurrell and Finot435,Reference Hemery, Fontan and Laillou436) . For instance, storage of ultra-high-temperature milk at room temperature for 18 weeks resulted in the complete disappearance of vitamin B12, probably due to exposure to dissolved oxygen in the container, while low temperature (7°C) did not alter the vitamin content for up to 18 weeks(Reference Gille and Schmid68,Reference Arkbåge131,Reference Godoy, Amaya-Farfan, Rodriguez-Amaya, Rodriguez-Amaya and Amaya-Farfan376,Reference Rolls and Porter396,Reference Oamen, Hansen and Swartzel407) . No appreciable losses of vitamin B12 were found in pasteurised milk during storage in a refrigerator for 9 d, regardless of how long it had been since the packages had been opened(Reference Andersson and Öste409). Effects of storage conditions (time, temperature, moisture content, oxygen and nitrogen) on the vitamin B12 amount in milk powders have also been studied(Reference Ford, Hurrell and Finot435). No remarkable changes in the vitamin B12 content occurred in vacuum-packaged salmon stored for 880 d at room temperature either on the Earth or exposed to spaceflight(Reference Zwart, Kloeris and Perchonok437). Cyanocobalamin loss reached up to 63% in fortified wheat flour packed in permeable paper bags, whereas no significant reduction of vitamin amounts occurred, when the flour was packed in multilayer aluminium/polyethylene bags (non-permeable to oxygen and humidity)(Reference Hemery, Fontan and Laillou436). When whole wheat flour fortified with cyanocobalamin was stored in air-tight plastic containers in the dark under different combinations of temperature (25°C and 45°C) and relative humidity (33%, 63% and 93%) to mimic the effects of various climatic conditions, the highest and lowest vitamin losses of 51% and 15% were recorded at 45°C/93% and at 25°C/all, respectively, after 120 d of storage, suggesting that wheat flour may be effectively fortified with vitamin B12 (Reference Bajaj and Singhal421). The degradation kinetics of vitamin B12 in fortified co-crystallised sugar cubes was studied under different storage conditions as for temperature and humidity; a half-life of 23 months was achieved at 25°C and 33% relative humidity(Reference Bajaj and Singhal438). Vitamin B12 was stable in a salt fortified with multiple micronutrients, including microencapsulated vitamins, during 6 months of storage(Reference Vinodkumar and Rajagopalan439). Different storage temperatures of fortified juices from carrot (pH 6) and lime (pH 2, richer in vitamin C promoting vitamin B12 degradation) showed losses of vitamin B12 of 8%, 15% and 19% in carrot juice and 82%, 95% and 100% in lime juice, respectively after 28 d at 4°C, 25°C and 37°C. Carrot juice is therefore more suitable for vitamin B12 fortification owing to its mild acidic character(Reference Bajaj and Singhal388).
Production of vitamin B12 and biofortification with B12
Vitamin B12, mainly in the most stable form cyanocobalamin, is commercially produced for use in fortified foods, dietary supplements, pharmaceuticals and animal feeds(Reference Hohmann, Litta and Hans67,Reference Sobczyńska-Malefora, Delvin and McCaddon81,88,Reference Allen, Miller and de Groot93,Reference Ströhle, Richter and González-Gross102,Reference Azzini, Raguzzini and Polito141,Reference Stabler, Bernadette, Marriott and Yates152,Reference Tucker, Olson and Bakun199,Reference Stabler and Allen214,Reference Chandra-Hioe, Lee and Arcot256,Reference Green, Allen and Bjørke-Monsen260,Reference Zugravu, Macri, Belc and Bohiltea304,Reference Damayanti, Jaceldo-Siegl and Beeson348,Reference Del Bo, Riso and Gardana351,Reference Lee, Lee, Huh and Choi379,Reference Obeid, Fedosov and Nexo440–Reference de Benoist465) . The total synthesis of vitamin B12 comprising about seventy reactions was achieved in 1972(Reference Woodward466–Reference Riether and Mulzer470). Due to the vitamin’s enormous structural complexity, its chemical synthesis is highly complicated and not economically feasible on an industrial scale. Therefore, industrial production of vitamin B12 is exclusively based on microbial fermentation. Currently, the most commonly employed micro-organisms are high-producing bacterial strains of Pseudomonas denitrificans and Propionibacterium freudenreichii, developed by means of random mutagenesis and selection, as well as genetic engineering from wild strains with high natural production ability(Reference Smith, Warren and Refsum6,Reference Martens, Barg, Warren and Jahn7,Reference Balabanova, Averianova, Marchenok, Son and Tekutyeva48,Reference Osman, Cooke and Young52,Reference Hohmann, Litta and Hans67,Reference Janice Marie Sych and Stevens70,Reference Pereira, Simões and Silva175,Reference Calvillo, Pellicer, Carnicer and Planas450,Reference Acevedo-Rocha, Gronenberg and Mack471–Reference Kumar, Singh and Tiwari502) . Fermentations produce a mixture of hydroxocobalamin, adenosylcobalamin and methylcobalamin; they are then converted to cyanocobalamin by the addition of potassium cyanide(Reference Martens, Barg, Warren and Jahn7,Reference Balabanova, Averianova, Marchenok, Son and Tekutyeva48,Reference Janice Marie Sych and Stevens70,Reference Calvillo, Pellicer, Carnicer and Planas450,Reference Acevedo-Rocha, Gronenberg and Mack471,Reference Shimizu474,Reference Laudert, Hohmann and Moo-Young476,Reference Binod, Sindhu and Pandey478,Reference Survase, Bajaj and Singhal479) . Current industrial biotechnological processes for the production of vitamin B12 are suboptimal. Accordingly, further possible micro-organisms suitable for large-scale production have been widely studied in recent years, e.g. natural producers Bacillus megaterium and Sinorhizobium meliloti, and even Escherichia coli, which is not able to synthesise vitamin B12 de novo in nature but can do so after genetic modifications (heterologous expression of the whole biosynthetic pathway). However, the reported yields are not yet competitive with those achieved in present-day manufacturing bioprocesses(Reference Martens, Barg, Warren and Jahn7,Reference Balabanova, Averianova, Marchenok, Son and Tekutyeva48,Reference Calvillo, Pellicer, Carnicer and Planas450,Reference Acevedo-Rocha, Gronenberg and Mack471,Reference Wang, Liu, Jin and Zhang472,Reference Mani503–Reference Xu, Xiao and Yu517) .
Biofortification aims to make crop plants naturally more nutritive rather than adding nutrient supplements to the foods during food processing. Biofortification can be achieved through three main approaches including plant breeding, transgenic techniques and agronomic practices(Reference Malik and Maqbool518–Reference Oh, Cave and Lu522). No efforts are being made to biofortify crops with vitamin B12 through conventional breeding or genetic engineering due to the fact that the biosynthetic pathway is present exclusively in some bacteria and archaea(Reference Titcomb and Tanumihardjo521,Reference Garg, Sharma and Vats523) . As for the agronomic approach, which requires physical application of cobalamin to plants for enriching them with this vitamin, some biofortification attempts have been reported, e.g. in wheat and spinach(Reference Mozafar524) and green tea(Reference Kittaka-Katsura, Watanabe and Nakano196) by the addition of organic fertilisers (naturally rich in vitamin B12) to the soil, in garden cress seedlings by growing on an agar medium containing cobalamin(Reference Lawrence, Nemoto-Smith and Deery197), in Japanese radish sprout (kaiware daikon) by soaking its seeds in cobalamin solution(Reference Sato, Kudo and Muramatsu415), in lettuce(Reference Bito, Ohishi and Hatanaka423) and spinach(Reference Zheng, Xiang and Zhang418) in hydroponic culture by treatment with cobalamin, and in the recombinant alga Chlamydomonas (expressing human intrinsic factor) by culturing in a medium supplemented with cobalamin(Reference Lima, Webb and Deery525). Milk concentrations of vitamin B12 are influenced by the genotype of the cow. Genomic regions associated with vitamin B12 concentrations in milk have been identified, which offer an interesting potential for marker-assisted genetic selection and breeding to increase the content of vitamin B12 in cow milk. However, feeding composition management could help optimise vitamin B12 amounts in milk only to a limited extent as there are adequate cobalt levels in the cow’s diet required by ruminal microbiota for cobalamin biosynthesis(Reference González-Montaña, Escalera-Valente and Alonso86,Reference Duplessis, Pellerin, Cue and Girard97,Reference Matte, Britten and Girard99,Reference Rutten, Bouwman and Sprong526–Reference Stemme, Lebzien, Flachowsky and Scholz531) . The introduction of vitamin B12 into foods may also be achieved via the in situ production by micro-organisms naturally capable of synthesising cobalamin. The propionibacterium Propionibacterium freudenreichii has been most often investigated (rarely other bacteria, e.g. Bacillus megaterium (Reference Kysil61) and Acetobacter pasteurianus (Reference Bernhardt, Zhu and Schütz532)) for fortification by fermentation during food processing; and various substrates have been fermented, including cereals (e.g. wheat, barley, rye, oat, rice, sorghum and millet), pseudocereals (e.g. buckwheat, quinoa and amaranth), legumes (e.g. faba bean, soy bean and lupin beans), whey, soy milk, sunflower seed milk, cabbage, ground elder and black tea(Reference Kysil61,Reference Edelmann, Chamlagain and Santin386,Reference Chamlagain420,Reference Calvillo, Pellicer, Carnicer and Planas450,Reference Chamlagain, Deptula and Edelmann533–Reference Coelho, de Almeida and do Amaral550) . The usefulness of lactic acid bacteria, such as Lactobacillus plantarum, Lactobacillus reuteri and Lactobacillus rhamnosus, for in situ fortification with vitamin B12 is questionable. It is not clear which, if any, of these strains synthesises true cobalamin and not merely pseudocobalamin. The ability of lactic acid bacteria to produce vitamin B12 is, in fact, usually evidenced by a microbiological assay that does not distinguish between cobalamin and pseudocobalamin and is not verified by a reliable analytical method for structure elucidation and/or by a genetic analysis confirming the presence of the whole biosynthetic pathway in the genome of a particular micro-organism(Reference Watanabe, Bito and Koseki79,Reference Masuda, Ide and Utsumi303,Reference Vincenti, Bertuzzo and Limitone372,Reference Deptula, Chamlagain and Edelmann536,Reference Xie, Coda and Chamlagain539,Reference Hugenschmidt, Schwenninger, Gnehm and Lacroix545,Reference Capozzi, Russo and Duenas547,Reference Gu, Zhang and Song551–Reference De Angelis, Bottacini and Fosso563) . The practical importance of vitamin B12 biofortification strategies, including in situ methods, is so far low, if any, compared with fortification (i.e. external addition of cobalamin to foods). However, it is a promising way to provide the vitamin to consumers and requires further research.
