Significant outcomes
-
Mas plays an important role in the neurobiology of depression.
-
Genetic deletion of the Mas leads to depressive-like behaviour and reduction of BDNF levels in prefrontal cortex (PFC) and hippocampus.
-
MrgD deficiency in male mice does not cause depressive-like behaviour.
Limitations
-
Given the fact that depression is more prevalent in females, this study has the limitation of having been performed only in adult male mice.
-
We performed only two behavioural tests, the FST and TST. It would be interesting to confirm our data in different preclinical tests, such as the sucrose preference test.
Introduction
The renin–angiotensin system (RAS) is well known as an essential system for hydroelectrolyte and cardiovascular homeostasis (Ferrario and Schiavone, Reference Ferrario and Schiavone1989; Bader, Reference Bader2010). However, crucial RAS effects have been demonstrated in central nervous system (CNS) beyond cardiovascular regulation (Lippoldt et al., Reference Lippoldt, Paul, Fuxe and Ganten1995; von Bohlen und Halbach and Albrecht, Reference von Bohlen und Halbach and Albrecht2006; Passos-Silva et al., Reference Passos-Silva, Brandan and Santos2015; Gironacci et al., Reference Gironacci, Cerniello, Longo Carbajosa, Goldstein and Cerrato2014; Almeida-Santos et al., Reference Almeida-Santos, Kangussu and Campagnole-Santos2017; Nakagawa and Sigmund, Reference Nakagawa and Sigmund2017; Santos et al., Reference Santos, Sampaio, Alzamora, Motta-Santos, Alenina, Bader and Campagnole-Santos2018; Jackson et al., Reference Jackson, Eldahshan, Fagan and Ergul2018).
Growing evidence has indicated a role for RAS components in the neurobiology of anxiety and depression (Kangussu et al., Reference Kangussu, Almeida-Santos, Bader, Alenina, Fontes, Santos, Aguiar and Campagnole-Santos2013; Vian et al., Reference Vian, Pereira, Chavarria, Köhler, Stubbs, Quevedo, Kim, Carvalho, Berk and Fernandes2017; Chrissobolis et al., Reference Chrissobolis, Luu, Waldschmidt, Yoakum and D’Souza2020; Kangussu, Reference Kangussu2020). In general, hyperactivation of classical RAS axis [angiotensin-converting enzyme (ACE)/Angiotensin (Ang) II/AT1 receptor] induces anxiety and depression-like behaviours in animals and humans. In contrast, pharmacological blockade of this axis through angiotensin-converting enzyme inhibitors (ACEi) and angiotensin receptor blockers (ARB) or activation of the counter-regulatory RAS axis [angiotensin-converting enzyme 2 (ACE2)/Ang-(1-7)/Mas receptor] induces antidepressant and anxiolytic effects (Zubenko and Nixon, Reference Zubenko and Nixon1984; Croog et al., Reference Croog, Levine, Testa, Brown, Bulpitt, Jenkins, Klerman and Williams1986; Germain and Chouinard, Reference Germain and Chouinard1988; Gard, Reference Gard2004; Saavedra et al., Reference Saavedra, Ando, Armando, Baiardi, Bregonzio, Juorio and Macova2005; Voigt et al., Reference Voigt, Hörtnagl, Rex, van Hove, Bader and Fink2005; Kangussu et al., Reference Kangussu, Almeida-Santos, Bader, Alenina, Fontes, Santos, Aguiar and Campagnole-Santos2013; Bild and Ciobica, Reference Bild and Ciobica2013; Almeida-Santos et al., Reference Almeida-Santos, Kangussu, Moreira, Santos, Aguiar and Campagnole-Santos2016; Kangussu and Almeida-Santos, Reference Kangussu and Almeida-Santos2017; Chrissobolis et al., Reference Chrissobolis, Luu, Waldschmidt, Yoakum and D’Souza2020; Kangussu, Reference Kangussu2020; Mohite et al., Reference Mohite, Sanches and Teixeira2020).
