Introduction
The Ediacaran Portfjeld Formation in North Greenland (Fig. 1) contains a suite of phosphatized microfossils, including acritarchs and embryo-like forms, comparable to the Doushantuo biota from Weng'an, South China (Willman et al., Reference Willman, Peel, Ineson, Schovsbo, Rugen and Frei2020). The assemblage includes animal-like eggs, embryos, acritarchs, and cyanobacteria, many of which are extremely well preserved. Helically coiled threads assigned to cyanobacteria are conspicuous and were described by Peel (Reference Peel1988).

Figure 1. Simplified geological map and lithostratigraphic column of the Portfjeld Formation. (1) Geological map showing the sampling site at the western end of Midsommersøer in North Greenland; (2) lithostratigraphic column through the Portfjeld Formation at eastern Midsommersøer where the fossiliferous horizon is located at a lower level than in the fossil locality at western Midsommersøer (see discussion in text; modified from Willman et al., Reference Willman, Peel, Ineson, Schovsbo, Rugen and Frei2020).
Simple cylindrical or tube-like fossils are common in the Proterozoic fossil record. Some of them are macroscopic, such as the carbonaceous Tawuia Hofmann in Hofmann and Aitken, Reference Hofmann and Aitken1979, and appear early in the Mesoproterozoic (Sun, Reference Sun1987). Others, such as the mineralized Cloudina Germs, Reference Germs1972, or the carbonaceous Sabellidites Yanishevsky, Reference Yanishevsky1926, emerge later, in the Neoproterozoic (Cortijo et al., Reference Cortijo, Martí Mus, Jensen and Palacios2010; Moczydłowska et al., Reference Moczydłowska, Westall and Foucher2014). In addition to macrofossils, many types of rod-like or filamentous microfossils are also preserved in the Ediacaran as compressions (Arvestål and Willman, Reference Arvestål and Willman2020), sedimentary casts and molds (Becker-Kerber et al., Reference Becker-Kerber, Baréa de Barros, Gomes Paim, Prado, Zucatti da Rosa, El Albani and Laflamme2021), and silicified or phosphatized replicas (Muscente et al., Reference Muscente, Hawkins and Xiao2015; Willman et al., Reference Willman, Peel, Ineson, Schovsbo, Rugen and Frei2020, respectively).
Due to their simple morphologies, the phylogenetic affinity of many of these fossils is controversial, with interpretations ranging from colonial bacteria to multicellular algae and to metazoans, often with annelid or cnidarian affinities (Butterfield et al., Reference Butterfield, Knoll and Swett1994; Butterfield, Reference Butterfield2004; Sharma et al., Reference Sharma, Mishra, Dutta, Banerjee and Shukla2009; Yang et al., Reference Yang, Steiner, Schiffbauer, Selly, Wu, Zhang and Liu2020). Firm assessment, however, relies on preservation of specific and identifiable traits, and exceptional preservation is therefore the key to our understanding of these groups of simple fossils.
Peel (Reference Peel1988) described well-preserved, annulated, non-branching and helically coiled specimens with a parallel-sided, sinistral helix as Jiangispirellus groenlandicus Peel, Reference Peel1988 (Fig. 2.1–2.3). Although numerous in the Portfjeld biota, they are usually preserved as fragments, and were interpreted as prokaryotic cyanobacteria by Peel (Reference Peel1988). The spiral threads are naked trichomes and the annulation may be represented both by external molds of the trichome or internal molds showing the constituent cell walls; they lack a covering sheath (Peel, Reference Peel1988; Peel and Willman, Reference Peel and Willman2022; Fig. 2.1–2.3). Jiangispirellus specimens are associated with smooth, non-annulated filaments that were assigned to Spirellus Jiang in Luo et al., Reference Luo, Zhiwen, Xiche, Xueliang and Lin1982, in which the enclosing sheath (Fig. 2.4–2.6) was often calcified prior to the diagenetic phosphatization that affects all specimens from the Portfjeld biota (Peel, Reference Peel1988; Willman et al., Reference Willman, Peel, Ineson, Schovsbo, Rugen and Frei2020; Peel and Willman, Reference Peel and Willman2022).

Figure 2. Examples of Jiangispirellus and Spirellus. (1–3) Holotype of Jiangispirellus groenlandicus trichome (MGUH 17.571) described in Peel (Reference Peel1988); (4–6) Spirellus shankari (Singh and Shukla, Reference Singh and Shukla1981); (4) un-mineralized sheath with slight deformation of the helix (MGUH 17.576); (5) with originally calcified sheath (MGUH 17.582); (6) with degraded un-mineralized sheath (PMU 39236/1). All specimens phosphatized and from GGU sample 271769, except (6), which is from 271770. Scale bar = 50 μm for all specimens.