Pharmacokinetics and homoeostasis
Absorption
In humans, the selective absorption of vitamin B12 is a multi-step process (Figure 2). The bioavailability depends on the individual’s gastrointestinal absorption capacity and, in terms of food sources, on the amount and type of protein consumed. Indeed, vitamin B12 ingested through food appears to have varying rates of absorption(Reference O’Leary and Samman5,564) . In general, the bioavailability of the vitamin from the usual diet is assumed to be about 50% (depending on the dietary source, the amount of cobalamin ingested, the ability to release cobalamin from food and the proper functioning of the intrinsic factor system), but lower from sources containing high amounts, e.g. from liver, due to saturation of the active absorption process(63,Reference Allen66,Reference Sobczyńska-Malefora, Delvin and McCaddon81,Reference Watanabe84,88,Reference Allen92,Reference Allen, Miller and de Groot93,Reference Doets, Szczecińska and Dhonukshe-Rutten98,Reference Stabler, Bernadette, Marriott and Yates152,Reference Yates565) .
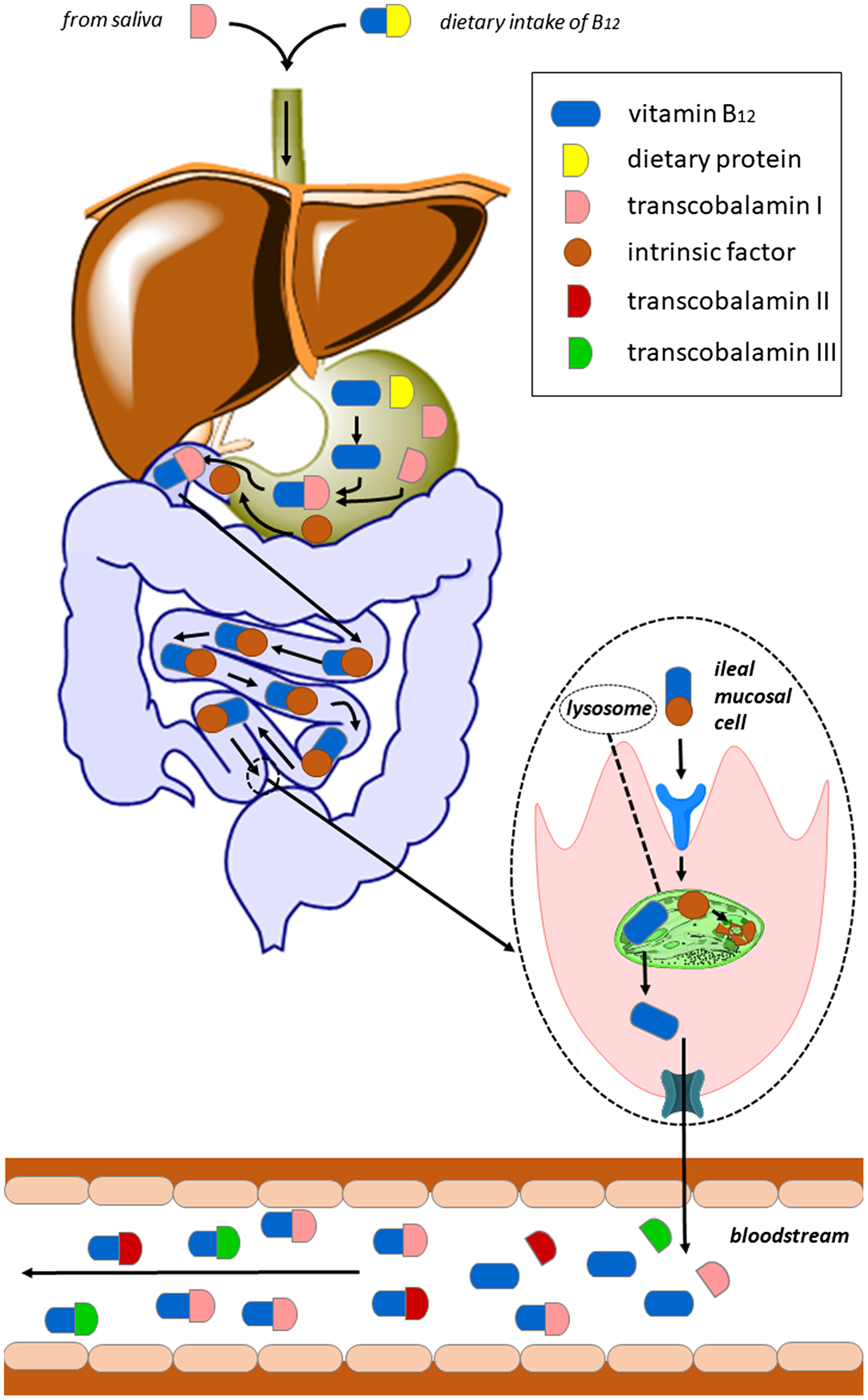
Fig. 2. Absorption of vitamin B12 via the IF pathway: Dietary protein-bound vitamin B12 can bind to transcobalamin I (TCI) only after its release mediated by pepsin and hydrochloric acid produced by the gastric mucosa. In the duodenum, TCI is degraded by pancreatic proteases and free cobalamin binds to intrinsic factor (IF). The IF–cobalamin complex is absorbed in the distal ileum by receptor-mediated endocytosis enabled by cubilin with participation of other protein(s), e.g. amnionless (AMN). IF is degraded in the lysosome and released cobalamin enters the cytoplasm likely by use of the transmembrane protein LMBD1. The precise mechanism of vitamin B12 efflux from enterocytes into the circulation is not yet well described. It appears to be mediated by several exporters; one of them is multidrug resistance protein 1 (MRP1, shown in teal colour).
Vitamin B12 is bound to proteins in food and is available for absorption only after releasing by pepsin and hydrochloric acid produced by the gastric mucosa. Subsequently, it binds to transcobalamin I (TCI) belonging to haptocorrins (HC)/R binders. Due to this classification, it is sometimes simply referred as haptocorrin. It is a glycoprotein that is found in saliva and gastric fluids and, inter alia, in blood serum. TCI has a high affinity for both B12 and for its analogues. In the duodenum, TCI is degraded by pancreatic proteases and free cobalamin binds to intrinsic factor (IF), a glycoprotein that is secreted by gastric parietal cells after a meal. There is high homology between IF and TCI, and both of them bind one molecule of vitamin B12 (Reference Kozyraki and Cases566). Cobalamin binds to IF with a higher affinity in a more alkaline environment; hence, in the stomach, where the pH is acidic, IF has a very low affinity for vitamin. This glycoprotein is much more specific for B12 binding than TCI and has limited affinity for cobalamin analogues. The IF–cobalamin complex is absorbed in the distal ileum by receptor-mediated endocytosis enabled by cubilin with participation of other protein(s), e.g. amnionless (AMN). IF is degraded in the lysosome, and released B12 enters the cytoplasm likely by use of the transmembrane protein LMBD1. The precise mechanism of vitamin B12 efflux from enterocytes into the circulation is not yet well described. It appears to be mediated by several exporters; one of them is multidrug resistance protein 1 (MRP1)(Reference O’Leary and Samman5,Reference Green, Allen and Bjørke-Monsen260,Reference Kozyraki and Cases566) .