Alamandine and its receptor, Mas-related G-protein coupled receptor of the type D (MrgD), were identified by our group as new components of the RAS complex system (Lautner et al., Reference Lautner, Villela, Fraga-Silva, Silva, Verano-Braga, Costa-Fraga, Jankowski, Jankowski, Sousa, Alzamora, Soares, Barbosa, Kjeldsen, Oliveira, Braga, Savergnini, Maia, Peluso, Passos-Silva, Ferreira, Alves, Martins, Raizada, Paula, Motta-Santos, Kemplin, Pimenta, Alenina, Sinisterra, Bader, Campagnole-Santos and Santos2013). Alamandine is an endogenous peptide, present also in human blood, that can be generated from Ang-A by ACE2 or from Ang-(1-7) through enzymatic decarboxylation of the aspartic acid at position 1 (Jankowski et al., Reference Jankowski, Vanholder, van der Giet, Tölle, Karadogan, Gobom, Furkert, Oksche, Krause, Tran, Tepel, Schuchardt, Schlüter, Wiedon, Beyermann, Bader, Todiras, Zidek and Jankowski2007; Lautner et al., Reference Lautner, Villela, Fraga-Silva, Silva, Verano-Braga, Costa-Fraga, Jankowski, Jankowski, Sousa, Alzamora, Soares, Barbosa, Kjeldsen, Oliveira, Braga, Savergnini, Maia, Peluso, Passos-Silva, Ferreira, Alves, Martins, Raizada, Paula, Motta-Santos, Kemplin, Pimenta, Alenina, Sinisterra, Bader, Campagnole-Santos and Santos2013). Alamandine selectively interacts with MrgD (Lautner et al., Reference Lautner, Villela, Fraga-Silva, Silva, Verano-Braga, Costa-Fraga, Jankowski, Jankowski, Sousa, Alzamora, Soares, Barbosa, Kjeldsen, Oliveira, Braga, Savergnini, Maia, Peluso, Passos-Silva, Ferreira, Alves, Martins, Raizada, Paula, Motta-Santos, Kemplin, Pimenta, Alenina, Sinisterra, Bader, Campagnole-Santos and Santos2013) which is widely expressed in many organs (Santos et al., Reference Santos, Oudit, Verano-Braga, Canta, Steckelings and Bader2019), including different brain areas (Oliveira et al., Reference Oliveira, Peluso, Qadri, Alenina, Bader and Santos2015; Marins et al., Reference Marins, Oliveira, Qadri, Motta-Santos, Alenina, Bader, Fontes and Santos2021).
In the brain, alamandine produces a pressor effect when microinjected into the rostral ventrolateral medulla, and a depressor effect when microinjected into the caudal ventrolateral medulla (Lautner et al., Reference Lautner, Villela, Fraga-Silva, Silva, Verano-Braga, Costa-Fraga, Jankowski, Jankowski, Sousa, Alzamora, Soares, Barbosa, Kjeldsen, Oliveira, Braga, Savergnini, Maia, Peluso, Passos-Silva, Ferreira, Alves, Martins, Raizada, Paula, Motta-Santos, Kemplin, Pimenta, Alenina, Sinisterra, Bader, Campagnole-Santos and Santos2013; Soares et al., Reference Soares, Barbosa, Campagnole-Santos, Santos and Alzamora2017). Shen et al. (Reference Shen, Chen, Yang, Liu and Li2018) showed that microinjection of alamandine into the hypothalamic paraventricular nucleus (PVN) increases blood pressure and sympathetic outflow via MrgD and cyclic adenosine monophosphate (cAMP)-protein kinase A (PKA) pathway. Additionally, Gong et al. (Reference Gong, Shen, Li, Zhao, Chen, Li, Sheng, Zhou and Kong2019) demonstrated that superoxide anions mediate the increased blood pressure and enhanced sympathetic activity observed after a microinjection of alamandine into the PVN in both normotensive and hypertensive rats. More recently, Marins et al. (Reference Marins, Oliveira, Qadri, Motta-Santos, Alenina, Bader, Fontes and Santos2021) revealed that microinjection of alamandine produces an increase in mean arterial blood pressure (MAP), heart rate (HR) and renal sympathetic nerve activity in rostral insular cortex (rIC). Alamandine effects were not altered by A779, a Mas antagonist, but were completely blocked by the D-Pro7-Ang-(1–7), an antagonist of both, Mas and MrgD.