Here, we describe enigmatic specimens in which annulation comparable to that seen in Jiangispirellus is preserved together with prominent internal tubules. In one of these, two tubules are initially present within an outer annulated covering, and one of these subsequently branches such that all three tubules run along the preserved length of the arcuate specimen (Fig. 3.1–3.5; see Fig. 3.6–3.8 and Fig. 4 for poorly preserved specimens that show some, but not all, features described in the best-preserved specimen). In a second specimen, an inner tubule is continuous through several consecutive volutions of the embracing Jiangispirellus helix (Fig. 5.1, 5.2). Comparison of the two specimens raises questions concerning their interpretation—in particular if they represent distinct organisms or result from the postmortal occupation of diagenetically formed cavities within mineralized Jiangispirellus trichomes (Peel and Willman, Reference Peel and Willman2022).

Figure 3. Holotype of Portfjeldia aestatis n. gen. n. sp. and other unnamed tubular fossils showing various types of similar internal structures: (1–5) Portfjeldia aestatis n. gen. n. sp. (PMU 36870/2) from various viewpoints displaying two tubules interpreted as being originally enclosed by a now partly degraded external sheath; (3) enlargement of lower left of (4) with lines indicating three tubes rather than two, indicating a possible triradial symmetry; arrows in (2) and (4) show possible branching and development of daughter tube; (5) possible third tubule originating as a ridge near the other extremity. (6) Broken tube (PMU 39237/1), possibly related to the problematic spiral tube also described; arrow indicates internal groove also visible in (4). (7) Broken tube (PMU 38168/2) showing three possible tubules indicating a triradial structure (white lines). (8) Single whorl of a degraded, annulated helix with internal tubular structure (arrow) (PMU 36876/4). Scale bar 100 μm for (1, 2, 6–8) and 50 μm for (3–5).
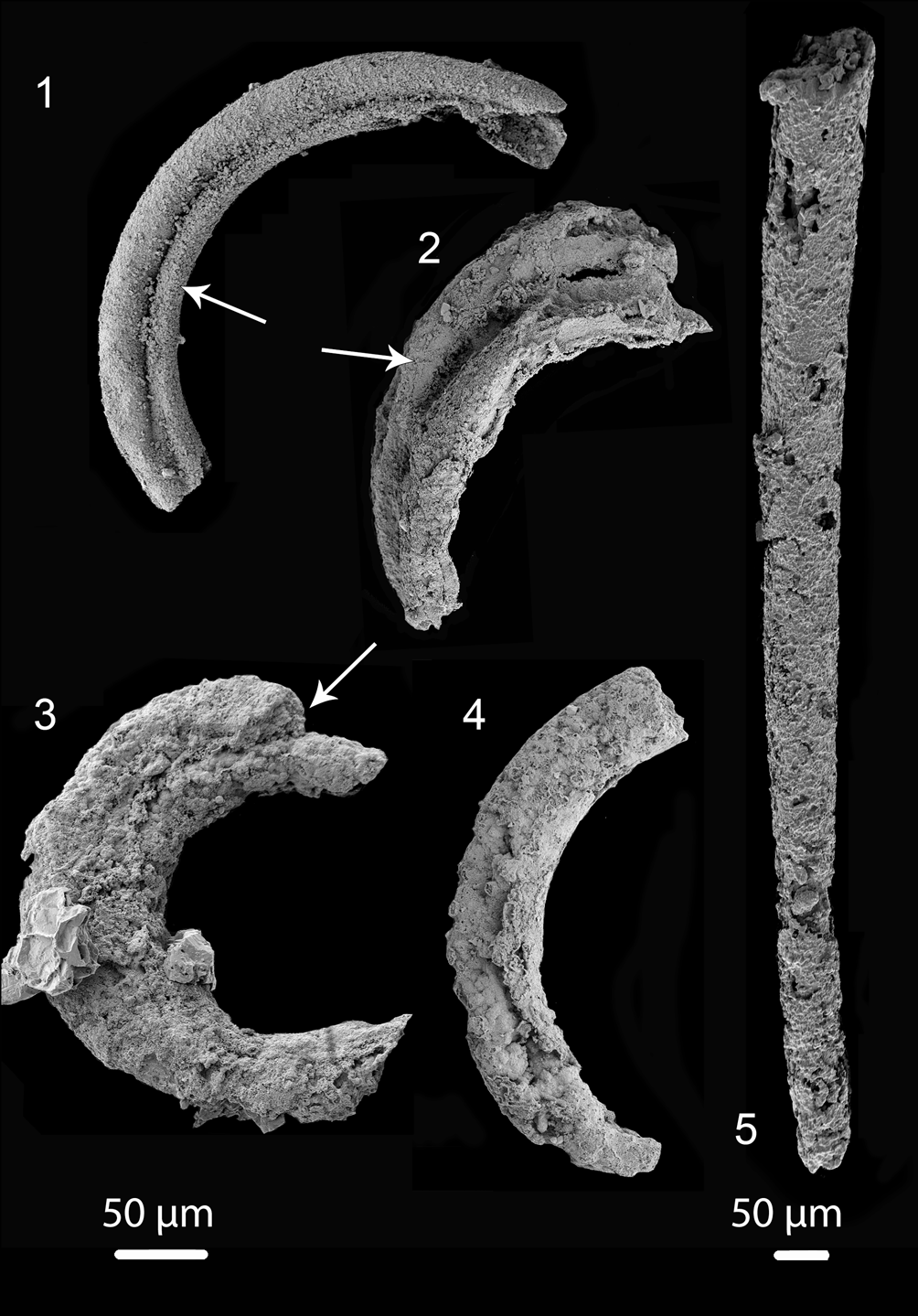
Figure 4. Tentative Portfjeldia aestatis n. gen. n. sp. in different states of preservation: (1–3) showing two tubules that appear to be branched and slightly separated from each other (arrows) (1) (PMU 36865/3), (2) (PMU 38169/2); (3) (PMU 36873/3); (4) outer annulated cover casing two tubes (PMU 39238/1); (5) slender slightly curved tube, with poorly preserved annulation at its narrow end (PMU 39239/1). Tentatively this single tube may be placed within Portfjeldia aestatis n. gen. n. sp., leading to the suggestion that 1–3 tubules may be present in Portfjeldia n. gen. Left scale bar 50 μm for (1–4), right scale bar 50 μm for (5).