The normal mechanism of absorption of orally administered vitamin B12, via the IF pathway, is readily saturated. While approximately 70% of vitamin B12 is absorbed from doses of 0.1–0.5 μg, it decreases to 56% at 1 μg, to 16% at 10 μg and to 3% for doses 25–50 μg(Reference Smith, Warren and Refsum6). High oral doses (100–100 000 μg) are absorbed passively, but the extent reaches only about 1% of the ingested dose(Reference Berlin, Berlin and Brante567).
Transport
In the bloodstream, the majority of B12 (80%) and all cobalamin analogues are bound to TCI, which, thanks to its relatively long biological half-life of 10 d, forms a circulating supply of vitamins in the body(Reference Fedosov and Stanger568). Of the total B12, 20–30% is carried by transcobalamin II (TCII), a non-glycosylated protein(Reference Smith, Warren and Refsum6). TCII binds physiological forms of vitamin B12, while TCI also binds B12 analogues. TCII and TCI deliver vitamin B12 to peripheral tissues and liver, respectively(Reference Shipton and Thachil569). The TCII–cobalamin complex binds in the presence of calcium to its receptor, a transmembrane, highly glycosylated protein CD320 (8D6A) containing two low-density lipoprotein-receptor class A domains. The receptor is selective to cobalamin–TCII, and neither TCI nor IF binds to it(Reference Quadros, Nakayama and Sequeira570). After endocytosis, the complex enters the lysosome, where TCII is degraded and free B12 is exported to the cytosol by use of the ATP-binding cassette transporter ABCD4. Presence of another membrane lysosomal protein LMBD1 is also necessary (Reference Smith, Warren and Refsum6,Reference Watkins and Rosenblatt571) . A third specific vitamin B12 transport protein found in human serum, transcobalamin III (TCIII), is also reported in the literature(Reference Bloomfield and Scott572–Reference Wickramasinghe, England, Saunders and Down576). Like TCI, TCIII is a glycoprotein and, in addition, these two transcobalamins are immunologically identical (Reference Burger, Schneider, Mehlman and Allen573,Reference Fràter-Schröder, Hitzig and Bütler574) .
Reabsorption and excretion
Vitamin B12 is secreted into the bile, and a part is reabsorbed by the enterohepatic circulation through ileal receptors that require IF(Reference Kozyraki and Cases566,Reference Herrmann and Obeid577) . Cobalamin is excreted in the faeces, which consists of unabsorbed biliary vitamin B12, vitamin B12 from gastrointestinal cells and secretions, and that synthesised by bacteria in the colon. When the vitamin B12 is found in the excess in the circulation, it outreaches the binding capacity of TCII, and it is also excreted in the urine(Reference O’Leary and Samman5). However, it is partially reabsorbed in the kidney by the transcobalamin II receptor megalin (Lrp2)(Reference Kozyraki and Cases566). Further losses of vitamin B12 occur through the skin and metabolic reactions(564).
Storage of cobalamin in the human body
Cobalamin, unlike other water-soluble vitamins, is stored in the human body. Most adults have stores of up to 5 mg. The liver is the main reservoir of this vitamin, and it stores normally up to one half of the total amount of the vitamin(Reference Herrmann and Obeid577,Reference Manzanares and Hardy578) . Smaller amounts of accumulated cobalamin can also be found in the kidneys and brain, and the circulating supply of TCI-bound vitamin B12 in plasma cannot be neglected(Reference Herrmann and Obeid577).
Physiological function
Vitamin B12 is essential for human metabolism, production and regeneration of carbohydrates, fats and proteins, as well as for the proper development of erythrocytes and the central nervous system. Only two forms are biologically active: methylcobalamin and adenosylcobalamin(564,Reference Kozyraki and Cases566) . The former is a cofactor for methionine synthase and the latter for l-methylmalonyl-CoA mutase (Figure 3). These B12-mediated reactions are facilitated by the ability of the cobalt ion to change its oxidation states, Co (I), Co (II) and Co (III) (Figures 4 and 5)(Reference Kräutler579,Reference Mascarenhas, Gouda, Ruetz and Banerjee580) . Co (I) is unstable and acts as a supernucleophile. The corrin ring helps to stabilise this form(Reference Smith, Warren and Refsum6).
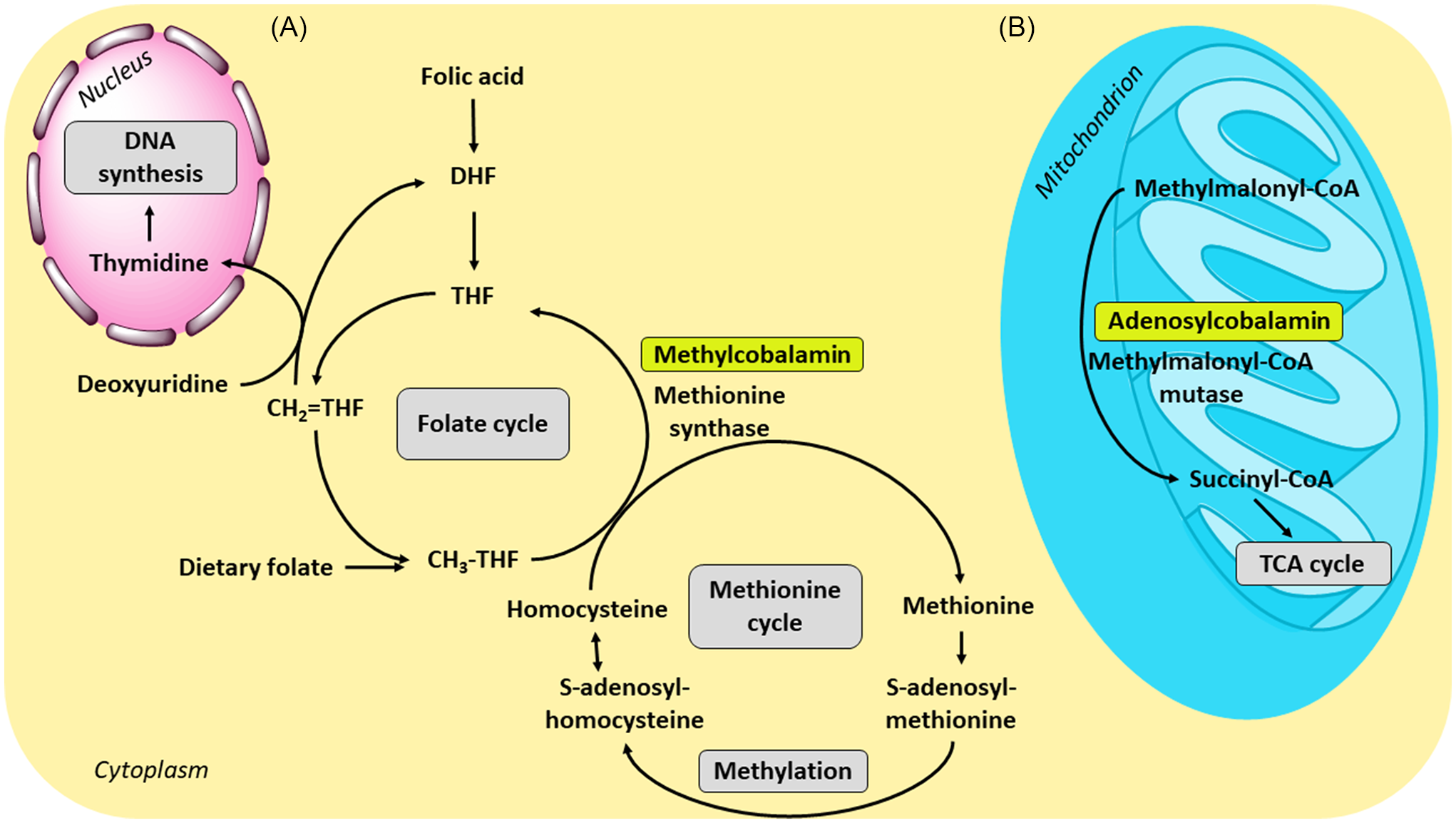
Fig. 3. Physiological function of vitamin B12 and its connection with folate metabolism: (A) Together with folic acid (vitamin B9), methylcobalamin as a cofactor for the enzyme methionine synthase is necessary for the formation of methionine. During the reaction, the methyl group is transferred from methyltetrahydrofolate (CH3-THF) to homocysteine by the enzyme; the resulting tetrahydrofolate can be then converted to methylenetetrahydrofolate (CH2=THF), the form required for de novo thymidine synthesis. (B) In the conversion of methylmalonyl-coenzyme A to succinyl-coenzyme A, B12 is involved in its active form adenosylcobalamin as a cofactor of the enzyme methylmalonyl-coenzyme A mutase. The resulting succinyl-coenzyme A is a major mediator of the tricarboxylic acid (TCA) cycle; CoA, coenzyme A; DHF, dihydrofolate; THF, tetrahydrofolate.