Our group was the first to report that alamandine, through MrgD, induces antidepressant-like effects in transgenic rats with low brain angiotensinogen (Almeida-Santos et al., Reference Almeida-Santos, de Melo, Gonçalves, Oliveira Amaral, Santos, Campagnole-Santos and Kangussu2021). Moreover, we and others have shown that Ang-(1-7) exerts antidepressant effects in different rat strains (Kangussu et al., Reference Kangussu, Almeida-Santos, Bader, Alenina, Fontes, Santos, Aguiar and Campagnole-Santos2013; Bild and Ciobica, Reference Bild and Ciobica2013; Almeida-Santos et al., Reference Almeida-Santos, Kangussu, Moreira, Santos, Aguiar and Campagnole-Santos2016). Nevertheless, although Walther et al. (Reference Walther, Balschun, Voigt, Fink, Zuschratter, Birchmeier, Ganten and Bader1998) have shown that Mas KO mice present anxiety, no study has yet evaluated whether these animals exhibit depressive-like behaviour. In the present study, we investigated the impact of genetic deletion of MrgD or Mas on depressive-like behaviour in male mice.
Materials and methods
Animals and environment conditions
Adult (8–12 weeks of age) MrgD (Zylka et al., Reference Zylka, Rice and Anderson2005) and Mas knockout (Walther et al., Reference Walther, Balschun, Voigt, Fink, Zuschratter, Birchmeier, Ganten and Bader1998) (MrgD KO and Mas KO) male mice and their controls C57BL/6J (WT) were used. All animals were kept in an environment with controlled temperature (21–23°C) and humidity (50–60%) under a light–dark cycle of 12–12 h. All behavioural experiments were performed during the light phase, between 8:00 a.m. and 11:30 a.m. Mice were kept in groups (4–5 animals) in a polypropylene cage (28 × 17 × 12 cm3). Food and water were available ad libitum. The protocols were approved by the Institutional Ethics Committee on the Use of Animals at the Universidade Federal de Minas Gerais (CEUA/UFMG) (no. 223/2020). All experiments were performed in accordance with the guidelines from the National Council for Animal Experimentation Control (CONCEA-BRAZIL). This legislation abides by the ARRIVE guidelines.
Drugs
[D-Ala7]-Ang-(1-7) (A779; Bachem, Switzerland), a selective Mas antagonist, was diluted in sterile saline at a concentration of 10 mg/mL. Aliquots of 10 µL were made and stored at −20°C until use. Each aliquot was diluted at a desired concentration at the moment of experiment and used only once.
Intracerebroventricular (icv) injection
In order to implant a single cannula into the right lateral ventricle, mice were anesthetised with ketamine–xylazine solution (80 mg/kg and 10 mg/kg, respectively; intraperitoneally) and positioned in a stereotaxic apparatus. For icv injection, a metallic cannula (guide cannula) was implanted into the right lateral ventricle (from the bregma: anteroposterior (AP) = − 0.4 mm, mediolateral (ML) = 1 mm, and dorsoventral (DV) = −2 mm) and cemented with two anchoring screws to the skull. An obturator was placed inside the guide cannula to prevent obstruction. At the end of the surgery, mice received a single intramuscular injection of Flunixin meglumine (Banamine; 0.3 mg/kg) and pentabiotic (160 mg/kg; Fort Dodge Animal Health, Brazil) and were left to recover for at least 5 days.
Icv injection was performed by gently replacing the obturator with an injector needle 0.5 mm longer than the guide cannula. A779 (0.5 μg) was injected in a volume of 0.5 µL during 1 min period with a microsyringe (Hamilton®; Reno, NV, USA) coupled to an infusion pump (Insight®, SP, Brazil). The guide cannula was connected to the microsyringe with a polyethylene catheter. In order to prevent reflux, the needle was kept in place for at least 30 s following the end of the injection. Behavioural tests were performed 10 min after icv injection. Immediately after the behavioural tests, mice were deeply anesthetised with urethane (2 g/kg) and were subjected to an icv injection of Evans blue dye (5%; 0.5 µL). Afterwards, brains were removed and sectioned. The presence of staining only in the ventricular system was used to confirm the position of the cannula in the lateral ventricle. This material was not used for biochemistry analysis.