Figure 5. Problematic helically spiraled tubular organism. (1, 2) Preserved as an internal tube inside an outer Jiangispirellus “trichome,” showing traces of cell wall (PMU 36870/3); (3) remains of a possible branching organism (arrow) within a “trichome” (PMU 36868/4); (4) preserved “trichome” of Jiangispirellus groenlandicus with internal secondary phosphatization (PMU 36874/5). Note that no internal septa are preserved, indicating that the phosphatized “trichome” covered a hollow chamber. Scale bar 100 μm for (1, 3, 4) and 50 μm for (2).
As far as we are aware, internal tubular structures of this kind have not been reported in known fossil or Recent cyanobacterial morphologies, and such structures are better interpreted as algae (Cunningham et al., Reference Cunningham, Vargas, Pengju, Belivanova, Marone, Martínez-Pérez, Guizar-Sicairos, Holler, Bengtson and Donoghue2015). However, Peel and Willman (Reference Peel and Willman2022) described numerous thread-like and branching cavity-dwelling organisms of uncertain, likely fungal, origin from within postmortal cavities formed within diagenetically mineralized specimens of Jiangispirellus derived from the same samples.
Geological setting
The carbonate-dominated Portfjeld Formation in southern areas of North Greenland unconformably overlies Mesoproterozoic sandstones of the Independence Fjord Group and outliers of Neoproterozoic tillites and associated carbonates inferred to be of Marinoan age (Higgins et al., Reference Higgins, Ineson, Peel, Surlyk and Sønderholm1991; Sønderholm and Jepsen, Reference Sønderholm and Jepsen1991; Ineson and Peel, Reference Ineson and Peel2011; Willman et al., Reference Willman, Peel, Ineson, Schovsbo, Rugen and Frei2020; Peel and Willman, Reference Peel and Willman2022). The Portfjeld Formation is in turn overlain unconformably by the Buen Formation, consisting mainly of transgressive fluvial to marine-shelf siliciclastic sediments (Fig. 1). The sandstone-dominated lower member of the Buen Formation yields trace fossils of early Cambrian age (Bryant and Pickerill, Reference Bryant and Pickerill1990), while the mudstone-dominated upper member contains rich faunas of Cambrian Series 2 (Stage 3–4) age (Peel and Willman, Reference Peel and Willman2018; Wallet et al., Reference Wallet, Slater, Willman and Peel2021).
Lithologically, the Portfjeld Formation can be divided into two separate stratigraphic packages that are separated by a widely distributed karstic unconformity (Willman et al., Reference Willman, Peel, Ineson, Schovsbo, Rugen and Frei2020). The lower unit is ~170 m thick and dominated by dolostones. It represents two transgressive-regressive cycles of a carbonate ramp and consists of mid- to outer-ramp hummocky cross-stratified, intraclast-rich, grainstones and cherty dark dolostones and typical inner ramp ooid-pisoid grainstones, with varied microbial facies. The upper package (thickness 70–90 m) records a transgressive succession of fluvial sandstones and mudstones followed by high-energy shallow marine carbonate and siliciclastic facies, truncated upwards by dolines and karstic collapse structures at the boundary between the Portfjeld and Buen formations (Fig. 1).
During the Ediacaran, at the time of the deposition of the lower Portfjeld Formation, North Greenland was part of the geographically isolated paleocontinent Laurentia, estimated to have lain south of the Equator at paleolatitudes of 30–75°S (Li et al., Reference Li, Evans and Halverson2013; Zhang et al., Reference Zhang, Li, Jiang, Evans, Dong, Wu, Yang, Liu and Xiao2015; Willman et al., Reference Willman, Peel, Ineson, Schovsbo, Rugen and Frei2020).