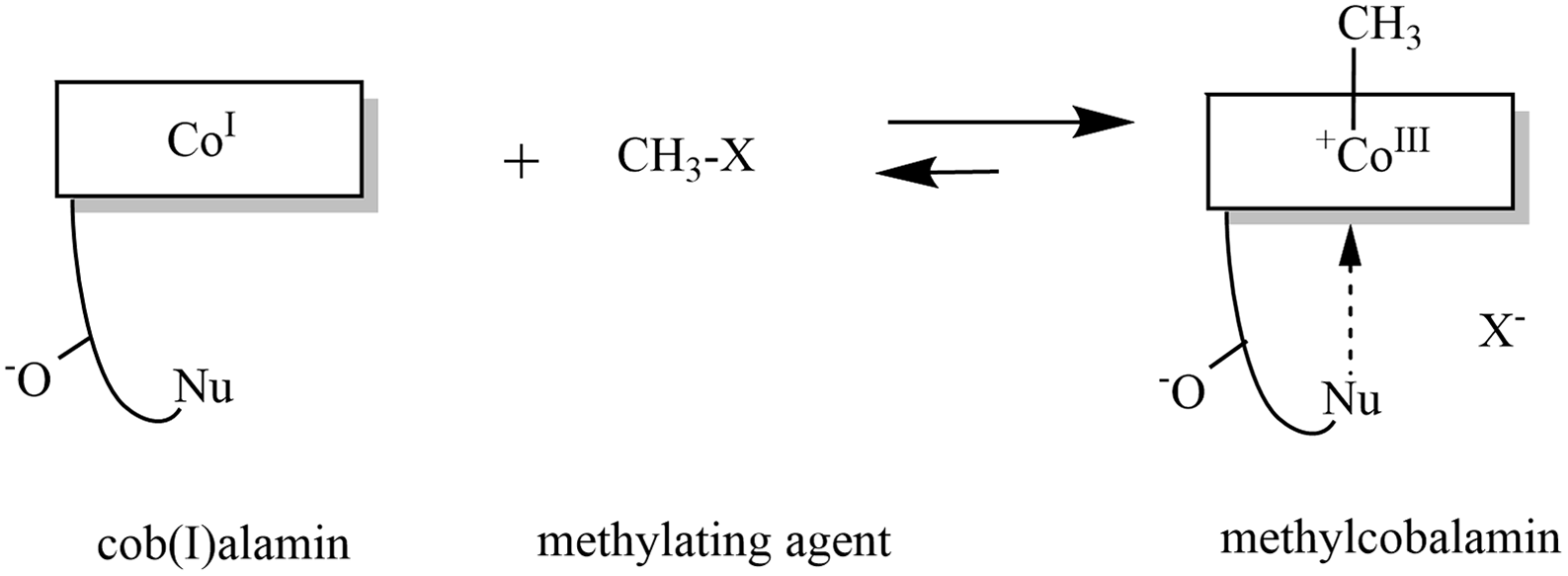
Fig. 4. Formation of methylcobalamin: The highly nucleophilic cob(I)alamin reacts with a methylating agent to form methylcobalamin. Modified in ChemDraw, version 20.0 on the basis of publication of Kräutler(Reference Kräutler579).
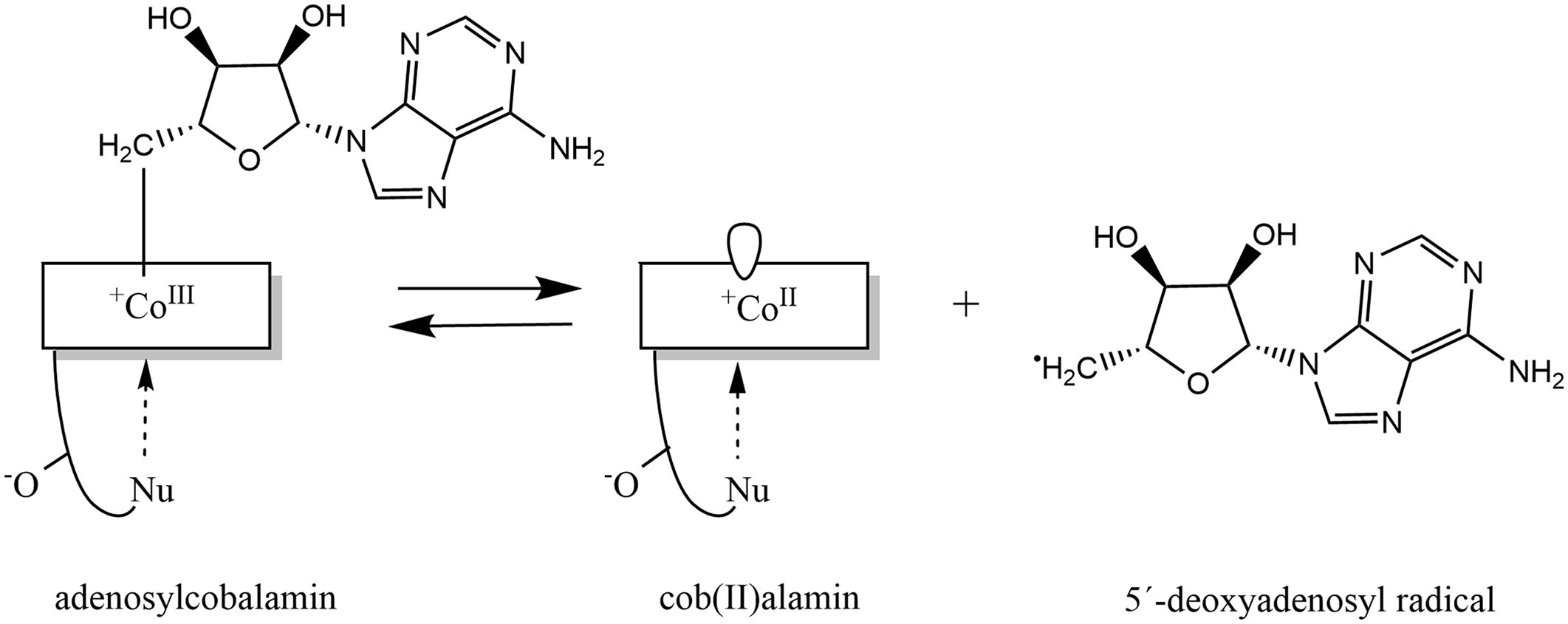
Fig. 5. Formation of adenosylcobalamin: Adenosylcobalamin functions as a reversible source of the 5′-deoxyadenosyl radical, this reaction produces cob(II)alamin. Modified in ChemDraw, version 20.0 on the basis of publication of Kräutler(Reference Kräutler579).
Methionine synthase
Methionine synthase is a cytoplasmic enzyme requiring both vitamin B12 and vitamin B9. It converts homocysteine into methionine by transfer of a methyl group. The donor of the methyl group is methylcobalamin, which is subsequently recovered by transfer of one carbon unit from methylenetetrahydrofolate (vitamin B9). It need not be emphasised that mutation in methionine synthase or derangement in vitamin B12 physiology leads to hyperhomocysteinaemia(Reference Watkins, Ru and Hwang581). High plasma homocysteine levels are considered vasculotoxic and neurotoxic, but the relationship between human diseases, homocysteine levels and supplementation by B vitamins to decrease the homocysteine levels it is still a matter of debate(Reference Kim, Kim, Roh and Kwon582,Reference McCaddon and Miller583) . Furthermore, the formation of the essential amino acid methionine allows several methylation reactions necessary for the synthesis of nucleotides for DNA/RNA and proteins. Failure of this step can be observed especially in rapidly multiplying cells, such as erythrocytes or enterocytes, and can affect several processes including the growth of vascular endothelial cells or the production of noradrenaline, which is involved in both stress response and cardiovascular system function(Reference Allen, Miller and de Groot93,Reference Kozyraki and Cases566,Reference Allen584) .
Methylmalonyl-coenzyme A mutase
The mitochondrial enzyme methylmalonyl-coenzyme A mutase is involved in the catabolism of odd-chain fatty acids, some branched-chain amino acids and cholesterol to form succinyl-coenzyme A. A defect in this response is thought to be involved in several neurological manifestations of vitamin B12 deficiency, including movement disorders, seizures and mental retardation(Reference Allen, Miller and de Groot93,Reference Kozyraki and Cases566,Reference Allen584) .