Forced swimming test (FST)
FST is a useful tool to access the depressive-like behaviour in mice and was carried out as described by Porsolt et al. (Reference Porsolt, Le Pichon and Jalfre1977). MrgD KO, Mas KO, and WT experimentally naive mice were subjected to a 6-min session of FST, where the immobility time was measured during the last 4 min. The FST was performed in a glass cylinder (29 cm height × 18 cm diameter) filled until 20 cm height with water at 25°C. The sessions were recorded and the total amount of time the animals remained immobile (immobility time), except for small limb movements necessary for floatation, were scored manually by an observer that was unaware of animals or treatments (double blind). Moreover, latency to first immobility was also evaluated with a minimal duration of 2 s. This evaluation started immediately after placing animals in the cylinder (Castagné et al., Reference Castagné, Porsolt and Moser2009; Chen et al., Reference Chen, Faas, Ferando and Mody2015). The water was changed after each trial to avoid the influence of alarm substances.
Tail suspension test (TST)
TST is based on the analysis of immobility induced by suspension posture as a measure to access the depressive-like behaviour (Steru et al., Reference Steru, Chermat, Thierry and Simon1985). Mice were individually taped by the tail to a metal rod (50 cm height) and allowed to hang upside down for 6 min. The immobility time (absence of any body movements not related to respiration) was quantified (in seconds) by an experimenter that was blind to the mice phenotype. After the test, the tape was gently removed and mice were replaced in their cages.
Open field test (OFT)
Naive animals had their spontaneous locomotor activity monitored in an open field apparatus (LE 8811 IR Motor Activity Monitors PANLAB, Harvard Apparatus; Spain), with acrylic box dimension of 450 × 450 × 200 mm (width × depth × height). The total distance travelled during 5 min was monitored automatically using the ACTITRACK software (Actitrackv 2.7.13). After the end of each animal test, the apparatus was cleaned with 70% ethanol.
Enzyme-linked immunosorbent assay (ELISA)
ELISA was performed to quantify brain-derived neurotrophic factor (BDNF) protein levels in prefrontal cortex (PFC) and hippocampus (HP). Five days after OFT, animals were killed and brain regions homogenates from both hemispheres were obtained using an extraction solution (100 mg of tissue per millilitre), containing 0.4 M NaCl, 0.05% Tween 20, 0.5% BSA, 0.1 mm phenyl methyl sulphonyl fluoride, 0.1 mm benzethonium chloride, 10 mm EDTA, and 20 KIU aprotinin, using Ultra-Turrax. Lysates were centrifuged at 13,000 g for 10 min at 4°C, and supernatants were collected. Concentrations of BDNF from the supernatants were determined in an ELISA (MyBioSourse – MBS2700738) setup, according to the manufacturer’s procedures, in duplicates. The data were expressed as picogram per 100 mg of tissue.
Statistical analysis
Data were expressed as mean ± SEM and the level of significance was set at p < 0.05. Differences between groups were assessed by either unpaired Student’s t test, one- or two-way analysis of variance (ANOVA) followed by Bonferroni post-test, as denoted in results and figure legends. Graphics and analysis were performed using GraphPad Prism software (version 7.0, La Jolla, CA, USA).