Material and methods
Stromatolitic dolostone was collected by J.S. Peel and P. Frykman on 22 July 1978 from ~70 m above the base of the Portfjeld Formation on the north side of Wandel Dal, west of Øvre Midsommersø (82°14′N, 36°06′W; Peel, Reference Peel1988). The original sample (GGU 271769) from this horizon, described by Peel (Reference Peel1988), and an identical split (GGU 271770), from which all current material was derived, were located by Willman et al. (2020, fig. 2) relative to a measured section to the east, overlooking Nedre Midsommersø, the easternmost of the two lakes comprising Midsommersøer (82°15′N, 33°25′W; Fig. 1.1), where the fossiliferous horizon lies at an equivalent stratigraphic level of ~50 m above the base of the formation where it transgressed the uneven surface beneath. The samples were macerated in weak acetic or formic acid and sieved in fractions down to 50 μm. The residues containing phosphatized microfossils were hand-picked under a binocular microscope. Specimens were mounted on aluminum stubs and imaged under high power using a Zeiss Supra 35VP scanning electron microscope. Images were cropped using Affinity Photo and Affinity Design.
Repositories and institutional abbreviations
GGU prefix indicates a sample collected during regional mapping campaigns of Grønlands Geologiske Undersøgelse (Geological Survey of Greenland, now Geological Survey of Denmark and Greenland; GEUS) in the Northeast Greenland National Park in compliance with operative conditions for access to, and activities within the park. MGUH indicates a specimen deposited in the paleontological type collection of the Natural History Museum of Denmark, Copenhagen. PMU indicates a specimen deposited in the paleontological type collection of the Museum of Evolution, Uppsala University, Sweden.
Systematic paleontology
Incertae sedis
Genus Portfjeldia new genus
Type species
Portfjeldia aestatis n. gen. n. sp., by monotypy, from the lower Portfjeld Formation (Ediacaran) of southern Peary Land, North Greenland.
Diagnosis
As for the type species by monotypy.
Etymology
After its derivation from the Portfjeld Formation.
Remarks
Portfjeldia n. gen. is distinguished from the parallel-sided helix of Jiangispirellus by its shallow crescentic form and the presence of internal tubules. Thus, fragments of Jiangispirellus have a tighter curvature than those of Portfjeldia n. gen. Jiangispirellus is considered to be a trichome with internal transverse partitions interpreted as cell walls (Peel, Reference Peel1988) that have not been observed in Portfjeldia n. gen. The two forms are similar in terms of the dimensions of the annulation on the outer surface.
Portfjeldia n. gen. is morphologically similar to a specimen of the much larger Ramitubus decrescens Liu et al., Reference Liu, Xiao, Yin, Zhou, Gao and Tang2008, from the Weng'an biota of South China (Liu et al., Reference Liu, Xiao, Yin, Zhou, Gao and Tang2008, text-fig. 4) where daughter tubules emerge from within an annulated mother tube. The mother tube in Ramitubus is compartmentalized by transverse cell walls, but this is neither seen in the illustrated specimen (Liu et al., Reference Liu, Xiao, Yin, Zhou, Gao and Tang2008, text-fig. 4), nor in the tubules of the Chinese specimen or holotype of Portfjeldia aestatis n. gen. n. sp. from Greenland (Fig. 3.1). The presence of a compartmentalized mother tube is not demonstrated in the Greenland specimen, but is inferred from the comparison to Ramitubus and the adpressed annulated outer covering.
Portfjeldia aestatis new species
Figures 3.1–3.5, ?4
Holotype
PMU 36870/2 from GGU sample 271770, Portfjeld Formation, southern Peary Land.
Diagnosis
Crescentic, seemingly with 1–3 slender, slowly expanding, tubules contained within an adpressed annulated outer covering.
Description
The holotype and best preserved specimen (Fig. 3.1–3.5) is crescent shaped and consists of an outer tubular covering with a well-developed, annulated, outer surface, but without demonstrated internal compartmentalization. In cross-section the annulated tube is not circular, but shows longitudinal grooves and folds because it is adpressed against the emerging inner tubules (Fig. 3.1), which may indicate it was originally flexible. Inside the tube, two smaller tubules run side by side along the length of the specimen. The two tubules are circular and share a common, now mineralized, wall, creating an external longitudinal groove between them (Fig. 3.2). The presence of a third tubule is suggested by the triradial cross-section at one specimen extremity (Figs. 3.3, 3.4 left), with the tubule originating as a ridge near the other extremity (Fig. 3.4, 3.5). This ridge originates close to the extremity and appears to represent a branch from the adjacent tubule. The specimen as preserved is a fragment ~400 μm long and 45 μm wide. The outer diameter of each tubule is ~20 μm and the internal cavity is 10 μm, but the thickness of the tubule walls reflects diagenetic mineralization rather than the original dimensions. The tubules lack preserved internal structures.
Etymology
From the Latin aestas, meaning summer, reflecting the occurrence near Midsommersøer, the mid-summer lakes.
Remarks
Several fragments of possible Jiangispirellus appear to have some features that are present in Portfjeldia aestatis, including similar tubes preserved as a channel impressed into an inner phosphatized layer (Fig. 3.6), possible triradial symmetry (Fig. 3.7), or internal tubules (Fig. 3.8). In addition to the holotype, in which the outer annulated covering is only partly phosphatized, four poorly preserved specimens (Fig. 4.1–4.4) show two tubules that appear to be branched and slightly separated from each other; an outer annulated covering is recognized in only one of these (Fig. 4.4). A single, slightly curved, slender tube, with poorly preserved annulation at its narrow end (Fig. 4.5) may be in place here, leading to the tentative interpretation that 1–3 tubules may be present in Portfjeldia aestatis n. gen. n. sp. The shallowly arcuate form of Portfjeldia aestatis n. gen. n. sp. is distinct from the parallel-sided, tight, sinistrally coiled helix of Jiangispirellus groenlandicus, but evidence of degradation from the helical form characteristic of Jiangispirellus groenlandicus is lacking. Furthermore, the external annulated layer in Portfjeldia aestatis n. gen. n. sp. is closely molded to the form of the inner tubules (Fig. 3.1), unlike the circular cross-section of Jiangispirellus groenlandicus.