Laboratory assessment of vitamin B12 status
Vitamin B12 status can be assessed by measuring the serum/plasma B12 level, which is the sum of B12 TCI-bound (holohaprocorrin) and TCII-bound (holotranscobalamin); serum holotranscobalamin (holoTC) concentration; serum/plasma methylmalonic acid (MMA) concentration; and total serum homocysteine (tHcy) concentration(4,Reference Harrington585) . However, no single laboratory marker is suitable for assessing B12 status in all patients. Combinations, such as multiple markers or sequential assay selection algorithms(Reference Harrington585) or the calculations(Reference Fedosov586), that combine the single B12 diagnostic indicators, such as combined indicator (cB12), which uses all four most commonly available markers: cB12 = log10[(holoTC × total serum B12 level)/(MMA × tHcy)] − (age factor), are usually employed for more precise determination of vitamin B12 levels(Reference Harrington585,Reference Fedosov, Brito and Miller587) . Analytical methods used for the measurement of vitamin B12 in serum/plasma and other biological fluids are summarised in Table 2.
Table 2. Summary of analytical methods for the assessment of vitamin B12 in biological fluids
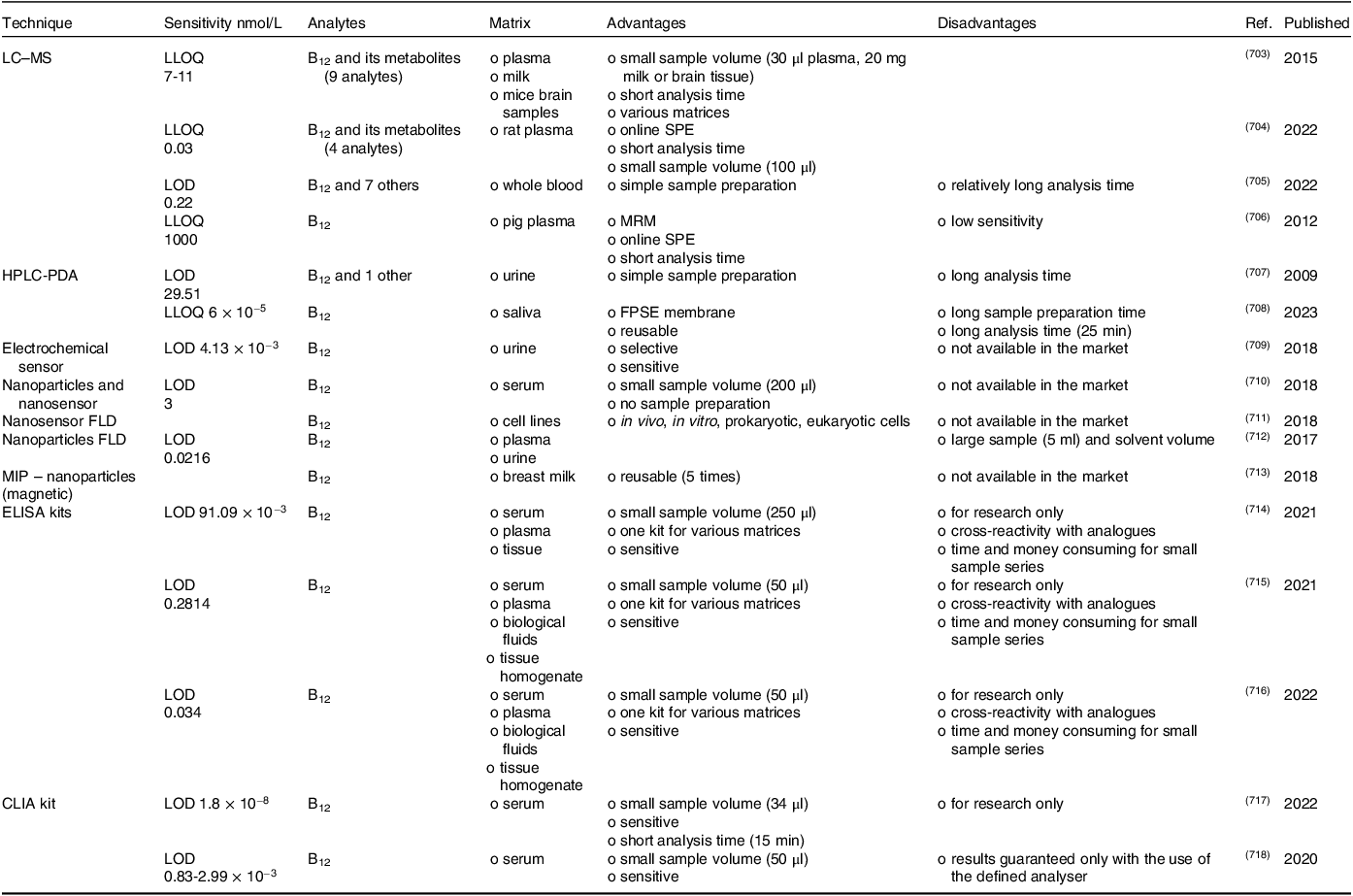
Determination of total serum/plasma cobalamin
Serum or plasma B12 levels provide information on the long-term B12 status and liver stores. Recent intake has no particular effect. This marker is not very sensitive or specific, leading to a false positive and negative diagnosis. A serum level of vitamin B12 below 148 pM with symptoms is a strong indicator of deficiency, but symptoms might be present even with serum levels above this value(Reference Wolffenbuttel, Owen, Ward and Green2). Conditions that increase TCI levels such as chronic granulocytic leukaemia, autoimmune lymphoproliferative syndrome, alcoholism, liver disease and cancer will also elevate vitamin B12 levels(Reference Allen, Miller and de Groot93).
Determination of holotranscobalamin
Serum holoTC levels determine the amount of the physiologically active form of cobalamin bound to TCII. As aforementioned, holoTC accounts for 20–30% of total B12 serum levels. This method is the most sensitive to recent intake with a response within few hours. Hence, this marker can be increased even when body reserves are low. HoloTC levels are, however, also increased in patients with renal impairment(Reference Allen, Miller and de Groot93).
Determination of methylmalonic acid
Serum MMA is the most sensitive biomarker of B12 status. It is a good indicator of liver stores, and it reflects the utility of vitamin B12 for metabolic functions, i.e. methylmalonyl CoA mutase activity. MMA as a by-product of methylmalonyl CoA metabolism increases in B12 deficiency. When total serum B12 is <287 pM, its concentrations increase. MMA metabolite is affected neither by folate nor other B vitamins, but it increases with renal dysfunction, so serum creatinine should be measured, especially in the elderly. MMA levels increase with aging, especially after age 70, and neither lower intake nor impaired renal function fully explains why this occurs. Higher serum concentrations of MMA occur also with an overgrowth of intestinal bacteria that produce propionic acid. Conversely, antibiotic treatment may lower the level(Reference Allen, Miller and de Groot93).
Determination of total serum homocysteine
The biomarker tHcy is not specific for B12 levels. It reflects the availability of B12 for metabolic functions, i.e. methionine synthase activity. Concentrations increase when total serum B12 is <300 pM, but also in deficiency of folates, riboflavin and vitamin B6, as well as with renal insufficiency and hypothyroidism(Reference Allen, Miller and de Groot93).
Combination of biomarkers
The combination of all four biomarker values (serum B12 levels, holoTC, tHcy and MMA) or the cB12 mathematical model using ‘four-biomarker’ analysis appears to be the most accurate for determining B12 levels, but in some cases is unbearably expensive(Reference Allen, Miller and de Groot93). A study by Fedosov et al.(Reference Fedosov, Brito and Miller587) showed that the cB12 model using analysis of ‘three-biomarker‘or ‘two-biomarker’ estimates the level of B12 within acceptable error limits compared with the analysis of ‘four-biomarker’. The combination of holoTC, MMA and total serum B12 levels appears to be the best in the ‘three-biomarker’ analysis. Further quality analysis is achieved by omitting total serum B12 or holoTC from the ‘four-biomarker’ analysis. In terms of ‘two-biomarker’ analysis, the smallest error was observed when holoTC was used in conjunction with the MMA assay. The article by Fedosov et al.(Reference Fedosov, Brito and Miller587) also provides formulas for cB12 for cases where one or two biomarkers are missing.
Identification of aetiology of vitamin B12 deficiency
A formerly used Schilling test was withdrawn mainly due to concerns of bovine spongiform encephalopathy transmission via its animal-derived intrinsic factor(Reference Harrington585,Reference Carmel588) . Another way to detect a deficiency caused by impaired absorption is the CobaSorb test, which uses holoTC as a sensitive marker of the recent intake of B12. The test consists of taking blood samples before and 1–2 d after taking an oral dose of 9 µg of cyanocobalamin three times a day and measuring the increase in holoTC(Reference von Castel-Roberts, Morkbak and Nexo589). This method can detect holoTC elevation of ≥10 pM only if the holoTC baseline was <75 pM. Under these conditions, both sensitivity and specificity are considered excellent(Reference Hvas, Morkbak and Nexo590). Hardlei et al. improved this method. After stating that a major part of the oral test dose of cyanocobalamin is absorbed without modification, it was suggested that the capacity to absorb vitamin B12 can be evaluated by measuring TC-cyanocobalamin before and after administration of the test dose of cyanocobalamin. C-Cobasorb, as this method is named, has a higher specificity than the previously mentioned CobaSorb(Reference Hardlei, Mørkbak and Bor591). Therefore, if holoTC baseline is >65 pM, it is recommended to use the C-CobaSorb assay for the assessment(Reference Nexo and Hoffmann-Lücke592). Other laboratory tests usable for determination of the cause of B12 deficiency are tests based on plasma IF antibodies, plasma gastrin and pepsinogen I, and plasma parietal cell antibodies(Reference Harrington585,Reference Alonso Nr and Salinas593) .
Cobalamin deficiency
Subclinical and clinical cobalamin deficiency
Low levels of vitamin B12 can be divided into four stages. The first two stages represent depletion, while the second two represent deficiency. Stage I is low serum vitamin B12; stage II is low stores in cells; stage III is biochemical deficiency; and stage IV is clinical deficiency with overt manifestations(Reference Herbert594). Other classification divides vitamin B12 deficiency into clinical and subclinical forms. Subclinical cobalamin deficiency involves mild biochemical changes without clinical manifestations. Clinical deficiency usually results from severe, persistent malabsorption, while dietary insufficiency, intermittent or partial malabsorption can usually merely induce only subclinical cobalamin deficiency(Reference Carmel595).
At the cellular and molecular level, vitamin B12 deficiency manifests itself in one or both forms of coenzyme B12 (methylcobalamin and adenosylcobalamin). Methylcobalamin deficiency leads to impaired nucleotide synthesis and methylation, and adenosylcobalamin deficiency disrupts the metabolism of methylmalonate, which is derived from the catabolism of odd-chain fatty acids and ketogenic amino acids(Reference Allen, Miller and de Groot93,Reference Green, Allen and Bjørke-Monsen260) . This can also be the key to understanding the mechanisms responsible for clinical manifestations.
Causes of vitamin B12 deficiency and diagnosis
B12 deficiency can be caused by several pathophysiological processes. They can affect both B12 supply and demand, and they can occur at any time during human life. Specifically, cellular B12 deficiency could be due to insufficient intake and/or bioavailability including malabsorption, chemical inactivation(Reference Flippo and Holder3) or disruption of B12 transport in the blood or intracellular uptake and metabolism. Also, some diseases, medications and bacterial overgrowth are related to B12 deficiency(Reference O’Leary and Samman5,Reference Green, Allen and Bjørke-Monsen260,Reference Herrmann and Obeid577) . It can also occur in people with increased demands, such as during major bleeding, and in pregnant or breastfeeding women, and this can impact their infants as well(Reference Green, Allen and Bjørke-Monsen260,Reference Herrmann and Obeid577) .
People from developing countries and those who do not voluntarily consume animal products because of their religion, culture or personal attitude may suffer from B12 deficiency(Reference Green, Allen and Bjørke-Monsen260,Reference Herrmann and Obeid577) . In terms of new vegans, the symptoms of vitamin B12 deficiency may not appear for several years owing to the large reserves in the human body and reabsorption of the vitamin(Reference Shipton and Thachil569,Reference Herrmann and Obeid577) . Lacto- and lacto-ovo-vegetarian diet could be sufficient thanks to milk and eggs that contain a low amount of cobalamin(Reference Herrmann and Obeid577). Metabolic and clinical signs of cobalamin deficiency have been reported in neonates from strict vegetarian mothers or in breastfed infants by cobalamin-deficient mothers(Reference Kozyraki and Cases566,Reference Higginbottom, Sweetman and Nyhan596,Reference Monsen, Ueland and Vollset597) . To avoid the risk of deficiency, they can obtain cobalamin from fortified foods (see section Prevention by fortification of food) or food supplements(Reference O’Leary and Samman5,Reference Green, Allen and Bjørke-Monsen260) .
Furthermore, the deficiency occurs because of cobalamin malabsorption. In the case of pernicious anaemia (chronic atrophic gastritis type A), cobalamin cannot be absorbed because of the lack of IF. It is a consequence of autoimmune gastritis when the parietal cells producing IF and hydrochloric acid in the stomach are destroyed. Chronic atrophic gastritis type B, which is related to persistent infection with Helicobacter pylori, may also impair vitamin B12 absorption. Both are due to disturbance of the pH of the stomach and the inability to release vitamin B12 from food proteins(Reference Green, Allen and Bjørke-Monsen260,Reference Kozyraki and Cases566,Reference Herrmann and Obeid577) . Proton pump inhibitors, H2-receptor antagonists and antacids also suppress the gastric acidity and, hence, similarly impair vitamin B12 absorption(Reference Green, Allen and Bjørke-Monsen260,Reference Shipton and Thachil569,Reference Herrmann and Obeid577,Reference Manzanares and Hardy578) . Diseases affecting the small intestine such as coeliac disease, Crohn’s disease or ulcerative colitis are connected with B12 malabsorption due to villous atrophy and mucosal injury or recurrent diarrhoea(Reference O’Leary and Samman5,Reference Green, Allen and Bjørke-Monsen260,Reference Shipton and Thachil569,Reference Herrmann and Obeid577) . Last but not least, cobalamin malabsorption is associated with gastric, post-gastric or ileal resection, or pancreatic insufficiency or pancreatectomy that cause inability of B12 release from HC binding(Reference O’Leary and Samman5,Reference Green, Allen and Bjørke-Monsen260,Reference Shipton and Thachil569) . Another disease associated with low levels of cobalamin is HIV infection. Plasma concentrations decrease with disease progression(Reference Allen66). In addition, older people are at risk of malabsorption not only because of the various diseases and medications they take, but also because of the natural aging process. Several age-related physiological factors can adversely affect the absorption of the vitamin from the intestine(Reference Herrmann and Obeid577).
In addition to the above-mentioned drugs, low levels of vitamin B12 are also associated with oral contraceptives(Reference Berenson and Rahman598), metformin, cholestyramine, colchicine, several antibiotics, and drugs such as p-aminosalicylic acid(Reference Green, Allen and Bjørke-Monsen260,Reference Manzanares and Hardy578) and in elderly patients also with ACE inhibitors(Reference Tal, Shavit, Stern and Malnick599). In some, the mechanism is known as with cholestyramine that may bind intrinsic factor, while colchicine, several antibiotics, and antituberculotic drug p-aminosalicylic acid, may act as inhibitors of intrinsic factor-B12 endocytosis(Reference Green, Allen and Bjørke-Monsen260).
In terms of the chemical inactivation, the anaesthetic gas nitrous oxide irreversibly oxidates cobalt. This oxidation is, however, relevant solely the enzyme methionine synthase(Reference Flippo and Holder3,Reference Green, Allen and Bjørke-Monsen260,Reference Chanarin600) . On the other hand, adenosylcobalamin is not changed. The reason is that mechanisms of the catalytic reaction differ between methionine synthase and methylmalonyl-coenzyme A mutase particularly in relation to changes in the oxidation state of the central cobalt ion. During methionine synthesis, cob(I)alamin intermediate, which is susceptible to oxidation, is formed, whereas during methylmalonyl-succinyl coenzyme A transformation, cobalt is never reduced to Co(I) and, thus, not susceptible to N2O-induced oxidation. (Reference Mascarenhas, Gouda, Ruetz and Banerjee580,Reference Chanarin600) .
Moreover, there are some rare genetic defects causing impairment in absorption, transport, metabolism or utilisation of cobalamin. Hereditary IF deficiency caused by recessive mutations in the IF gene (CBLIF) is an inherited disorders of vitamin B12 absorption associated with congenital pernicious anaemia. Imerslund–Gräsbeck syndromes 1 and 2 (also known as hereditary megaloblastic anaemia 1 and 2) are associated with selective vitamin B12 malabsorption in the ileum; there is a defect in transport of cobalamin into the enterocytes due to mutation in cubilin (gene CUBN) and amnionless (gene AMN), respectively. With respect to the physiological function of TCI (gene TCN1) and TCII (gene TCN2), it is clear that congenital deficiency of at least one of them leads to defective absorption as well as transport into cells. In addition, mutations in TCII receptor CD320 are known. There is also a group of historically so-called cobalamin mutant diseases cblA, cblB, cblC, cblD, cblE, cblF, cblG, cblJ, cblK and cblX. These diseases affect different processes associated with vitamin B12: (1) efflux from lysosomes to cytosol (cblF – gene LMBRD1, cblJ – gene ABCD4); (2) intracellular vitamin B12 trafficking via chaperone MMACHC (cblC, /gene MMACHC/), silencing its gene (‘epi-cbl C’, gene PRDX1), or proteins regulating its expression – cblX /gene HCFC1 on X chromosome/ and cblK /gene ZNF143/; (3) likely transport to methionine synthase – cblD /gene MMADHC/; (4) metabolism by methionine synthase (cblG, methione synthase, gene MTR) or methionine synthase reductase (cblE, gene MTRR); (5) synthesis of adenosylcobalamin by ATP:cobalamin adenosyltransferase /cblB, gene MMAB/ and transport of produced adenosylcobalamin to methylmalonyl CoA mutase /cblA, gene MMAA/. Methylmalonyl CoA mutase deficiency (gene MMUT) is also known(Reference Kozyraki and Cases566,Reference Watkins and Rosenblatt571,Reference Herrmann and Obeid577,Reference Watkins, Ru and Hwang581,Reference Rosenblatt and Cooper601) .