Results
As can be observed in Fig. 1, there was no significant difference in immobility time between MrgD KO and WT mice in FST [WT: 102.5 ± 8.5 s, n = 8; MrgD KO: 86.5 ± 8.6 s, n = 8], and TST [WT: 121.9 ± 7.8 s, n = 8; MrgD KO: 114.1 ± 7.1 s, n = 8]. There was also no difference in latency to first immobility [WT: 52.2 ± 1.4 s, n = 16; MrgD KO: 49.1 ± 2.2 s, n = 8] in FST. In order to investigate whether Mas conceals an effect of MrgD deficiency on depression-like behaviour, MrgD KO and WT mice received acute icv injection of the Mas antagonist, A779. Interestingly, as shown in Fig. 2, A779 treated MrgD KO mice presented an increase of immobility time in FST [genotype factor F (1,18) = 34.14, p < 0.0001; drug factor F (1,18) = 38.24, p < 0.0001; interaction genotype × drug F (1,18) = 25.48, p < 0.0001; d = 2.339; two-way ANOVA] and an increase of immobility time in TST [genotype factor F (1,21) = 9,468, p = 0.0057; drug factor F (1,21) = 22.78, p = 0.0001; interaction genotype × drug F (1,21) = 11.18, p = 0.0031; two-way ANOVA]. These results suggest that blockade of Mas can reveal a depressive-like behaviour phenotype in MrgD KO mice. A779 did not change immobility in both FST or TST in WT mice (Fig. 2) indicating that acute Mas blockade does not induce depressive-like behaviour in mice.

Fig. 1. Immobility time in forced swimming (A) and tail suspension (B) tests. MrgD KO did not exhibit changes in immobility time in both tests in comparison to WT mice (Student t test). Bars represent the mean ± SEM. n = 8 per group.

Fig. 2. Acute intracerebroventricular (icv) injection of A779 (0.5 μg), a selective Mas antagonist, caused increase in immobility time in both forced swimming (A) and tail suspension (B) tests in MrgD KO mice. Bars represent the mean ± SEM. n = 5/7 per group. * p < 0.05 in comparison to WT saline, # p < 0.05 in comparison to MrgD KO mice and † p < 0.05 in comparison to WT A779 group (two-way ANOVA followed by the Bonferroni post-test).
In order to assess the role of chronic Mas ablation in depression behaviour, we performed FST and TST in Mas KO mice. Indeed, as shown in Fig. 3, Mas KO animals showed a significant increased immobility time in both FST [WT: 103.5 ± 6.2 s, n = 8; Mas KO: 159.8 ± 9.6 s, n = 6] and TST [WT: 144.3 ± 10.6 s, n = 8; Mas KO: 196.8 ± 10.8 s, n = 8], as well as in latency to first immobility [WT: 52.2 ± 1.4 s, n = 16; Mas KO: 36.2 ± 2.1 s, n = 6] in FST. This result reinforces the involvement of Mas receptor in neurobiology of depression. Moreover, to verify possible changes in the locomotor activity of MrgD KO and Mas KO mice, the distance crossed in the OFT was measured and no significant differences were observed, suggesting that these animals had no limitation in their motor system (Fig. 4).

Fig. 3. Mas KO mice exhibit increased immobility time in both forced swimming (A) and tail suspension (B) tests compared to WT mice. Bars represent the mean ± SEM. n = 6/8 per group. * p < 0.05 in comparison to WT group (Student t test).
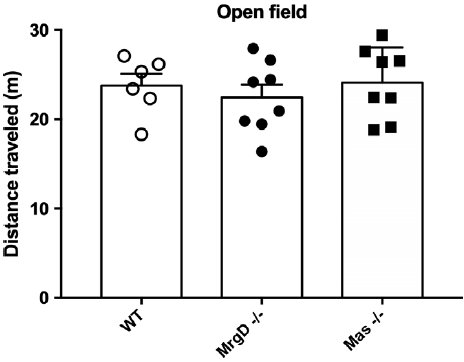
Fig. 4. Locomotor activity was not altered in either MrgD KO and Mas KO groups subjected to open field test, compared to WT group (One-way ANOVA). Bars represent the mean ± SEM. n = 6/8 per group.
Aiming to explore possible mechanisms in the increased depressive-like behaviour of Mas KO mice, we investigated whether there were alterations in cerebral BDNF levels in two key regions related to depression, that is, PFC and HP. As shown in Fig. 5, BDNF level in PFC and HP was decreased in Mas KO mice [PFC: 104 ± 4.6 pg/100 mg tissue vs 148 ± 8 pg/100 mg tissue, n = 6; HP: 219 ± 12 pg/100 mg tissue vs 287 ± 17.4 pg/100 mg tissue, n = 5-6]. However, BDNF was not altered in mice lacking MrgD (Fig. 5).