When the Portfjeld biota was first described (Peel, Reference Peel1988), another filamentous fossil, Spirellus shankari (Singh and Shukla, Reference Singh and Shukla1981), was found co-occurring with Jiangispirellus groenlandicus in the same sample. Jiangispirellus groenlandicus is distinguished from Spirellus shankari in that the latter has a smooth outer surface considered to represent a filament with an outer sheath (Fig. 2). Peel (Reference Peel1988) considered that the two types were not taphonomic variants. Thus, Jiangispirellus was not a Spirellus that had lost its external sheath but a separate species, primarily due to the fact that Spirellus filaments from the Portfjeld Formation generally have smaller dimensions than Jiangispirellus groenlandicus, although these may be greatly increased by carbonate mineralization of the sheath. The integrity of Jiangispirellus was recognized by Knoll (Reference Knoll1992) and Mankiewicz (Reference Mankiewicz1992).
Problematic spiral tube
Figure 5.1, 5.2
Description
A robust parallel-sided, currently phosphatized, un-ornamented tube up to almost 2 mm in length, is of uniform width with an outer diameter of ~20 μm and an internal diameter of ~10 μm. The fossil is located within the parallel-sided sinistral helix of a postmortally phosphatized specimen of Jiangispirellus groenlandicus and can be traced, with a slight interruption due to breakage, through three or four consecutive coils of the helix that are recognized from traces of the inner (adaxial) surface (Fig. 5.1). The tubule is coiled obliquely within the Jiangispirellus helical coil such that it translates from the upper part of the first coil (uppermost, as oriented in Fig. 5.1) to the lower part of the final preserved coil (lowermost, as oriented in Fig. 5.1). In the first coil (Fig. 5.2), the annulation of the Jiangispirellus trichome persists, and is imposed on the surface of the tubule, while traces of compartmentalization (mineralized cell walls) are retained within the Jiangispirellus trichome. The tubule is broken at each end and displays no evidence of branching or internal structure.
Materials
One well-preserved specimen (PMU 36870/3) and several fragments from GGU sample 271770, Portfjeld Formation, southern Peary Land.
Remarks
Several fragments of Jiangispirellus appear to show similar tubes preserved as a channel impressed into an inner phosphatized layer (Fig. 3.6, see also Fig. 4.1).
The problematic spiral tube differs from Portfjeldia aestatis n. gen. n. sp. in being unequivocally located within the tightly coiled, parallel-sided helix of a diagenetically phosphatized specimen of Jiangispirellus groenlandicus, although the association with the host is interpreted as being postmortal—a consequence of the nature of the coiling of the tubes within Jiangispirellus. Tubules of Portfjeldia aestatis n. gen. n. sp. differ in having a low rate of expansion while the problematic tube is parallel-sided. Portfjeldia aestatis n. gen. n. sp. has an outer annular covering similar to that of circular coils of the trichome in Jiangispirellus groenlandicus, but this is closely adpressed to the tubules (Fig. 3.1), a feature also seen in some specimens of Ramitubus decrescens (illustrated by Liu et al., Reference Liu, Xiao, Yin, Zhou, Gao and Tang2008, text-fig. 4). Furthermore, Portfjeldia aestatis n. gen. n. sp. differs in its shallow arcuate form from the tight helix of Jiangispirellus that accommodates the problematic tube. A taphonomic variant of a problematic, branching, inner tube is seen in Figure 5.3. Secondary phosphatization of a Jiangispirellus groenlandicus (Fig. 5.4) shows no internal septa, indicating that the “trichome” might have been hollow, which is similar to the preservation of the problematic spiral tube.
Interpretation of the tubes
Various problematic tube-like structures of micro- to macroscopic size have been reported from different Ediacaran and older terranes worldwide. The phylogenetic affinities of most of these morphologically simple structures are debated, but the majority of the macroscopic tubes are considered to be metazoan (Liu et al., Reference Liu, Xiao, Yin, Zhou, Gao and Tang2008; but see Cunningham et al., Reference Cunningham, Liu, Bengtson and Donoghue2017). Some macroscopic tubes (e.g., Cloudina Germs, Reference Germs1972, Shaanxilithes Xing, Yue, and Zhang in Xing et al., Reference Xing, Ding, Luo, He and Wang1984, and Sinotubulites Chen et al., Reference Chen, Chen and Qian1981) also may represent the earliest biomineralizing organisms, whereas others, such as Conotubus Zhang and Lin in Lin et al., Reference Lin, Zhang, Zhang, Tao and Wang1986, may be only weakly mineralized (Hua et al., Reference Hua, Chen and Yuan2007). As a consequence of their novel construction, some of these early tubular organisms are important in our understanding of the earliest metazoans. Generally, however, these stacked, cone-in-cone structures have a diameter normally reaching several millimeters in length (Germs, Reference Germs1972; Yang et al., Reference Yang, Steiner, Schiffbauer, Selly, Wu, Zhang and Liu2020), considerably larger than the tubular fossils reported herein. The slightly curved or sinuous, and sometimes branching morphology (Cortijo et al., Reference Cortijo, Cai, Hua, Schiffbauer and Xiao2015; Wang et al., Reference Wang, Zhang, Zhang, Cui and Li2021) seen in many of the macroscopic tubes is shared with the specimens described here. However, the size differences and overall differences in morphology mean that the biological affinities of the organisms described here should not be directly compared with this group of macroscopic, tubular, and probably mineralized fossils.
Interpretation of the paleobiological affinity of microscopic filamentous structures offers several phylogenetic alternatives. Depending on size and shape, microscopic filaments are usually interpreted as cyanobacteria, algae, or fungi, but it is often challenging to even distinguish between prokaryotic and eukaryotic organisms due to overlap in size and morphology (Knoll et al., Reference Knoll, Javaux, Hewitt and Cohen2006). In an attempt to describe different grades of filamentous organisms Butterfield (Reference Butterfield2009) proposed a set of morphological groups, or body plans: simple uniseriate filaments; simple multiseriate filaments; simple coenocytic/semi-coenocytic filaments; branched coenocytic/semi-coenocytic filaments; and complex multicellular filaments. These grades represent everything from prokaryotes to multicellular eukaryotes. Consequently, the morphology of the fossils described here must therefore be compared with several different groups before attempting to interpret their affinity.
Affinity to cyanobacteria
Bundled filaments of similar size to the specimens described here, enclosed in a sheath, are known from various fossil cyanobacteria, such as Microcoleus Gomont, Reference Gomont1892, and Schizothrix Gomont, Reference Gomont1892 (Lee and Golubic, Reference Lee and Golubic1998; Castenholz, Reference Castenholz, Boone, Castenholz and Garrity2001), and even in sulfur-oxidizing bacteria such as Thioploca Lauterborn, Reference Lauterborn1907 (Teske and Nelson, Reference Teske, Nelson, Dworkin, Falkow, Rosenberg, Schleifer and Stackebrandt2006). Other cyanobacteria, such as Trichodesmium Gomont, Reference Gomont1892, or Subtifloria Maslov, Reference Maslov1956, a Cambrian calcareous tubular filament with cable-like bundled filaments, can be compared morphologically to our specimen. The bundled filaments in those mentioned are, however, not enclosed within a sheath (Maslov, Reference Maslov1956; Riding, Reference Riding and Riding1991a, Reference Riding and Ridingb), and therefore not closely related to our specimens.
A collection of three-dimensionally preserved phosphatized fossils described from the latest Ediacaran in Shaanxi Province, China (Min et al., Reference Min, Hua, Liu, Sun, Cui and Dai2020) includes Subtifloria. The assemblage was identified as a mixture of (originally calcified) oscillatorialeans and rivulariaceans, the latter being among the most complex cyanobacteria at the present day. The larger assemblage described from China is similar to the Portfjeld biota in containing Obruchevella Reitlinger, Reference Reitlinger1948 (Min et al., Reference Min, Hua, Liu, Sun, Cui and Dai2020, fig. 4A, E), which occurs in North Greenland together with the other helical forms, Jiangispirellus and Spirellus (Peel, Reference Peel1988), but contains nothing resembling Portfjeldia aestatis n. gen. n. sp. More importantly, however, the combination of biomineralized skeletons, algae, cyanobacteria, and possible metazoans in the Chinese and Greenland Ediacaran biotas shows that considerable diversity remains to be discovered in the phosphatized micro-remains.
Modern, more diversified and complex cyanobacteria, such as the Order Nostocales, develop resting cells, called akinetes, from solitary cells towards the end of the vegetative growth period. The akinetes can germinate to form new filaments when conditions improve (Komárek and Johansen, Reference Komárek, Johansen, Wehr, Sheath and Kociolek2015), but unlike the individual structures here, these are often associated with microbial mats. Although complexity is an undefined term, our specimens undoubtedly have a more complex grade of organization than any standard photoautotrophic cyanobacteria, which rules out a relationship with filamentous cyanobacteria. Consequently, if the tubules are part of an integrated organism, the organism does not belong within the cyanobacteria.
Another Recent cyanobacterium, Blennothrix geneshi Watanabe and Komárek, Reference Watanabe and Komárek1989 (Oscillatoriaceae), is characterized by the presence of several trichomes in a lamellated sheath in which the trichomes can branch or, rarely, false branch (Jiménez et al., Reference Jiménez, Magos and Collado-Vides2005). This construction is similar to that seen in Portfjeldia aestatis n. gen. n. sp. However, false branching has annulated filaments within the outer sheath whereas Portfjeldia n. gen. displays branching within an outer annulated sheath.