The reference intervals of the individual biomarkers as well as their values indicating B12 deficiency are given in Table 3 (Reference Green, Allen and Bjørke-Monsen260,Reference Fedosov, Brito and Miller587) . To determine the aetiology of vitamin B12 deficiency, the (C-)CobaSorb assay or plasma IF antibodies, plasma gastrin and pepsinogen I, and plasma parietal cell antibodies (Reference Harrington585,Reference Alonso Nr and Salinas593) can be used, as mentioned above.
Table 3. The reference intervals of the individual biomarkers, values indicating transitional status and B12 deficiency

B12, total serum B12 concentrations; cB12, combined indicator of vitamin B12 status.
Clinical manifestations
Clinical deficiency is manifested by haematological, neurological and neuropsychiatric symptoms(Reference Wolffenbuttel, Owen, Ward and Green2,Reference Green, Allen and Bjørke-Monsen260,Reference Stabler441,Reference Shipton and Thachil569,Reference Herrmann and Obeid577,Reference Dali-Youcef and Andrès602–Reference Reynolds604) . No specific correlation between haematological and neurological symptoms has been demonstrated, so patients with neurological manifestations may not have any haematological abnormalities and vice versa(Reference Allen, Miller and de Groot93,Reference McCaddon605) .
Haematological manifestations are usually but not always connected with macrocytic or megaloblastic anaemia that is characterised by enlarged but less numerous erythrocytes, and by hypersegmented neutrophils. It is the result of disruption of DNA synthesis. In B12 deficiency, the recovery of tetrahydrofolate is disrupted. This limits the supply of folate for the synthesis of thymidylate, purine nucleotides and, subsequently, DNA. The haematopoietic system is particularly affected because blood cells are cells with a rapid turnover. DNA synthesis in bone marrow blood cell precursors is stopped, which prevents mitosis but allows cytoplasmic maturation(Reference Allen, Miller and de Groot93,Reference Green, Allen and Bjørke-Monsen260) . Because other haematopoietic cells are also affected, it can lead to isolated thrombocytopenia and neutropenia and even pancytopenia with impairment of cellular and humoral immunity(Reference Allen, Miller and de Groot93,Reference Green, Allen and Bjørke-Monsen260,Reference Stabler441,Reference Shipton and Thachil569,Reference Dali-Youcef and Andrès602) .
Cobalamin deficiency appears to be more common in patients with a number of chronic neurological and neuropsychiatric diseases. Clinical manifestations of vitamin B12 deficiency can include several CNS symptoms (peripheral neuropathy, subacute combined degeneration of the spinal cord, paraesthesia, ataxia, abnormal reflexes, bowel and bladder incontinence, erectile dysfunction, stroke, optic atrophy, orthostatic hypotension, dementia, multiple sclerosis, Alzheimer’s disease, parkinsonian syndromes, depression, mania, irritability, paranoia, delusions, psychosis, delirium), but also atherosclerosis. However, it is still not clear whether there is a direct causality(Reference Stabler441,Reference Shipton and Thachil569,Reference Herrmann and Obeid577,Reference Dali-Youcef and Andrès602,Reference Lachner, Steinle and Regenold603) . There are several theories that link cobalamin deficiency with neurological and neuropsychiatric problems such as (a) disorders of the formation of monoamine neurotransmitters, because cobalamin and folate are essential for the production of tetrahydrobiopterin that is required for monoamine synthesis(Reference Hutto606); (b) DNA synthesis disturbances due to deficiency of methyl donors(Reference Reynolds604); (c) hyperhomocysteinaemia and its discussed vasculotoxic and neurotoxic effects(Reference McCaddon and Miller583,Reference Reynolds604,Reference Obeid, McCaddon and Herrmann607,Reference Norbert Goebels and Michael Soyka608) ; (d) demyelination due to a lack of S-adenosylmethionine as a consequence of inhibition of homocysteine methylation to methionine(Reference Reynolds604,Reference Norbert Goebels and Michael Soyka608,Reference Metz609) ; (e) inhibition of the methylmalonyl-succinyl CoA pathway causes disruption of odd–chain fatty acid metabolism and may also lead to demyelination(Reference Reynolds604,Reference Metz609) .
In addition, there is a higher risk for infants born to B12-deficient women to develop neural tube defects with impairment of psychomotor function and brain development, anaemia and growth disorders. Neural disturbances may be irreversible(Reference Niklewicz, Smith and Smith244,Reference Finkelstein, Layden and Stover610) .
Prevention by fortification of food
Fortification of foods with vitamin B12 is primarily intended for populations at risk for its deficiency. The highest prevalence of deficiency occurs in people who have a low dietary intake of the vitamin and in those, primarily the elderly, suffering from malabsorption of food-bound cobalamin, whose vitamin B12 status can be poor despite intakes often appearing to be adequate. As already discussed, vegetarian diets also represent a clear risk factor for vitamin B12 deficiency, as those diets have become very popular in the past few decades, especially in developed countries, because of their potential health benefits but also due to ethical and environmental issues. The diets of populations in low- and middle-income countries are typically low in animal-source foods because of their relatively high cost, lack of availability, and/or cultural and religious reasons(Reference Allen66,Reference Sobczyńska-Malefora, Delvin and McCaddon81,Reference Jiang, Christian and Khatry611–Reference Thankachan, Rah and Thomas613) . Reduced acid production in the stomach associated with atrophic gastritis does not interfere with the absorption of the free crystalline vitamin present in fortified foods or supplements because intrinsic factor is still secreted(63,Reference Allen66,Reference Sobczyńska-Malefora, Delvin and McCaddon81,Reference Watanabe, Yabuta, Tanioka and Bito85,Reference Allen, Miller and de Groot93,Reference Truswell137,Reference Stabler, Bernadette, Marriott and Yates152,Reference Tucker, Olson and Bakun199,Reference Winkels, Brouwer and Clarke200,Reference Melina, Craig and Levin205,Reference Green, Allen and Bjørke-Monsen260,Reference Carmel455,Reference Yates565,Reference Allen, Rosenberg, Oakley and Omenn612,Reference Allen614–Reference Oakley623) . Fortification with cobalamin has also been recommended in foods fortified with folate because of concerns that excess intake of folic acid alone by people with a low vitamin B12 status, particularly in the elderly, may delay the diagnosis of cobalamin deficiency. High levels of folate may mitigate (‘mask’) symptoms of anaemia caused by cobalamin deficiency and cannot correct nervous system damage, allowing or even accelerating its progression in persons with unrecognised and untreated cobalamin deficiency. Significance of this phenomenon has been an issue of persistent debate(63,Reference Winkels, Brouwer and Clarke200,Reference Agnoli, Baroni and Bertini242,Reference Green453,Reference Refsum and Smith615–Reference Blacher, Czernichow and Raphaöl619,Reference Oakley623–Reference Miller, Garrod and Allen656) . Whether the concerns are substantiated or not(Reference Obeid, Heil and Verhoeven108,Reference Carmel456,Reference Allen, Rosenberg, Oakley and Omenn612,Reference Mills, Molloy and Reynolds627,Reference Brouwer and Verhoef639,Reference Berry657–Reference Crider, Bailey and Berry666) , cobalamin deficiency should be prevented in any case, and fortification with both folate and cobalamin would reduce possible risks. As of 2022, there are twenty-five countries in Africa, Central and South America, and Asia having mandatory fortification of wheat flour, maize flour or rice with vitamin B12 (Burundi, Cameroon, Chad, Ethiopia, Ghana, Kenya, Liberia, Malawi, Mozambique, Nigeria, Rwanda, Tanzania, Uganda, Zimbabwe, Costa Rica, Cuba, Guatemala, Nicaragua, Panama, Peru, Afghanistan, Jordan, Palestine, Uzbekistan and Vietnam)(667–Reference Bobrek, Broersen and Aburto670). In addition, food is fortified with the vitamin on a voluntary basis in many other countries including, e.g., the United States, Brazil, India, Bangladesh, Myanmar, Indonesia, Sudan, Sierra Leone and countries of the European Union(Reference Zeuschner, Hokin and Marsh224,444,449,Reference Calvillo, Pellicer, Carnicer and Planas450,Reference Hopkins, Gibney and Nugent461,Reference Titcomb and Tanumihardjo521,667,668,Reference Muthayya, Hall and Bagriansky671–Reference Laird, O’Halloran and Carey674) . Foods fortified with vitamin B12 include cereal-based foods, meat substitute products (such as tofu), milk substitutes (such as soy-based milk and yoghurt alternatives), nutritional yeast, fruit juices, multivitamin sweets and milk powder infant foods(Reference Watanabe, Yabuta, Tanioka and Bito85,Reference Allen, Miller and de Groot93,Reference Stabler, Bernadette, Marriott and Yates152,Reference Tucker, Olson and Bakun199,Reference Sebastiani, Herranz Barbero and Borrás-Novell216,Reference Zeuschner, Hokin and Marsh224,Reference Chandra-Hioe, Lee and Arcot256,Reference Marczykowski and Breidenassel259,444,449,Reference Allen, Rosenberg, Oakley and Omenn612,Reference Thankachan, Rah and Thomas613,Reference Garrod, Buchholz and Miller636,Reference Engle-Stone, Nankap and Ndjebayi669,Reference Muthayya, Hall and Bagriansky671,Reference Sirohi, Pundhir and Ghosh672,Reference Dunn, Jain and Klein675–Reference Madhari, Boddula and Ravindranadh679) . Attractive strategies for fortification have been investigated, such as fortification of milk (Reference Dhonukshe-Rutten, van Zutphen and de Groot616,Reference Sanchez, Albala and Lera621) , yoghurt(Reference Melo, Ng and Tsang680), tea (Reference Vora, Alappattu and Zarkar681,Reference Vora and Antony682) , fruit and vegetable juices(Reference Bajaj and Singhal388), ready-to-blend fresh-cut fruit/vegetable mix(Reference Artés-Hernández, Formica-Oliveira, Artés and Martínez-Hernández683), mineral water(Reference Tapola, Karvonen, Niskanen and Sarkkinen684), sugar cubes(Reference Bajaj and Singhal438), salt (Reference Modupe and Diosady391,Reference Vinodkumar and Rajagopalan439) , dried soup(Reference Sanchez, Albala and Lera621) and toothpaste(Reference Zant, Awwad and Geisel685,Reference Siebert, Obeid and Weder686) .