Fig. 5. Brain-derived neurotrophic factor (BDNF) levels in prefrontal cortex (A, pg/100 mg of tissue) and hippocampus (B, pg/100 mg of tissue) were decreased in Mas KO mice but not in MrgD KO mice compared to WT group. Bars represent the mean ± SEM. n = 5/6 per group. * p < 0.05 in comparison to WT, # p < 0.05 in comparison to MrgD KO mice (one-way ANOVA followed by the Bonferroni post-test).
Discussion
The main finding of the present study, contrary to what was expected, is that MrgD KO mice do not show depressive-like behaviour. Nevertheless, acute icv injection of a Mas antagonist induced a depressive phenotype in MrgD KO mice. In keeping, Mas KO mice presented depression-like behaviour. Furthermore, corroborating our findings, Mas KO mice presented a reduction of BDNF in PFC and HP, while in MrgD KO mice BDNF levels were not altered.
In retrospect, brain was the first organ in which Mas was found to be highly expressed (Young et al., Reference Young, O’Neill, Jessell and Wigler1988). Santos et al. (Reference Santos, Campagnole-Santos, Baracho, Fontes, Silva, Neves, Oliveira, Caligiorne, Rodrigues and Gropen Júnior1994) described A779 as a selective Ang-(1–7) antagonist, and in 1998 Walther et al. reported that mice lacking the Mas show anxiety-like behaviour assessed in the elevated-plus maze (Walther et al., Reference Walther, Balschun, Voigt, Fink, Zuschratter, Birchmeier, Ganten and Bader1998). Later, in 2003, our group identified Mas as a functional selective receptor for Ang-(1-7). More recently, our group has demonstrated that icv injection of Ang-(1–7) reversed the anxiety-depressive like state in transgenic rats with low brain angiotensinogen and in transgenic hypertensive (mRen2)27 rats (Kangussu et al., Reference Kangussu, Almeida-Santos, Bader, Alenina, Fontes, Santos, Aguiar and Campagnole-Santos2013; Almeida-Santos et al., Reference Almeida-Santos, Kangussu, Moreira, Santos, Aguiar and Campagnole-Santos2016). Besides, Mas is essential for anxiolytic-like effect induced by Ang-(1-7) (Kangussu and Almeida-Santos, Reference Kangussu and Almeida-Santos2017), and the present study is the first to show that Mas KO mice present depressive phenotype.
Previous studies using animals with genetic deletion of Mas described several alterations, including cardiovascular, metabolic, behavioural, among others. Lack of Mas results in impairment of in vitro and in vivo heart function (Santos et al., Reference Santos, Castro, Gava, Pinheiro, Almeida, Paula, Cruz, Ramos, Rosa, Irigoyen, Bader, Alenina, Kitten and Ferreira2006), increased blood pressure, endothelial dysfunction, and an imbalance between nitric oxide and reactive oxygen species (Peiró et al., Reference Peiró, Vallejo, Gembardt, Azcutia, Heringer-Walther, Rodríguez-Mañas, Schultheiss, Sánchez-Ferrer and Walther2007; Xu et al., Reference Xu, Costa-Goncalves, Todiras, Rabelo, Sampaio, Moura, Santos, Luft, Bader, Gross, Alenina and Santos2008), besides an important imbalance in the neural control of blood pressure, altering not only the baroreflex but also the chemo- and Bezold–Jarisch reflexes (de Moura et al., Reference de Moura, dos Santos, Campagnole-Santos, Todiras, Bader, Alenina and Haibara2010).
Mas deficiency in mice leads to dramatic changes in glucose and lipid metabolisms, inducing a metabolic syndrome-like state (Santos et al., Reference Santos, Fernandes, Mario, Ferreira, Pôrto, Alvarez-Leite, Botion, Bader, Alenina and Santos2008) and it is associated with worsening of lipid profile and severe hepatic steatosis in ApoE-knockout mice (Silva et al., Reference Silva, Aguilar, Alvarez-Leite, da Silva, Arantes, