Introduction
Alluvial platinum-group minerals (PGM) in the western hills of the Freetown Peninsula, Sierra Leone, West Africa were first found in 1926 (Junner, Reference Junner1929, Reference Junner1930; Pollet, Reference Pollett1931, Reference Pollett1951). Artisanal mining recovered the PGM from small rivers known locally as Big Water, and the Alako in the York area (Fig. 1) and, subsequently, from Guma Water and the Babadori further to the north ( Pollet, Reference Pollett1931, Reference Pollett1951; Barber, Reference Barber1962, Forde, Reference Forde2011).
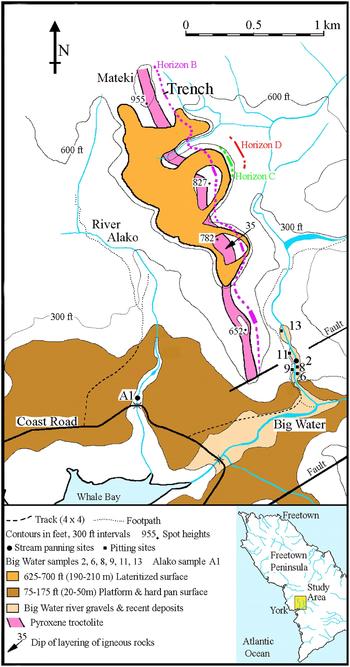
Fig. 1. Map of the York area, Freetown Peninsula, Sierra Leone showing the known occurrences of the primary PGE-bearing layers Horizon B, Horizon C and Horizon D, the main lateritized surfaces, and the sampling sites in the Alako and Big Water rivers, either side of Mateki Ridge.
The PGM from the Freetown Complex have been examined in detail from the Babadori (Pollet, Reference Pollett1951) and Guma Water areas (Pollet, Reference Pollett1931; Bowles, Reference Bowles1981, Reference Bowles1986, Reference Bowles, Prichard, Potts, Bowles and Cribb1988, Reference Bowles2000a; Bowles et al., Reference Bowles, Atkin, Lambert, Deans and Phillips1983; Hattori et al., Reference Hattori, Cabri and Hart1991, Reference Hattori, Chalokwu and Hart1996; Cabri et al., Reference Cabri, Harris and Weiser1996). The alluvial PGM consist mainly of Pt-Fe alloys. Euhedral crystals of Os-Ir alloys and laurite–erlichmanite (RuS2–OsS2) can be set in the Pt-Fe alloys or occur independently. Minor proportions of tulameenite (Pt2CuFe) are also present. Sulfides of platinum-group elements (PGE) and tellurides as well as Cu-sulfides have been reported as inclusions within the Pt-Fe nuggets. The nuggets have a coarse grain size, usually in the range 0.5 to 2 mm but considerably larger nuggets (1–2 cm) have been reported, including dendritic crystalline growths (Pollet, Reference Pollett1951).
The processes that result in the presence of the PGM in placer deposits have been the subject of debate. Two contrasting models have been proposed for the formation of PGM and the most likely situation is that both processes contribute, depending upon local conditions. The first process involves primary formation of large PGE grains within an intrusion, followed by weathering where PGM are liberated and transported mechanically with no alteration apart from surface abrasion of the nuggets (Cabri et al., Reference Cabri, Harris and Weiser1996; Weiser, Reference Weiser and Cabri2002 and references therein). This process undoubtedly occurs where large PGM are present in the host rocks and the weathering is purely mechanical as in temperate, cold and polar climates. The major problems with this proposal are the contrast in the primary and the alluvial mineral assemblages, mineral chemistry as well as the three orders of magnitude difference in the size of the PGM placer nuggets compared with those found in the igneous rock. Several authors (e.g. Oberthür et al., Reference Oberthür, Weiser and Gast2003) have been able to find rare large PGM within the host rocks and have shown that the size difference might just be a question of appropriate sampling. To date no indication of any occurrence of large PGM has been encountered in the Freetown rocks despite lithogeochemical and geomagnetic surveys of the area. Micrometre-sized PGM have been located in the rocks (Bowles, Reference Bowles2000b; Bowles et al., Reference Bowles, Prichard, Suárez and Fisher2013, Reference Bowles, Suárez, Prichard and Fisher2017) but it is the differences between the mineral assemblages in the primary and placer occurrences which are most significant (Bowles, Reference Bowles1981; Bowles et al., Reference Bowles2000a, Reference Bowles, Prichard, Suárez and Fisher2013; Oberthür et al., Reference Oberthür, Weiser, Melcher, Gast and Wöhrl2013).
The second model involves solution of PGE during weathering, possibly aided by biochemical leaching and high Eh and low pH conditions. This is followed by transport of the PGE in solution leading to supergene growth of nuggets within lateritic soils where conditions such as lower Eh and neutral pH favour deposition (Fuchs and Rose, Reference Fuchs and Rose1974; Bowles, Reference Bowles1986, Reference Bowles, Prichard, Potts, Bowles and Cribb1988; Bowles et al., Reference Bowles, Giże and Cowden1994a, Reference Bowles, Lyon, Saxton and Vaughan2000; Talovina and Lazarenkov, Reference Talovina and Lazarenkov2001; Aiglsperger et al., Reference Aiglsperger, Proenza, Zaccarini, Lewis, Garuti, Labrador and Longo2015, Reference Aiglsperger, Proenza, Font-Bardia, Baurier-Aymat, Galí, Lewis and Longo2016). The problem with this model is that the mechanisms of this process are largely unknown and the evidence circumstantial. There is clear evidence of alteration and weathering of the primary PGM leading to transport of PGE in solution (Bowles et al., Reference Bowles, Suárez, Prichard and Fisher2017). The Fe content of the altered product remains within the range encountered for the Pt-Fe alloys. The change in composition associated with the alteration of Pt-Fe alloy is principally a loss of Pt and a gain of O or OH. Bowles (Reference Bowles1986) argued that the Eh and pH of iron-rich, lateritized soils are sufficiently extreme to allow the formation of Pt-oxide but Hattori et al. (Reference Hattori, Takahashi and Augé2010) have found that alteration in New Caledonia has produced a very fine-grained mixture of relict Pt-Fe alloy and Fe-oxides or hydroxides. We have not yet examined the Freetown material in this detail so this paper uses ‘oxidized PGM’ to describe the alteration product. How can neoform growth lead to the formation of large nuggets? The contribution by Reith et al. (Reference Reith, Zammit, Shar, Etschmann, Bottrill, Southam, Ta, Kilburn, Oberthür, Bail and Brugger2016) offers a mechanism for bacterially-aided mineral growth. The nuggets are normally single crystals with no sign in polished section of the remains of grain boundaries that could suggest mechanical or chemical ‘agglutination’. The explanation for nugget formation must include early nuclei to be a preferred site for continued growth.
Occurrences of eluvial and alluvial PGM that exhibit a contrasting mineralogy to their source rocks have been reported especially in areas of long duration intense weathering such as tropical weathering of ancient cratons. Examples include placer deposits derived from ophiolitic chromitites in Borneo (Burgath, Reference Burgath, Prichard, Potts, Bowles and Cribb1988); serpentinite lateritic coatings in Cameroon (Ndjigui and Bilong, Reference Ndjigui and Bilong2010); lateritic soils covering the deeply weathered Yubdo ultramafic intrusion, Ethiopia (Belete et al., Reference Belete, Mogessie, Hoinkes and Ettinger2000, Reference Belete, Mogessie and Bowles2002), the Uktus Complex in Central Urals, Russia (Talovina and Lazarenkov, Reference Talovina and Lazarenkov2001; Zaccarini et al., Reference Zaccarini, Pushkarev, Garuti, Krause, Dvornik, Stanley and Bindi2013), supergene leaching leading to neoformation in Minas Gerais, Brazil (Cabral et al., Reference Cabral, Beaudoin, Choquette, Lehmann and Polônia2007, Reference Cabral, Galbiatti, Kwitko-Ribeiro and Lehmann2008) and PGM neoformation in Ni-laterites, Dominican Republic (Aiglsperger et al., Reference Aiglsperger, Proenza, Zaccarini, Lewis, Garuti, Labrador and Longo2015, Reference Aiglsperger, Proenza, Font-Bardia, Baurier-Aymat, Galí, Lewis and Longo2016, Reference Aiglsperger, Proenza, Galí, Rius, Longo and Domènech2017). Evidence from the rivers draining The Great Dyke in Zimbabwe (Oberthür et al., Reference Oberthür, Weiser, Melcher, Gast and Wöhrl2013) provides a view of the process from start to finish. Here PGE-bismuthotellurides and sulfarsenides found in the pristine sulfide-bearing zone and oxidized PGM in the oxide zone (Oberthür et al., Reference Oberthür, Weiser and Gast2003) are absent from the alluvium. Pt-Fe alloys occur instead, the majority of which are considered to be neoformations.
It is relevant that the Freetown intrusion has undergone a very long period of weathering. Wave-cut platforms indicate erosion and weathering have been active since the Eocene (Gregory, Reference Gregory1962). In addition, the peninsula is exposed to very heavy rainfall, which is currently 3000–5000 mm/year (Hughes and Hughes, Reference Hughes and Hughes1992). The source of the Freetown placer PGM was obscure until recently, so comparison of the source, the eluvial deposits and the placers was not possible. Bowles (Reference Bowles2000b) and Bowles et al. (Reference Bowles, Prichard, Suárez and Fisher2013) defined four stratiform layers of magnetite-bearing gabbros with PGE mineralization (up to 0.69 g/t Pt) and Cu-rich sulfides describing a primary source of the Freetown PGM and identifying primary PGM for the first time. The host rocks are fresh with coarse-grained olivine, plagioclase, clinopyroxene, orthopyroxene and later, interstitial, amphibole (Bowles et al. Reference Bowles, Prichard, Suárez and Fisher2013). There has been re-equilibration of the Fe-Ti-oxides during cooling but no indication of significant metamorphism or serpentinization. The PGM occur in the interstices between the main silicate minerals and within the late amphibole. The PGM in the host rocks are considered here to be ‘primary PGM’, the PGM that have been altered by weathering are described as ‘weathered PGM’ and the different alluvial suite are called ‘neoform PGM’.
Bowles et al. (Reference Bowles, Suárez, Prichard and Fisher2017) investigated the sequence of mineralogical changes undergone by the primary PGE mineralization during in situ weathering. The present contribution uses results from a systematic survey of the alluvial PGM from the river terraces and the beds of the Alako and the Big Water (Fig. 1) that drain PGE-bearing horizons to compare the PGM in the primary and weathered rocks with those in the alluvial assemblage to assess the origin of the secondary PGM.
Platinum from the Freetown Peninsula
In 1926 Pt-bearing grains were found in the westward draining streams of the complex and, by 1949, 5242 oz of platinum concentrate had been recovered. Analysis of the concentrate showed Pt 87, Pd 2, Os + Ir 1.3 and Fe 9.9 wt.% (Pollett, Reference Pollett1931). The extraction used artisanal methods with sluice boxes and local labour panning by hand. It is worth quoting the description of the alluvial occurrences from Pollett (Reference Pollett1931). “Platinum in the native state occurs in the gravels of streams draining the hills forming the peninsula of Sierra Leone. To a limited extent it has also been found in beach gravels in certain coast localities. The most promising deposits are in the vicinity of York, more specifically in the streams flowing into Whale River on its northern banks. Here the metal occurs as small nuggets, pitted and water worn grains, delicate dendritic crystalline growths and dust. Occasionally cubic crystals are found. Many of the small nuggets and water-worn grains have a peculiar brass colour. The largest nugget yet recovered weighed 121/2 dwt., and numerous nuggets ranging between 5 and 101/2 dwt. have been found.” [1 dwt or one pennyweight is 20 g, so the weights mentioned range from 10 to 250 g]. “These larger nuggets are invariably well water worn. An interesting feature is the presence of practically non water worn, delicate dendritic crystalline growths of platinum in gravels that also contain well-rounded grains of the metal. This dendritic non water worn form, however, is rarely seen in fragments larger than 1/2 dwt. Another noticeable feature is the small percentage of platinum dust.”
The platinum described as being in the native state is now known to be platinum-iron alloy, occurring as PtFe and Pt3Fe (Bowles, Reference Bowles1981). The density of isoferroplatinum (Pt3Fe) is 18.2 g/cc and that of tetraferroplatinum (PtFe) is 15.2 g/cc. For the purposes of an illustrative calculation an intermediate value of 16 g/cc can be chosen so the ‘largest nugget yet recovered’ (121/2 dwt.) could be represented by a cube with an edge of 2.5 cm! The numerous nuggets of 5 to 101/2 dwt. would have had a volume corresponding to cubes with edges of 1.8 to 2.4 cm and the dendritic growths reached a volume equivalent to a cube with an edge of 0.85 cm.
Experiments with nuggets from the present study have shown that the ‘brass colour’ of the PGM is a coating of Fe- and Al-oxides and organic matter that can be removed by washing in warm 6M HCl for 1 to 2 days and then reacting with 30% H2O2 for about one month. The coating hides the otherwise silvery colour of the nuggets making them difficult to locate in the sediments. This coating appears comparable with the biofilms identified by Reith et al. (Reference Reith, Zammit, Shar, Etschmann, Bottrill, Southam, Ta, Kilburn, Oberthür, Bail and Brugger2016).
The description of the morphology of the PGM by Pollett (Reference Pollett1931) is comparable with the PGM from Guma Water, Freetown described by Bowles (Reference Bowles1981). The ‘small percentage of platinum dust’ may be a result of panning and hand picking of the grains in the presence of a large amount of coarse black sand so that fine-grained PGM would not have been recovered.
Pollett (Reference Pollett1951) suggested that artisanal mining had ceased because of a low platinum price and it was unclear if economic PGM concentrations remained. Therefore, exploratory surveys of the alluvium were undertaken by panning in the streams and by pitting of the river terraces to assess the present situation, and also to aid the search for the hard-rock source.
Methods
Alluvial sampling and analysis
The alluvial samples consist of black sand concentrates collected from stream beds and pits in the river terraces (Tables 1 and 2, Fig. 1). Gravel from the beds of the Alako (sample A1) and Big Water (sample BW2) was panned by a team of local workers. At each site a volume of gravel corresponding to 30 ‘standard head pans’ was reduced to black sand. The head pan is a shallow wooden dish ~70 cm in diameter that the local workers use for panning (and for carrying goods on the head – hence the name). The volume of a head pan was measured by filling it with water and weighing. The result indicated a volume of 11.68 litres although that represents the pan filled to the brim whereas a pan filled with gravel was often piled above the brim. For the purposes of estimation of a grade in the source material, a pan volume of 12 litres has been used. During panning of the streams rocks and boulders were avoided so the grade calculated in this way is an overestimate of a grade that would be achieved by mechanical extraction. The black sand from the rivers was split using a riffle splitter and a split assayed for Pt, Pd and Au by Lakefield Research in Canada.
Table 1. Panning of river gravels using a ‘headpan’.
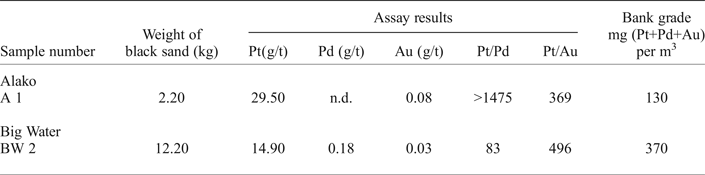
n.d. – less than detection limit (0.02 g/t).
The bank grade is an estimate of the in situ grade calculated using a ‘headpan’ volume of 12 litres and a factor of 1.38 to allow for the difference between the loose gravel in the pan and the undisturbed gravel. It is an overestimate as boulders were avoided when the gravel was collected.
Table 2. Results from pitting in the Big Water river terraces.
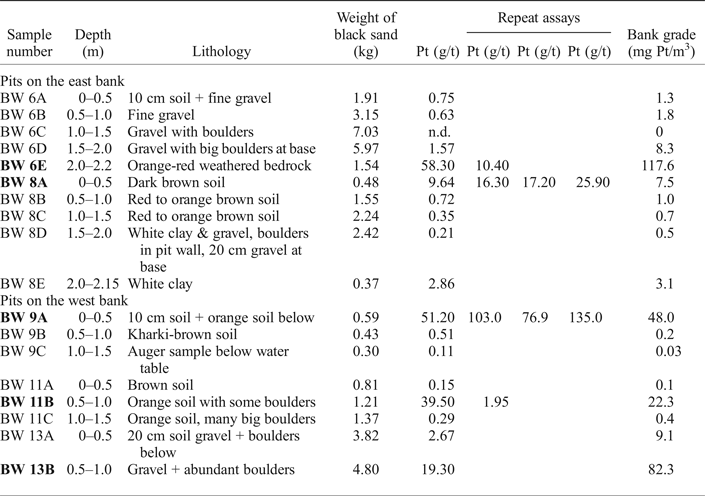
The samples studied are shown in bold type.
The river terraces either side of Big Water were examined by pitting. Square pits, 1.5 m on each side, were dug and all of the material panned (Pits BW6 – BW13, only pits reporting significant Pt values are discussed here). The depth of the pits was variable and constrained by reaching bedrock or rapid ingress of water through gravel below the water table. A ‘4 inch’ (100 mm diameter input) pump only allowed limited progress below the water table and an auger was used for deeper samples. Each interval of 0.5 m in depth in the pit was treated as a separate sample and panned to black sand. Each sample was dried on site and subsequently subsampled using a riffle splitter before assay only for Pt by Lakefield Research of Canada. High Pt assay results were checked by repeat assays.
Recovery and study of the PGM
The black sand concentrates (60–500 g of each sample) were panned again in the laboratory to recover the densest fraction and the PGM nuggets found during the process were hand picked. The remaining lightest fraction was processed with a Frantz LB-1 Magnetic Barrier Separator fitted with a Low Field Control Unit, which allowed a satisfactory separation of the most ferromagnetic and diamagnetic fractions. The majority of the alluvial nuggets were hand picked from the dense and the less magnetic fractions using a stereoscopic microscope. All the PGM recovered from the samples were mounted separately on stubs for examination of their morphology and, subsequently, in polished blocks for analysis using a Zeiss SMT S360 analytical scanning electron microscope (SEM) with an Inca EDX system at Cardiff University under the same analytical conditions described by Bowles et al. (Reference Bowles, Suárez, Prichard and Fisher2017). The remainder of the densest fraction of each sample was also examined by SEM to search for grains overlooked during hand picking.
Assay results and their relationship to drainage
Assays of the samples described here are shown in Table 1 (panning in the rivers) and Table 2 (pitting the river terraces). Repeated assay values show a significant variation due to the ‘nugget effect’. The assay values are reported as a proportion of the black sand and they have been converted to ‘bank grade’ to estimate the proportion of Pt in the sediment. The results all relate to small volumes of gravel and do not suggest an economic potential.
Pitting in the level river terrace of the east bank of the Big Water (pits BW6 and BW8) revealed gravel and lateritized soils to a depth of ~2 m overlying weathered bedrock. On the west bank soil overlies gravel in pit BW13. Soil and weathered bedrock are found between the patches of gravel and the boulders in pit BW11. These pits are at the foot of a steep hillside below the ridge on which the PGM-bearing horizons have been reported (Bowles, Reference Bowles2000b; Bowles et al., Reference Bowles, Prichard, Suárez and Fisher2013) and there has clearly been some slumping.
Platinum concentrations appear in the upper 0.5 m of sediment (up to 135 g/t Pt in black sand), at the bottom of some pits in weathered bedrock (up to 58.3 g/t Pt in black sand) and in intermediate horizons (up to 39.5 g/t in black sand) with no consistent pattern (Table 2). As a Pt concentration in the sediment, the highest values (up to 117.6 mg Pt/m3) occur close to bedrock but the near surface sediment can contain up to 48 mg Pt/m3. The Pt assays from the gravel in the Alako and the Big Water are similar and in the same range as the Big Water terraces (Table 1). The Pd and Au assay results are low resulting in very high Pt/Pd and Pt/Au ratios.
The Alako and the Big Water drain entirely different sequences of the layered rocks yet the alluvial PGM are similar. The Alako runs for 2 km along the west side of Mateki Ridge in a small, well defined watershed that does not include known PGM-bearing horizons. Drilling in this area (Barber, Reference Barber1962) was not followed up. There is no evidence of river capture or change of course that could have brought alluvial PGM from elsewhere. The west slope of Mateki Ridge above the Alako is the dip slope of the layers above the PGM-bearing horizons B, C and D that outcrop on the east slope of Mateki ridge. There are no tributaries or dry valleys on the west side of the ridge indicating sufficient erosion to expose the PGE-enriched layers. Therefore, simple erosion of the PGE-enriched layers should only release PGM into the Big Water and cannot explain the occurrence of PGM in the Alako.
Big Water rises 5 km above the sampling points (Fig. 1) and flows along the eastern side of Mateki Ridge with several dry valleys cutting into the ridge. The main stream comes from the east, crossing the strike of the layered rocks including the PGM-bearing Horizon M which has an As-, Sb-, PGE-bearing mineralogy (Bowles et al., Reference Bowles, Prichard, Suárez and Fisher2013). There could be some PGM input from Horizon M at the sampling sites but the nuggets do not show significant mineralogical differences between west bank, stream bed and east bank (Table 3) and there is no sign of As- or Sb-bearing phases. It seems likely that the majority of the PGM in Big Water are derived from the PGE-bearing horizons that follow Mateki Ridge.
Table 3. Alluvial PGM in the Big Water and Alako.
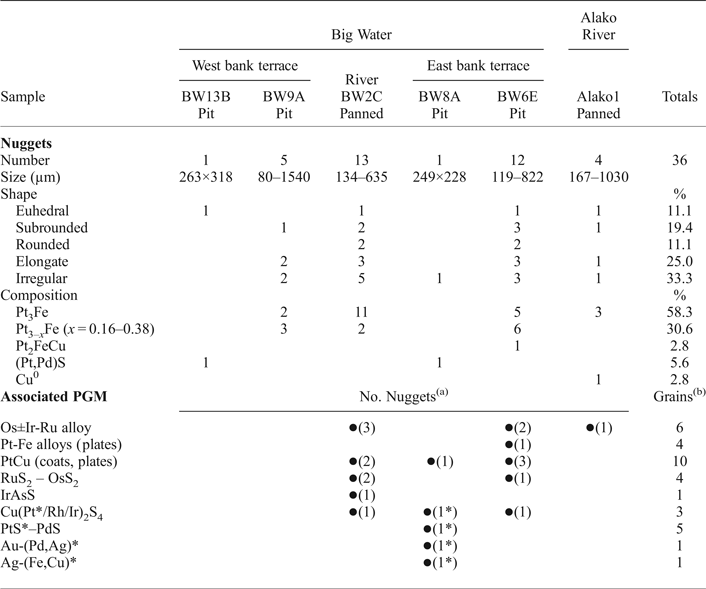
(a) Number of nuggets on which these associated PGM and native metals were found.
(b) Total number of grains located (approx.).
* Attached to ilmenite grains.
Mineralogy of the black sands
The black sands are mainly composed of magnetite, ilmenite and hematite grains about 200 to 600 µm across. Titanomagnetite sometimes displays oxidation textures with ilmenite lamellae, but generally magnetite is replaced by hematite and striking martitization textures are frequently observed. In other cases magnetite shows the shrinkage cracks that are typically caused by maghemitization. Ilmenite grains occasionally show hematite exsolution and less frequently the hematite is replaced by rutile. Some ilmenite grains enclose elongate Al-spinels near their rims. Ilmenite has generally been replaced by secondary Ti-bearing oxides at the edges and along fissures or is altered to Al-bearing maghemite at the edges. Accessory phases are rutile, zircon, garnet and baddeleyite with rare galena and sphalerite. There is a lighter fraction of rounded quartz grains with rare relicts of feldspar, pyroxene and mica. Goethite and gibbsite are present as rounded aggregates enclosing minute fragments of the resistate minerals.
PGM nuggets
Thirty-six PGM nuggets have been recovered from four pit samples from Big Water and from the gravels panned from the Big Water and the Alako (Table 3). The majority (89%) are brass coloured Pt-Fe alloys occasionally forming composite grains with minor hongshiite (PtCu). There is also one nugget of tulameenite (Pt2FeCu) and two nuggets of PGE-sulfides belonging to the cooperite–braggite–vysotskite series (PtS–PdS). One nugget of native Cu has been recovered. The nuggets are typically between 200 and 800 µm across and the largest, from the panned stream concentrates, are up to 1.5 mm × 1.4 mm in size.
The shape of the nuggets is variable as shown in Figs 2 and 3. Four euhedral nuggets were located (Fig. 2a–c). The nugget shown in Fig. 2b is very clearly a cube with well preserved corners that have not suffered abrasion. The nugget in Fig. 2a has (100) faces and five of them can be distinguished in the image. The region between the (100) faces has been abraded but there appear to be the remnants of (111) faces present. Subrounded to rounded shapes (n = 11 grains; Fig. 2d–j) or elongate shapes (n = 9; Fig. 2k–o) are more common. Some nuggets occur as irregular grains often with multiple isolated planar surfaces (n = 12; Fig. 2p–x).
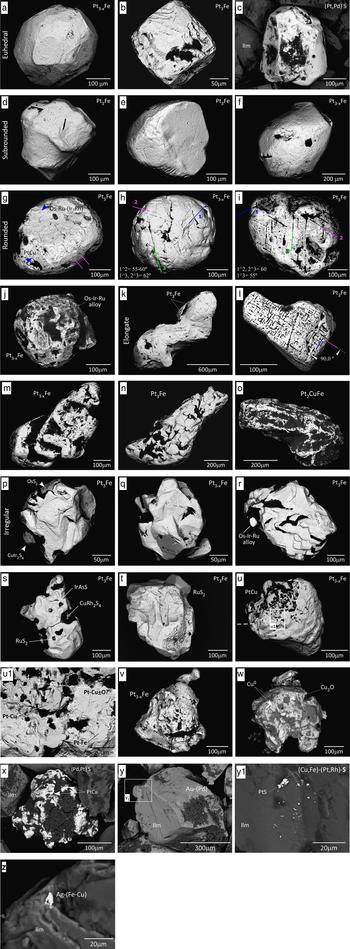
Fig. 2. Scanning electron microscope images of alluvial PGM nuggets from the Alako and Big Water, York area, Freetown. (a) Abraded Pt3–xFe with remnants of (111) faces; (b–c) euhedral isoferroplatinum (Pt3Fe) and Pd-bearing cooperite (PtS) nuggets; (d–e) subhedral Pt3Fe with pits and furrows on surface, (f) a more rounded Pt3–xFe; (g) subrounded Pt3Fe with Os-Ru-Ir and hongshiite (PtCu) plates disposed parallel to the surface; (h–i) rounded Pt3–xFe and Pt3Fe nuggets showing three sets of surface linear features; (j) rusty Pt3–xFe bearing an irregular plate of Os-Ir-Ru alloy; (k) elongate Pt3Fe with fine striations covering the whole nugget; (l) another elongate Pt3Fe with two sets of surface structures at right angles; (m) elongate and irregular single nugget of Pt3–xFe; (n) elongate Pt3Fe nugget with a heterogeneous surface showing aggregates of more euhedral to irregular crystals of Pt-Fe alloys; (o) tulameenite (Pt2FeCu); (p–s) irregular Pt3Fe and Pt3–xFe nuggets with large PGM attached including erlichmanite (OsS2), cuproiridsite (CuIr2S4), Os-Ir-Ru alloys, irarsite (IrAsS), osmian laurite (RuS2) and cuprorhodsite (CuRh2S4); (t) irregular Pt3Fe nugget with several regular shaped depressions on the surface, a large pyritohedron of laurite is attached; (u) irregular composite nugget of Pt3–xFe and PtCu displaying a lobate and irregular contact between them, where (u 1) Pt-Cu-bearing oxides and platelets of Pt-Fe and Pt-Cu lie parallel to the surface of the nugget; (v) irregular and rather porous Pt3–xFe nugget; (w) nugget of native Cu with cuprite (Cu2O) on the surface; (x) irregular (Pd,Pt)S bearing PtCu platelets on surface along with large masses of Fe-Al-oxides; (y–z) two examples of ilmenite (Ilm) that have small clusters of PGM (cooperite and probable malanite‒CuPt2S4), Au and Ag grains, all of them <5 µm in size attached to their surface.
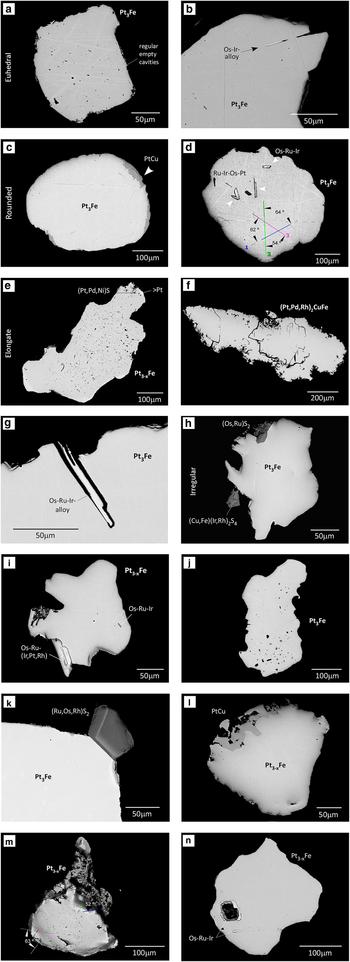
Fig. 3. Scanning electron microscope images of the polished sections of some of the alluvial PGM shown in Fig. 2. (a) Euhedral Pt3Fe nugget (Fig. 2b) showing ragged edges and regular voids; (b) a well-developed Os-Ir lamella extending to and eroded at the edge of a Pt3Fe nugget; (c) PtCu replacing Pt3Fe at the edge (Fig. 2g); (d) cluster of Os-Ru-Ir alloy inclusions (bright) with Ru-Ir-Os alloy inclusions (dark) in a Pt3Fe nugget (Fig. 2i). The inclusions follow preferred orientations which are intersecting at 55‒60°; (e) elongate Pt3–xFe (Fig. 2m) full of base-metal bearing Rh-S inclusions. One of the edges (top right) encloses PGE-sulfides (cooperite and braggite); (f) a nugget of tulameenite (Fig. 2o) showing porous and corroded edges in section; (g) thin crystal of an Os-Ru-Ir alloy that may correspond to a broken or corroded Os platelet extending to the edge of the host Pt3Fe; (h) erlichmanite (OsS2) and cuproiridsite (CuIr2S4) crystals at the edges of Pt3Fe (Fig. 2p); (i) a large platelet of Os-Ru-Ir alloy attached to Pt3–xFe (Fig. 2q); (j) irregular elongate Pt3Fe grain (Fig. 2s) full of varied polygonal crystals of PGM; (k) Pt3Fe with the large crystal of laurite shown in Fig. 2t; (l) section of a composite Pt3–xFe-PtCu nugget (Fig. 2u, u 1) showing the irregular contact between the phases suggesting replacement by the PtCu; (m) porous Pt3–xFe nugget (Fig. 2v) hosting various Pt-Pd-alloys; (n) irregular Pt3–xFe nugget with a large crystal and lamellae of Or-Ru-Ir alloys.
Several grains show pits and etched sub-parallel furrows on the surface (Fig. 2e, k). There are also surface linear features that either intersect at ~60° or 90° indicating a crystallographic control (Fig. 2h, i, l). The pattern of intersecting features maintains the same orientation across the whole surface of the nuggets, suggesting that the nuggets are probably single crystals.
The nuggets are often partially coated by Fe- and Al-oxides or altered silicates commonly on irregular grains that are also often covered by smaller PGM grains (Fig. 2j, p, r, s, u, x).
There are many smaller PGM associated with the Pt nuggets (Table 3); they occur singly or as clusters on the nugget surface or within the nugget. They are also seen as free grains. Those at the nugget surface are generally larger (0.1 to 0.4 × the diameter of the nugget) whilst those within the nuggets are micrometre sized but exhibit the same mineral assemblage. The surface PGM form irregular platelets up to 80 µm across consisting of Os ± Ir-Ru-(Rh) alloys (osmium, according to the nomenclature in Harris and Cabri, Reference Harris and Cabri1991) as shown in Figs 2g, j, r, 3i. There are smaller irregular platelets up to 20 µm across of Pt-Fe and Pt-Cu alloys (Fig. 2u, u 1), non water worn euhedral pyritohedra of Os-bearing laurite (~130 µm in diameter, Figs 2t, 3k), and crystals of laurite (RuS2), erlichmanite ((Os,Ru)S2), irarsite ((Ir,Rh)AsS), cuproiridsite and cuprorhodsite ((Cu,Fe) (Ir/Pt,Rh)2 S4) that are ~50 µm across (Figs 2p, s; 3h).
The osmium platelets can be set at a steep angle or lie parallel to the surface of the Pt-Fe host (Figs 2j, r; 3b, g, i; also Bowles, Reference Bowles1981, fig. 1; Bowles, Reference Bowles1995, figs 8, 9, 10). The laurite–erlichmanite crystals project from the surface of the Pt-Fe alloy host and are frequently euhedral (Figs 2p, t; 3h, k; also Bowles, Reference Bowles1981, fig. 2; Bowles, Reference Bowles1995, figs 1, 7; Cabri, Reference Cabri1981 figs 7.9A–C and 7.26A). Cuproiridsite and cuprorhodsite (Figs 2p, s; 3h) may also show this relationship. In polished section the contact between the host and the associated mineral is a straight line (Fig. 3g, h, i). Examples (Fig. 3k and Bowles Reference Bowles1995, fig. 10) demonstrate that this contact is between planar crystal faces of two crystals that grew at the same time. In some cases the host appears to have grown outwards to surround the associated mineral (Fig. 2g, j and Bowles, Reference Bowles1995, fig. 10). A totally different relationship is seen (Fig. 3c, l) where hongshiite is situated along the rim of a Pt-Fe alloy. Here the contact between the two minerals is curved or lobate and within the boundary of the whole grain. These textures suggest replacement of Pt-Fe by PtCu. A cartoon diagram to illustrate these relationships is shown in Fig. 4.