Without the internal problematic spiral tubule, the specimen presented here (Fig. 5.1, 5.2) would be interpreted as a typical fragment of the cyanobacterium Jiangispirellus groenlandicus due to the presence of the clear annulations and traces of compartmentalization (Fig. 5.1, 5.2). The problematic spiral tube is, however, clearly a cavity-dwelling organism within the postmortal diagenetic cavity formed by a phosphatized specimen of Jiangispirellus (similar to other cavity-dwellers described by Peel and Willman, Reference Peel and Willman2022). While the shape of the problematic spiral tube is comparable to many cyanobacteria, its occurrence within such a closed cavity may hinder its interpretation as a photoautotrophic cyanobacterium.
Affinity to algae
In modern ecosystems, algae are important and diverse components of both marine and terrestrial ecosystems (Stevenson, Reference Stevenson2014), and thallus structures can vary from simple and unbranched to complex branching, and from non-motile to motile. Precambrian filamentous eukaryotic algae are usually identified by showing evidence of branching or heteromorphic cells (Butterfield, Reference Butterfield2004; Tang et al., Reference Tang, Pang, Yuan and Xiao2020) whereas organisms that are small (generally <50 μm, but there is overlap between large cyanobacteria and small algae) and do not show evidence for eukaryotic or multicellular construction are considered to be prokaryotes (cyanobacteria) (Butterfield et al., Reference Butterfield, Knoll and Swett1994). Filamentous red algae are probably the most ancient group of algae (1.6 Ga old, sensu Bengtson et al., Reference Bengtson, Sallstedt, Belivanova and Whitehouse2017), but other forms, including the filamentous green algae Proterocladus Butterfield in Butterfield et al., Reference Butterfield, Knoll and Swett1994, appear shortly after (1.0 Ga, sensu Tang et al., Reference Tang, Pang, Yuan and Xiao2020) and the group diversifies throughout the Neoproterozoic (Butterfield and Rainbird, Reference Butterfield and Rainbird1998). It is likely that algae played an important role in the metazoan radiation during the Ediacaran-Cambrian transition primarily due to large primary productivity in shallow epeiric seas, and establishing new ecological tiering (LoDuca et al., Reference LoDuca, Bykova, Wu, Xiao and Zhao2017).
An algal affinity for the organisms described here is possible, even likely. The possible budding/branching structure in Portfjeldia aestatis n. gen. n. sp. (Fig. 3.4) is a typical algal trait, as is the curved, generally tubiform morphology. Filamentous algae can also show annulations similar to the outer structure of the Portfjeld organisms. However, as far as we are aware, structures comparable to the continuous internal tubules within an annulated outer wall are not known from any algal groups. Similarly, the single problematic spiral tube is readily interpreted as an alga.
Affinity to Metazoa
The presence of metazoans in the Ediacaran can be deduced from various complex trace fossils (Budd and Jensen, Reference Budd and Jensen2000) or from body fossils, such as the bilaterian Kimberella Glaessner and Wade, Reference Glaessner and Wade1966, although it is well understood that many other components of the biota are difficult to interpret (Seilacher, Reference Seilacher1992; Peterson et al., Reference Peterson, Waggoner and Hagadorn2003). Nevertheless, mineralized tubular organisms, a construction facilitated by high levels of carbonate saturation, are common in the Ediacaran (Wood et al., Reference Wood, Ivantsov and Zhuravlev2017). For example, the anabaritids, a group of early skeletal fossils, are globally distributed in strata of Late Precambrian–Cambrian age (Kouchinsky et al., Reference Kouchinsky, Bengtson, Feng, Kutygin and Vaĺkov2009; Zhuravlev et al., Reference Zhuravlev, Linan, Vintaned, Debrenne and Fedorov2012). Anabaritids are tubular organisms that grew by apertural accretion and their main morphological feature is a triradial symmetry seen as lobes and grooves (Kouchinsky and Bengtson, Reference Kouchinsky and Bengtson2002). Their biological affinity is uncertain, but points to Cnidaria. A triradial symmetry reminiscent of anabaritids is seen in Figure 3.3, but the triradial symmetry in Portfjeldia n. gen. represents three conjoined tubes whereas that in anabaritids is developed within a single tube. Other anabaritids are curved and display transverse sections comparable to our specimen (compare with Devaere et al., Reference Devaere, Korn, Ghaderi, Struck and Bavandpur2021, fig. 11G, P).
Wutubus annularis Chen et al., Reference Chen, Zhou, Xiao, Wang, Guan, Hua and Yuan2014, from the Denying Formation of the Yangtze Gorges area of South China, is an annulated tubular or narrowly conical fossil in which annulae are arranged uniserially to form an erect benthic organism of unknown phylogenetic affinity (Chen et al., Reference Chen, Zhou, Xiao, Wang, Guan, Hua and Yuan2014). Wutubus is much larger (length 20–180 mm) than the microscopic Portfjeld specimens described here.