Therapy
The first-line treatment in patients with risk factors for the vitamin deficiency is prevention of this deficiency and/or supplementation with vitamin B12. Treatment should be started as soon as possible(Reference Green, Allen and Bjørke-Monsen260). Parenteral therapy or high-dose oral administration can be used to treat vitamin B12 deficiency if due to inadequate dietary intake. In diagnosed vitamin B12 deficiency, intramuscular administration should be preferred as the effect or oral treatment is lower based on current knowledge(Reference Wolffenbuttel, Owen, Ward and Green2). Intramuscular injections of 1000 μg of cyanocobalamin or hydroxocobalamin are given daily or every other day for at least one week followed by weekly injections for at least one month. Thereafter, they are reduced to a dose of 1000 μg of cyanocobalamin monthly. Injections of hydroxocobalamin can be given each 3 months after the initial intensive therapy. Alternatively, subcutaneous administration is recommended in cases of contraindications of intramuscular application, e.g. when a patient is treated with anticoagulants. Oral treatment consists of 1000–2000 μg of cyanocobalamin orally per day. In some countries, also sublingual and intranasal vitamin B12 is available, but their clinical profit has not yet been clearly established(Reference Wolffenbuttel, Owen, Ward and Green2,Reference Green, Allen and Bjørke-Monsen260,Reference Stabler441,Reference Dali-Youcef and Andrès602) . The development of nanoparticles for oral vitamin B12 administration able to overcome the IF-absorption pathway can enrich the palette of current treatment modalities in the future(Reference Fidaleo, Tacconi and Sbarigia452).
The duration of treatment depends on the cause and clinical manifestations of the deficit. Blood count usually recovers within 2 months, and neurological signs correct or improve within 6 months(Reference Stabler441). However, in patients with severe neurological disorders, improvement usually will be weak after 1 year of adequate therapy. These patients may suffer from permanent impairments(Reference Green, Allen and Bjørke-Monsen260). In case of malabsorption, treatment continues even after the symptoms have disappeared; it is usually a life-long therapy. Both routes of administration (including self-injection at home) can be used(Reference Wolffenbuttel, Owen, Ward and Green2). Selection of the route of administration usually depends on patient preferences and the compliance. As mentioned above, other less studied routes of administration (sublingual, nasal or even transdermal) are available, but they are also more expensive(Reference Stabler441,Reference Carmel455) . Regarding dietary deficiency, it is recommended to take at least 6 μg/d after the symptoms have disappeared and the body’s vitamin B12 levels have been restored. In infants, treatment is usually started with intramuscular injections of 250–1000 μg cyanocobalamin or hydroxocobalamin daily, then until recovery once a week, followed by an oral administration of 1–2 μg daily using various B12-containing formulas. Treatment of the mothers is also indicated to adjust breast milk vitamin levels(Reference Green, Allen and Bjørke-Monsen260).
There is dose–response relationship between oral B12 dose and serum/plasma B12 levels(Reference Dullemeijer, Souverein and Doets687). Doubling vitamin B12 increases serum/plasma levels approximately by 11% and slightly more in the elderly (13%) than in adults (8%).
Pharmacological use
Vitamin B12 is mainly used, as mentioned above, to supplement at-risk people and to treat deficiency. In particular, older people who are more susceptible to food–cobalamin malabsorption should consume vitamin B12 in crystalline form, i.e. from supplements or fortified foods, as this form is likely to be better absorbed(Reference Allen, Miller and de Groot93).
It is speculated that vitamin B12 could be used as an adjunctive or integrative treatment for painful conditions such as various types of neuralgia and neuropathy, low back pain and aphthous stomatitis. Further studies are needed in this area(Reference Buesing, Costa, Schilling and Moeller-Bertram688,Reference Julian, Syeed and Glascow689) . Vitamin B12 could have a positive effect on sperm quality. It primarily increased sperm count and secondarily elevated sperm motility and reduced sperm DNA damage(Reference Banihani690). However, in both cases, further studies are needed as well.
Toxicity
To date, there are minimal claims on cobalamin toxicity. Vitamin B12 is usually well tolerated with rare incidence of adverse effects. Allergic reactions are unusual but may be anaphylactic(Reference Caballero, Lukawska, Lee and Dugué691,Reference Tordjman, Genereau and Guinnepain692) . Injectable forms appear to be more allergenic than pills, but this could be associated rather with some preservatives (e.g. benzylalcohol) in injections than by the vitamin itself(Reference Wolffenbuttel, Owen, Ward and Green2). High oral dose supplementation seems to be better tolerated, but there are known exceptions(Reference James and Warin693). Although there is a cross-reaction between hydroxocobalamin and cyanocobalamin, it is possible to reintroduce vitamin B12 with concomitant administration of glucocorticoids or antihistamines or with desensitisation therapy(Reference Caballero, Lukawska, Lee and Dugué691,Reference Tordjman, Genereau and Guinnepain692) . There are also cases of acneiform eruptions after intramuscular or high oral doses of vitamin B12, which easily disappear after discontinuation(Reference Morales-Gutierrez, Díaz-Cortés, Montoya-Giraldo and Zuluaga694,Reference Veraldi, Benardon, Diani and Barbareschi695) . Nausea, dry mouth and blurred vision were reported after administration of vitamin B12 as well(Reference Campbell, Heydarian and Ochoa696). Other rare documented side effects of vitamin B12 include discolouration of the skin and urine, mild arterial hypertension, hypokalaemia, congestive heart failure, pulmonary oedema and local pain at the injection site in case of parenteral administration(Reference Manzanares and Hardy578).
Conclusion
This review summarised all critical aspects of vitamin B12 biology. It emphasised that animal-based diets are almost the sole source of this vitamin for humans and, hence, vegetarians are at risk of developing its deficiency, which can progress to very severe and irreversible stages. At present, many countries have mandatory or voluntary fortification of food by this vitamin, but the risk of vitamin B12 deficiency is still not negligible worldwide. Moreover, there are many diseases, drugs and surgical procedures which can cause its deficiency, which is mostly delayed by several years as humans have relatively large stores of this vitamin. Prevention or treatment should be ideally case specific due to the complicated absorption mechanism of this vitamin. There are no convincing data on the pharmacological administration of vitamin B12 with exception of its deficiency. Its administration is considered safe in the majority of patients, but hypersensitive reactions can occur particularly after its parenteral administration.
Financial support
This open-access review paper was supported by the Erasmus+ Programme of the European Union, Key Action 2: Strategic Partnerships, project no. 2020-1-CZ01-KA203-078218. M.M. thanks the Charles University (SVV 260 663). L.K.K. thanks MH-CZ-DRO (UHHK, 00179906).
Competing interests
None.
Authorship
M.M. wrote the initial draft of the whole paper. T.S. prepared the parts related to sources of the vitamin, while L.K.K. and K.M. prepared that in relation to the detection of the vitamin. P.M. prepared the concept and revised the prepared paper. All authors revised the paper before submission and as a part of the peer-review process.