Fig. 4. Cartoon to assemble various textures of the nuggets in one diagram. The host Pt-Fe alloy (grey) may have a sculpted or euhedral outline. Laurite-erlichmanite (green) and Os-rich alloys (blue) exterior to the Pt-Fe alloy are normally euhedral. Osmium-rich alloys within the nugget are often euhedral. Alteration of the Pt-Fe alloy to hongshiite (PtCu, in orange) shows cuspate textures. This sketch is drawn from examples shown in Figs 2 and 3, and from Bowles (Reference Bowles1981, Reference Bowles1995). Inclusions from the present work (Fig. 3g, k) show broken surfaces where they protrude from the host. Observations from previous work of the probable original form of these crystals are drawn with lighter tones of blue and green.
PGM on the surface of ilmenite grains
In a separate association, very small (5 µm) PGM and native Au and Ag grains have been found attached to ilmenite grains recovered from the pits (Table 3). In one case a cluster of cooperite and malanite grains occurs on the surface of an ilmenite grain (Fig. 2y, y 1) and euhedral grains of Pd-Ag-bearing gold are also present. A grain approximating to native silver was also observed on the surface of another ilmenite grain (Fig. 2z).
Composition of the PGM nuggets
Pt-Fe alloys
The 32 Pt-Fe nuggets recovered have a composition ranging from Pt3Fe to Pt2.6Fe1.4. The majority of the nuggets (70%) have a composition close to isoferroplatinum with a general formula of Pt3–xFe with x = 0–0.38. Compositions are plotted in Fig. 5 and representative analyses are presented in Table 4. There is substitution of Pt by other PGE (mainly Pd with Rh, and rarely Ir and Ru) and Fe by Cu and, to a lesser extent, Ni. Palladium and Rh have been recorded in ~20 of the Pt-Fe nuggets recovered and the concentration of either is usually <8 at.% but rarely nuggets can contain as much as 25 at.% Pd and these can contain Pd-bearing inclusions.

Fig. 5. The compositions of the eluvial and alluvial PGM from the Mateki area plotted on a triangular (Pt+Pd)–Cu–(Fe+Ni) diagram. Some analyses of the small eluvial PGM are qualitative. Additional data from the literature is included for comparison.
Table 4. Representative compositions of the Pt-nuggets and large associated PGM from energy-dispersive spectrometry.
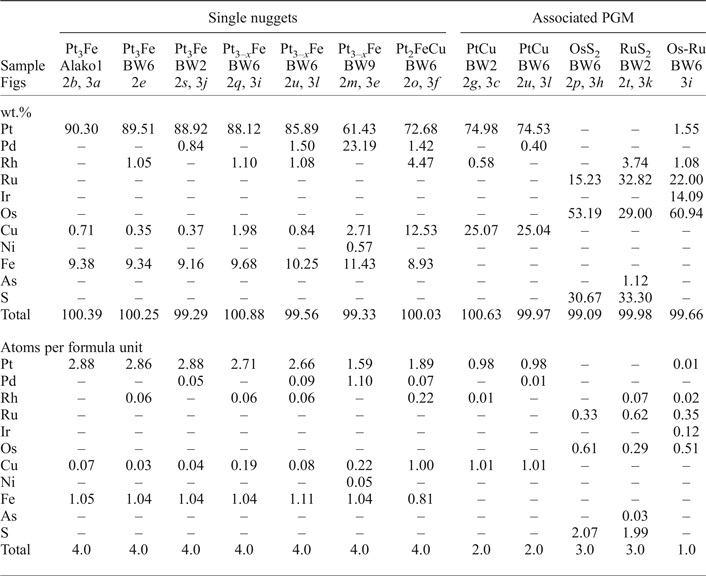
– not detected.
Hongshiite (PtCu)
Hongshiite (Fig. 2g, u, u 1) forms both irregular rims to six of the Pt-Fe alloys (Fig. 3c, l) and plates lying parallel to the nugget surface (Fig. 2u 1). The hongshiite has uniform Pt and Cu contents with occasional minor Pd (av. 1.8 at.%), Fe (1.8 at.%) and Rh (0.5 at.%) (Table 4).
Tulameenite
One elongate nugget of tulameenite was found (BW6, Table 3). It is 600 µm across and largely coated with patches of Al-Si-(Fe)-oxides (Fig. 2o). In section, the nugget appears slightly cracked and has very irregular and porous edges (Fig. 3f). The composition is consistent throughout ((Pt,Pd,Rh)2Cu1.25Fe0.75) having higher Cu than ideal tulameenite and uniform Pd and Rh contents totalling ≤4.0 at.% (Fig. 5; Table 4). Unlike the tulameenite from Guma Water (Bowles, Reference Bowles1981), no traces of Os and Ru were detected in this nugget.
Pt-Pd sulfides
Two nuggets belonging to the cooperite–braggite–vysotskite series were located during pitting beside Big Water (Table 3). One nugget is euhedral cooperite 300 µm in diameter (Fig. 2c) containing an average of 42 at.% Pt and 10 at.% Pd along with low Ni and Cu contents totalling 1.5 at.%. The other nugget is an irregular grain of braggite–vysotskite 250 µm in diameter (Fig. 2x) that is partially coated by Al-oxides and has small plates of hongshiite 22 µm across on its surface. This nugget has an average of 34.2 at.% Pd, 14.3 at.% Pt and minor amounts of Fe (1.4 at.%), Ni (0.6 at.%) and Co (0.3 at.%).
Ru-Os sulfides
Members of the laurite (RuS2)–erlichmanite (OsS2) series occur attached to Pt3Fe nuggets from Big Water and none were found as discrete grains (Table 4). One example is an irregular grain of erlichmanite (Fig. 2p) whilst the other grains are laurite with variable Os content, the largest being a 50 µm diameter pyritohedron (Fig. 2t, 3k) with Os (av. 11.9 at.%), Rh (2.3 at.%) and minor As (0.18 at.%).
Rh, Ir, Pt base metal sulfides
Two grains of the cuprorhodsite–cuproiridsite–malanite series were found both attached to Pt3Fe nuggets from Big Water (Table 3). One grain is about 40 µm in diameter (Figs 2p, 3h) with a composition close to the cuproiridsite (Cu,Fe) (Ir, Rh)2 S4 and ~20 at.% Ir and 8 at.% Rh. The other grain is 30µm across (Fig. 2s) and has a composition closer to cuprorhodsite (Cu,Fe) (Rh, Pt, Ir)2 S4, with about 12 at.% Rh, 10.5 at.% Pt and 7.3 at.% Ir. A platinum-rich thiospinel resembling malanite (Cu,Fe)(Pt,Rh)2S4 containing Pt (av. 17.2 at.%) and Rh (10.3 at.%) was found on an ilmenite surface (Fig. 2y, y 1).
Osmium alloys
Alloys of Os-Ru±Ir form part of many of the composite nuggets found in the Big Water and the Alako (Table 3). They occur as thin platelets lying either parallel to the host surface (Figs 2g, j, 3i), or at a steep angle to the surface (Fig. 2q, r). Some of the steeply set platelets are partially contained within the nugget and extend outwards from the nugget surface where damage can occur (Fig. 3g). Inclusions of Os-Ru±Ir alloys are seen in cross sections and in Big Water both Os-Ru-Ir alloy inclusions and Ru-Ir-(Os-Pt, Rh) alloys occur in the same host (Fig. 3d).
The osmium-rich alloys contain variable Ru (0–37 at.%) and Ir (6–21 at.%), and minor Rh, Pt and As totalling up to 4.8 at.%. About 70% of the Os-alloys analysed contain higher Ru than Ir regardless of the relation to their hosts. Similar variable compositions were reported for the hexagonal platelets of Os on the surface of the Guma Water nuggets (Bowles, Reference Bowles1981).