Sinocyclocyclicus guizhouensis Xue, Tang, and Yu, Reference Xue, Tang and Yu1992, is another tube interpreted as a possible stem cnidarian (Liu et al., Reference Liu, Xiao, Yin, Zhou, Gao and Tang2008), although Cunningham et al. (Reference Cunningham, Vargas, Pengju, Belivanova, Marone, Martínez-Pérez, Guizar-Sicairos, Holler, Bengtson and Donoghue2015) considered it to be an alga. Some Sinocyclocyclicus (Xiao et al., Reference Xiao, Yuan and Knoll2000, fig. 2 H, I) are morphologically similar to the fossils described herein.
As with Ramitubus Liu et al., Reference Liu, Xiao, Yin, Zhou, Gao and Tang2008, and other forms reviewed by Cunningham et al. (Reference Cunningham, Vargas, Pengju, Belivanova, Marone, Martínez-Pérez, Guizar-Sicairos, Holler, Bengtson and Donoghue2015), the original probably organic tubes show cross-wall compartmentalization and may be branched but are generally a size order larger than the present material. However, specimens of Ramitubus decrescens (illustrated by Liu et al., Reference Liu, Xiao, Yin, Zhou, Gao and Tang2008, text-fig. 4) show several tubules and an associated outer annulated covering similar to that also present in Portfjeldia aestatis n. gen. n. sp. (Fig. 3.4). As mentioned, the metazoan affinities have been questioned and alternative explanations, such as cyanobacteria (Sinocyclocyclicus) or algae (Ramitubus), are considered more likely (Sun et al., Reference Sun, Yin, Donoghue, Liu, Shang and Zhu2019).
Our specimens do not show any evidence of flexible bending or compression, which might indicate that they are actually originally mineralized rather than organic. However, while specimens of Spirellus in the Portfjeld biota were often degraded prior to phosphatization (Fig. 2), the co-occurring helices of the naked trichomes of Jiangispirellus usually display only minimal deformation.
Other alternatives
The single problematic, spiral, hollow tube is associated with traces of cell walls preserved on the inner surface of the Jiangispirellus helix (Fig. 5.2), but there is no direct evidence that it penetrated these walls in life. On the contrary, its migration in consecutive revolutions from the top of the first coil to the bottom in the final coil would not be possible if there were septa separating the cells unless the septa were penetrated. The problematic spiral tube is therefore interpreted as a cavity-dwelling organism occupying the postmortally formed cavity formed within a partially mineralized specimen of Jiangispirellus groenlandicus.
If the tubules of Portfjeldia aestatis n. gen. n. sp. are not part of an integrated organism such as an alga, they could be interpreted as a cavity-dwelling microorganism (cryptoendolith, sensu Stockfors and Peel, Reference Stockfors and Peel2005; see discussion of terminology in Peel and Willman, Reference Peel and Willman2022) like the problematic spiral tube, akin to other forms described by Peel and Willman (Reference Peel and Willman2022). These organisms inhabit the postmortally formed empty spaces within mineralized skeletons of other organisms or hard substrates. Cavity-dwelling organisms can be representatives of different phyla, such as bacteria, algae, or fungi (Campbell, Reference Campbell1982; Gan et al., Reference Gan, Luo, Pang, Zhou, Zhou, Wan, Li, Yi, Czaja and Xiao2021; Peel and Willman, Reference Peel and Willman2022), but described cavity-dwelling organisms do not show the level organization seen here. A complex of tubular structures within a specimen of Jiangispirellus (Fig. 3.8) is closely similar to cavity-dwelling organisms from the same sample illustrated by Peel and Willman (Reference Peel and Willman2022, fig. 3.14). However, unlike most cryptoendoliths, the tubules in the holotype of Portfjeldia aestatis n. gen. n. sp. are not thread-like, irregular, or part of a meshwork, but more robust, forming an arcuate bundle of semi-parallel tubules. Their form is therefore interpreted as an original morphological feature, similar to the branching Ramitubus illustrated by Liu et al. (Reference Liu, Xiao, Yin, Zhou, Gao and Tang2008), and not the result of cavity-dwelling behavior.
The possibility that the tubules of Portfjeldia aestatis n. gen. n. sp. and the problematic spiral tube may represent a parasitic infestation, or even some kind of symbiotic relationship, is considered unlikely.
Conclusions
Portfjeldia aestatis n. gen. n. sp. is probably an alga, although its diagenetically mineralized tubules do not preserve internal structures that might indicate a cellular compartmentalization. Portfjeldia aestatis n. gen. n. sp. can be compared morphologically with the larger Ramitubus, originally described from the Weng'an biota of South China, and may be related, in particular if one accepts the alternative suggestion that Ramitubus is an alga. The problematic long, single, slender tubule also lacks preserved internal structures. It occurs in diagenetic cavities formed within postmortally phosphatized specimens of the cyanobacterium Jiangispirellus groenlandicus, an environment that likely favors interpretation as an alga rather than a phototrophic cyanobacteria.
Acknowledgments
Samples were collected during the North Greenland Project (1978–1980) of Grønlands Geologiske Undersøgelse. J.R. Ineson (Copenhagen) is thanked for sharing his knowledge of the Portfjeld Formation. Constructive comments from two anonymous reviewers, editor E. Currano, and associate editor J. Schiffbauer are greatly acknowledged.