Discussion
Contrasting explanations have been offered for the occurrence of alluvial PGM nuggets: (1) Nuggets derived from the erosion of igneous rocks can travel with soil that is washed down slope and into rivers to be trapped in patches of gravel that form a natural riffle. The PGM are unaltered, except for surface abrasion (Cabri and Harris, Reference Cabri and Harris1975; Cabri and Laflamme, Reference Cabri and Laflamme1997; Cabri, Reference Cabri1981; Cabri et al., Reference Cabri, Criddle, Laflamme, Bearne and Harris1981, Reference Cabri, Harris and Weiser1996; Malitch and Thalhammer, Reference Malitch and Thalhammer2002; Weiser Reference Weiser and Cabri2002). (2) Weathering of the rocks, aided by organic acids in the soil, breaks down the primary PGM releasing the PGE which move through the soil in solution. Soil close to a river is a location where the contrast between the Eh and pH of the soil and the river is highest. A large change from acidic to neutral conditions accompanied by a reduction in Eh can cause precipitation of the PGE allowing the growth of nuggets. This growth may be aided by bacterial activity (Reith et al., Reference Reith, Zammit, Shar, Etschmann, Bottrill, Southam, Ta, Kilburn, Oberthür, Bail and Brugger2016). Areas of tropical weathering with high rainfall and abundant organic material seem to be where neoformation can occur. The distinctive mineralogy (mineral assemblage, size, composition and surface features) of alluvial PGM led to proposals of a neoform origin for such PGM. This view has been taken during studies in Minas Gerais, Brazil (Hussak, Reference Hussak1906; Cassedanne and Alves, Reference Cassedanne and Alves1992; Cabral et al., Reference Cabral, Beaudoin, Choquette, Lehmann and Polônia2007), Yubdo, Ethiopia (Ottemann and Augustithis, Reference Ottemann and Augustithis1967; Augustithis, Reference Augustithis, Laverov and Distler1998), the Witwatersrand reefs and the Bushveld Intrusion, South Africa (Cousins, Reference Cousins1973; Cousins and Kinloch, Reference Cousins and Kinloch1976), and for the Freetown Peninsula in Sierra Leone (Bowles, Reference Bowles1981, Reference Bowles1986, Reference Bowles, Prichard, Potts, Bowles and Cribb1988, Reference Bowles1995).
The alteration of primary PGM may involve several stages during both serpentinization and weathering (Prichard et al., Reference Prichard, Ixer, Lord, Maynard and Williams1994; Wang et al., Reference Wang, Prichard, Zhou and Fisher2008; Aiglsperger et al., Reference Aiglsperger, Proenza, Galí, Rius, Longo and Domènech2017) leading to the formation of oxidized PGM. The Great Dyke, Zimbabwe (Locmelis et al., Reference Locmelis, Melcher and Oberthür2010) and the Aguablanca deposit, Spain (Suárez et al., Reference Suárez, Prichard, Velasco, Fisher and McDonald2010) offer notable examples. The oxidized PGM are then lost during the transition from PGM in soils or laterites close to the source forming a Pt-alloy dominated placer PGM as demonstrated by Oberthür and Melcher (Reference Oberthür, Melcher and Mungall2005) for the oxidized Main Sulfide Zone and placer PGM in the Great Dyke. The study of PGM in fresh rocks and closely associated saprolite in the Freetown area (Bowles et al., Reference Bowles, Suárez, Prichard and Fisher2017) has shown that the primary PGM have been destroyed by weathering. This has released the PGE which have moved separately through the saprolite.
The processes postulated for PGM neoformation include: (1) long term weathering in an area that has, or has had, a warm climate and high rainfall; (2) destruction of the primary PGM and transport of the PGE in solution; (3) organic compounds, such as humic or fulvic acids that are abundant in tropical rain forest soils appear likely to be involved in solution and transport of the PGE; (4) differential movement of the PGE in solution; (5) concentration of the least mobile PGE in soils close to the rivers, the more mobile PGE (especially Pd) being carried away in solution by the rivers; (6) accretion of the remaining PGE to form a new PGM assemblage; possibly where there is a change in Eh and pH conditions favourable for deposition; and (7) bacterial action may assist, or be responsible for, the PGM growth.
The main evidence that PGM grow in situ as neoforms is that they comprise a different mineral assemblage from the PGM in the source rocks. The differences are: (1) PGE arsenides, tellurides and sulfides present in the host rocks become less abundant or disappear completely from the alluvial suite; Pt-Fe alloys including tulameenite, Os-Ir alloys and laurite–erlichmanite become more abundant; (2) oxidized PGM appear in weathered or oxidized rocks. Cloudy, porous or filamentous altered PGM may also be present; (3) there is a loss of the more soluble Pd which is present in the host rocks, but much less abundant in an alluvial mineral suite; (4) there is a considerable difference in size (typically three orders of magnitude) between micrometre-sized PGM in the host rocks and millimetre-sized PGM in the alluvial suite; (5) some PGM in an alluvial suite show delicate crystal features that would not survive mechanical transport, these features include dendritic growths, perfect crystal faces and perfect edges and corners between crystal faces. The corners are the most susceptible to mechanical damage and the presence of undamaged corners provides convincing evidence for the absence of abrasion. The dendritic PGM are not known to occur in the host rocks. (6) There is over plating of mineral faces, colloform, cyclic and fibrous textures not characteristic of PGM in the host rocks.
Examples of alluvial PGM showing these textures have been described by Oberthür et al. (Reference Oberthür, Weiser and Gast2003, Reference Oberthür, Weiser, Melcher, Gast and Wöhrl2013); Zaccarini et al. (Reference Zaccarini, Pushkarev, Garuti, Krause, Dvornik, Stanley and Bindi2013); Bowles (Reference Bowles1981,Reference Bowles1986,Reference Bowles1995); Cabral et al. (Reference Cabral, Beaudoin, Choquette, Lehmann and Polônia2007, Reference Cabral, Galbiatti, Kwitko-Ribeiro and Lehmann2008) and Burgath (Reference Burgath, Prichard, Potts, Bowles and Cribb1988).
Some workers have pointed out that the question of the smaller grain size in the host rocks compared to the grain size of the alluvial PGM could be due to sampling. For a given grade, small grains are more numerous and more likely to be encountered by chance in a polished surface than large grains. It has been suggested, therefore, that the large grains are present in the rocks but are not detected. Indeed Oberthür et al. (Reference Oberthür, Weiser and Gast2003) have shown that larger PGM are difficult to locate but do occur in the Main Sulfide Zone (MSZ) of the Great Dyke, Zimbabwe. Large Pt-Fe aggregates do occur in the rocks of the Nizhni Tagil complex and the Vetnaya–Vyvenka Belt in Russia, and the Alto Condoto complex in Colombia (Duparc and Tikonowitch, Reference Duparc and Tikonowitch1920; Cabri and Genkin, Reference Cabri and Genkin1991; Salinas et al., Reference Salinas, Muñoz, Burgath and Tistl1992; Tistl, Reference Tistl1994; Zaitsev et al., Reference Zaitsev, Loginov, Melkomukov, Vorogushin, Vildanova, Litvinov, Patoka and Sidorov1998). Where such large nuggets occur in the host rocks they can unquestionably be released by weathering. It has also been suggested that perfectly crystallized or delicate alluvial grains could have been transported within a protecting rock matrix from which they have recently been released (e.g. Hagen et al., Reference Hagen, Weiser and Than1990, Weiser and Bachmann, Reference Weiser and Bachmann1999; Cabri et al., Reference Cabri, Harris and Weiser1996). Inclusions of PGM and other minerals found in the alluvial nuggets such as chromite, silicates, sulfides or composite glass-silicate inclusions (e.g. Nixon et al., Reference Nixon, Cabri and Laflamme1990; Johan et al., Reference Johan, Ohnenstetter, Fischer and Amossé1990; Augé and Legendre, Reference Augé and Legendre1992) are taken to indicate a primary origin for the inclusions and, therefore, the nuggets.
Although growth of placer PGM appears to be favoured by a warm wet climate and a long period of weathering, the dissolution of igneous PGM, their transport in solution and growth of the PGM in the placer could be relatively rapid. Vehicle catalytic converters have been in use for <40 years but particulate Pt and Pd catalytic converter fragments occur in road dust (Prichard and Fisher, Reference Prichard and Fisher2012) and are concentrated in nearby acidic stream sediments (Prichard et al., Reference Prichard, Jackson and Sampson2009). In the water courses the PGE can become bioavailable after reaction with artificial additives (phosphates from fertilisers, NaCl from road de-icing) or natural organic compounds (especially citrates and fulvic acids, Pawlak et al., Reference Pawlak, Łodyga-Chruścińska and Chrustowicz2014). Bowles et al. (Reference Bowles, Giże, Vaughan and Norris1994b, Reference Bowles, Giże, Vaughan and Norris1995) examined the relevance of the organic acids present in soils to the solution, transport and deposition of the PGE. Experiments showed that organic acids can take Pt into solution from fine-grained PtS in as little as 393 days. The laboratory experiments on bacterial growth of PGM by Reith et al. (Reference Reith, Zammit, Shar, Etschmann, Bottrill, Southam, Ta, Kilburn, Oberthür, Bail and Brugger2016) were conducted over 112 days. This is particularly significant because purely inorganic growth at low temperatures is likely to be very slow. The evidence indicates that the dissolution of igneous PGM, their transport in solution and growth of the PGM in the placer could be rapid.
Recent studies have very reasonably shown that alluvial PGM assemblages contain components derived by both mechanical means and by PGM alteration (e.g. Goodnews Bay, Alaska, Mardock and Barker, Reference Mardock and Barker1991; Eastern Bushveld, South Africa, Melcher et al., Reference Melcher, Oberthür and Lodziak2005; Great Dyke, Zimbabwe, Oberthür et al., Reference Oberthür, Weiser, Gast, Schoenberg and Davis2002, Reference Oberthür, Weiser and Gast2003, Reference Oberthür, Weiser, Melcher, Gast and Wöhrl2013; Uktus, Central Urals, Zaccarini et al., Reference Zaccarini, Pushkarev, Garuti, Krause, Dvornik, Stanley and Bindi2013).
Evolution of the PGM in the York area, Freetown
Lithogeochemical and geomagnetic surveys associated with the present study have not encountered any anomalies in the rocks or soils indicating PGM-bearing horizons within the catchment areas of the streams examined in addition to those already reported (Bowles, Reference Bowles2000b; Bowles et al., Reference Bowles, Prichard, Suárez and Fisher2013). Repeated assays of samples, of splits from those samples, and of repeated samples from the same outcrops show consistent results. This shows that the Freetown primary PGM grain size in the rocks is clearly small with no indication in the rocks of a ‘nugget effect’.
The PGM assemblage shows a development from the source rock through the saprolite to the alluvial deposits (Figs. 6, 7) with the source rocks dominated by Pt-Fe alloys (Pt3Fe, PtFe) and cooperite (PtS) with minor Pt-Rh-Ir base metal sulfides, Pd-Cu minerals, laurite, tulameenite, Pd-Pt alloys and scarce Pt-arsenides and Pt-antimonides. The weathered rocks contain oxidized PGM (Bowles et al., Reference Bowles, Prichard, Suárez and Fisher2013, Reference Bowles, Suárez, Prichard and Fisher2017) which marks the first stage of weathering. In more weathered rocks the PGM assemblage is dominated by Pt3Fe–PtFe and Pt2FeCu–Pt(Fe,Cu) compositions and PtS. The alloys show a wider range of compositions than those in the source rocks. Sulfides rich in Pt-Rh and osmian laurite remain as accessory minerals, but Pd-PGM are absent suggesting that both Pd and Cu are dispersed. Within the saprolite only a few Pt3–xFe–PtFe3 alloys seem to survive (Fig. 6). Weathering of the PGM would enable PGE-bearing solutions to drain towards both Big Water and the Alako resulting in a similar alluvial PGM assemblage in both rivers (Figs. 6, 7).
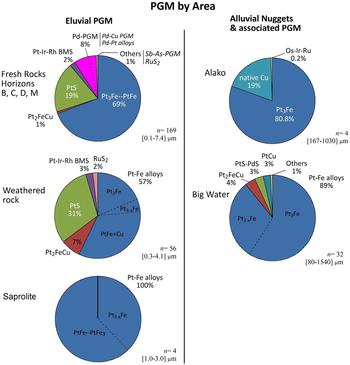
Fig. 6. Pie charts showing the changes in the Freetown PGM from the fresh source rocks to the laterite (Bowles et al., Reference Bowles, Prichard, Suárez and Fisher2013, Reference Bowles, Suárez, Prichard and Fisher2017) and to the alluvial deposits in the Alako and Big Water streams. BMS = base metal sulfides, n = number of PGM grains and their size range.
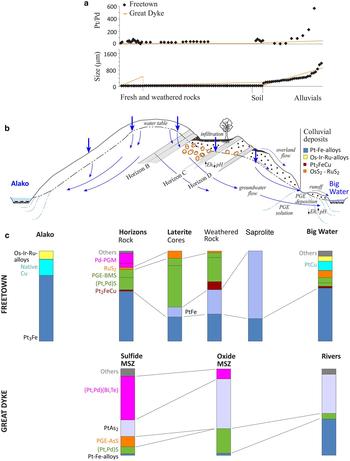
Fig. 7. The mineralogical evolution in the PGM assemblage from rock through the weathering profile to alluvial deposits. (a) The variation in Pt/Pd ratio and grain size for the Freetown examples described here and comparable PGM from the Great Dyke, Zimbabwe (Reference Oberthür, Weiser and GastOberthür et al. 2003). (b) The possible processes involved in the alluvial concentration of PGM in the rivers. (c) Freetown mineral assemblage from the PGE-bearing primary rocks through weathered rocks, soils to alluvial deposits in the rivers. The evolution of the PGM from the Great Dyke, Zimbabwe (Reference Oberthür, Weiser, Melcher, Gast and WöhrlOberthür et al. 2013) is shown for comparison. BMS = base metal sulfides, MSZ = main sulfide zone. PGM shown by number of occurrences found.
The alluvial PGM nuggets are predominantly Pt-Fe alloys mainly of Pt3Fe; disordered Pt3–xFe is also present with minor tulameenite and there is an infrequent occurrence of native copper. The PtFe in the weathered rocks does not appear in the alluvium. The Pt-Fe nuggets are often partly replaced by hongshiite (PtCu). This may be part of a relationship between hongshiite and the Pt-Fe alloys that has been recorded from Brazil where hongshiite has been further altered to native platinum (Kwitko et al., Reference Kwitko, Cabral, Lehmann, Laflamme, Cabri, Criddle and Galbiatti2002; Cabral et al., Reference Cabral, Galbiatti, Kwitko-Ribeiro and Lehmann2008). Members of the cooperite–vysotskite series (PtS–PdS), laurite and erlichmannite also appear as large, single grains in the alluvium. Less abundant phases (<16% of the total number of PGM located) are the PGM and native metal grains that occur enclosed in the Pt-nuggets or more rarely, on the surface of ilmenite (Fig. 2y, z; Fig. 3). A comparable story is told by the weathered rocks of the Great Dyke, Zimbabwe where PGE bismuthotellurides and sulfarsenides in the MSZ in fresh rock underground and the oxidized PGM in the oxidized ores are all missing in the alluvium. Alloys of Pt-Fe that form a minor proportion of the MSZ and the oxidized MSZ are much more abundant in the alluvial nuggets together with sperrylite, cooperite, braggite, laurite, rare Pd-Sb-As compounds, and Os-Ir-Ru alloys (Oberthür et al., Reference Oberthür, Weiser and Gast2003, Reference Oberthür, Weiser, Melcher, Gast and Wöhrl2013).
The Freetown mineral assemblage reflects both Pt and Pt, Ir, Os and Ru enrichment down slope, as observed in placer PGM deposits worldwide (Weiser, Reference Weiser and Cabri2002). It is the loss of Pd that is the most notable especially in the alluvial deposits. In the panned samples, it is evident that the Pt/Pd ratio is highest in those samples that show the highest Pt. In samples rich in Pt where Pd was below detection the Pt/Pd ratios can be even higher (Table 1). These samples appear to be the most evolved and are found in natural traps in the stream bed where the course of the stream is level and the water is moving slowly.
Size
The alluvial nuggets in Big Water and Alako streams are in the same size range as the colluvial PGM in Guma Water (maximum nugget size 2 cm). There is a sharp increase in size from a maximum of 7 µm for the PGM in the rocks and the laterite to the placers examined in this study. The nuggets reported here have a maximum size of 1.5 mm but larger nuggets (up to 2 cm) have been reported by earlier workers (Pollet, Reference Pollett1931). For example, laurite is <1 µm across in the rock but occurs as 1 mm sized euhedral crystals downslope. The Os alloys, not found in the rocks, are typically 50 µm across in the alluvium with occasional examples up to 80 µm across. The three orders of magnitude difference between the PGM in the alluvium and the source rocks contributes to the contrast between the mineral assemblages.
In the Big Water small PGM (0.6–5 µm) have also been found during this study with grains of gold and silver forming clusters on ilmenite. Ilmenite-rich black sand concentrates from the artisanal workings were assayed to give 240 g/t Pt metal and the slimes, after titanium pigment preparation, gave about 400 g/t Pt (Pollet, Reference Pollett1931) which suggests that these small PGM may be quite common in the stream black sands. They are the same size as the PGM in the igneous rocks and include cooperite and malanite so they may be primary PGM but they may equally well represent the early stage of nugget growth.
Shape
The Big Water and Alako are rejuvenated streams with extensive stretches of water moving quickly among rocks, an environment where abrasion and rounding of nuggets would be expected. The presence of euhedral nuggets and nuggets with well-preserved crystal faces indicates either that their source is very close, or that they have grown in situ. Some nuggets display clear hexagonal and triangular-shaped depressions (Fig. 2i, p, q, t; Fig. 3b) suggesting a recent loss of attached euhedral grains. Other surface inclusions have survived abrasion, for example a large pyritohedron of laurite (Fig. 3k) and an Os platelet (Fig. 3i have been damaged). The irregular surface of some PtFe alloys also suggests some erosion has occurred as illustrated in Fig. 4.
Pt-Fe alloy compositions
Pt-Fe alloys with compositions approximating to Pt2Fe have been reported previously especially from placer deposits. Some examples are the Chocó region, Colombia (Cabri et al., Reference Cabri, Harris and Weiser1996); the Aikora River Area, Papua New Guinea (Weiser and Bachmann, Reference Weiser and Bachmann1999); the Great Dyke (Oberthür et al., Reference Oberthür, Weiser and Gast2003, Reference Oberthür, Weiser, Melcher, Gast and Wöhrl2013); British Columbia, Canada (Barkov et al., Reference Barkov, Fleet, Nixon and Levson2005); the Urals (Malitch and Thalhammer, Reference Malitch and Thalhammer2002; Evstigneeva, Reference Evstigneeva2009; Zaccarini et al., Reference Zaccarini, Pushkarev, Garuti, Krause, Dvornik, Stanley and Bindi2013) or Yubdo (Evstigneeva, Reference Evstigneeva2009). Malitch and Thalhammer (Reference Malitch and Thalhammer2002) and Evstigneeva (Reference Evstigneeva2009) discuss in detail whether a composition near Pt2Fe represents a new mineral phase, a fine intergrowth of PtFe and Pt3Fe (as suggested by Zhernovsky et al., Reference Zhernovsky, Mochalov and Rudashevsky1985) or a disordered structure that is stable under particular conditions. The majority of the grains examined using X-ray diffraction consisted of a disordered cubic phase but the studies also confirmed a micrometric intergrowth in two grains from the Nizhni Tagil placers.
It seems probable that the Freetown Pt-Fe alloy with a composition near to Pt2Fe is cubic and is similar to cubic tetraferroplatinum (Pt3Fe) but containing more Fe with some degree of disordering of the structure. It should probably be described as Pt3–xFe where x = 0.16–0.38 in the alluvial nuggets. There is a larger range of disordering in the weathered Pt-Fe alloy where x = 0.02–0.58 (Bowles et al., Reference Bowles, Suárez, Prichard and Fisher2017).
The existence of the disordered phases with a range of compositions is relevant to consideration of the genesis of these PGM. At high temperature, with greater mobility of the metals, there is a preference towards forming minerals with stoichiometric compositions having a restricted compositional range and an ordered structure. The inference that can be made from the presence of an almost continuous range of compositions, leading to the formation of disordered phases, is that formation occurred under conditions of restricted mobility as implied by a lower temperature.
The compositions of Pt-Fe alloys from similar localities are plotted in Fig. 5. Some of the Uralian nuggets described by Zaccarini et al. (Reference Zaccarini, Pushkarev, Garuti, Krause, Dvornik, Stanley and Bindi2013) have isoferroplatinum-type solid cores (Pt3–xFe) surrounded by porous PtFe. The PtFe in turn includes minute particles with a transitional, Pt3–x (Fe,Cu) composition (x ≤ 0.35) that contain a higher base-metal content than either the alluvial grains from Freetown or from the Great Dyke (Oberthür et al., Reference Oberthür, Weiser, Melcher, Gast and Wöhrl2013). A minority of grains examined in the Uktus complex and Great Dyke have Pt/BM ratios close to 1 that represent solid solutions of PtFe and Pt2FeCu. These all fall in the same field as the Pt-Fe-Cu alloys in the Freetown laterite (Fig. 5). In other areas such as Borneo and Madagascar (Burgath, Reference Burgath, Prichard, Potts, Bowles and Cribb1988; Augé and Legendre, Reference Augé and Legendre1992) the alloy compositions occupy the Pt-rich part of the system.
Conclusions
The suite of alluvial PGM identified in this study comprises mainly Pt3Fe to Pt3–xFe and a lesser proportion of tulameenite and PtS–PdS nuggets. Large crystals associated with the nuggets are Os-Ir-Ru alloys, laurite–erlichmanite and PGE base-metal sulfides (e.g. cuprorhodsite and cuproiridsite). The presence of tulameenite and native copper as individual nuggets and hongshiite alteration of the rims of Pt-Fe alloy is an aspect of the involvement of Cu in the development of the nuggets. A late generation of Pt-enriched isoferroplatinum is observed on a few nugget surfaces. Some alluvial PGM such as tulameenite or Pt-sulfides can contain higher Pd than their counterparts in the rocks. This denotes changes in the mineralogy and geochemistry between the primary and alluvial PGM in Freetown.
The significant points are:
Assemblage
There is a distinctive difference between the PGM assemblage in the fresh rocks and the alluvium. Cooperite, abundant in the fresh rocks, is less abundant in the streams whereas Pt-Fe alloys form a much larger proportion of the alluvial mineral suite. Hongshiite appears as an alteration to the alluvial Pt-Fe alloys and tulameenite is more abundant. Palladium minerals in the fresh rocks are not encountered in the alluvium.
Oxides
Oxidized PGM in the weathered rocks (Bowles et al., Reference Bowles, Prichard, Suárez and Fisher2013, Reference Bowles, Suárez, Prichard and Fisher2017) are a transitional stage in the alteration of the primary PGM and the formation of the placer nuggets. Their fragile textures indicate that they are the result of alteration of primary PGM and mobility of PGE.
Disordering
The Pt-Fe alloys in the weathered rocks show an almost continuous range of composition from Pt3Fe to PtFe and nearly PtFe3, indicating varying degrees of disordering due to low-temperature alteration. The alluvial Pt-Fe nuggets are closer to Pt3Fe.
Palladium
There is a significant loss of Pd relative to Pt. Ratios of Pt/Pd of <75 in the host rocks rise to 100–500 in the alluvial deposits demonstrating the greater mobility of Pd in solution.
Copper
Some Pt-Fe alloys in the placers contain a small proportion of Cu (0.3–2.7 wt.%) that increases the compositional variability and degree of disorder. Tulameenite, hongshiite and native Cu are present and appear to be a part of the involvement of Cu with some Pt-Fe nuggets being replaced by hongshiite at the edges. Apart from the presence of smaller grains of tulameenite, these features are not encountered in the fresh rocks.
Size
There is a three-orders of magnitude difference in size between the PGM seen in the fresh rocks compared with the larger alluvial mineral suite.
Delicate crystals
The delicate and well-preserved crystal features of some nuggets suggest a local origin; damaged nuggets have had a longer residence time in the rivers.
Organic coating
The coating of organic material on the nuggets is evidence of an association between the nuggets and organic material and may indicate involvement of organic material or bacterial action during formation of the nuggets.
PGM on ilmenite
Fine-grained PGM and grains of gold and silver occurring on the surface of ilmenite correspond to historic reports of an association of PGE with ilmenite in the rivers and may reveal initial stages of nugget growth.
Drainage
The presence of PGE-enriched horizons only cropping out in the Big Water drainage basin suggests that the PGE required to form the nuggets in both the Big Water and Alako streams were derived from PGE-bearing solutions draining both sides of the Mateki ridge rather than by mechanical erosion.
Taken together these points clearly indicate neoformation of the nuggets within the saprolite and alluvium after leaching of the PGE from the source rocks and transport of the PGE in solution. They are not indicative of derivation of all of the nuggets by erosion and mechanical transport.
Acknowledgements
This work has been made possible by the technical support of Drs. J. Rodríguez, R.S. García, J. Sangüesa, M. Sánchez-Lorda (SGIker, UPV/EHU) and generous funding for SS from the Department of Education, Universities and Research of the Basque Government (Refs. BFI-2011-254, IT762-13). Our manuscript was improved by the helpful advice of two reviewers who wish to remain anonymous. The panning and pitting were done by the people of York, Sierra Leone who we thank for their cheerful efforts.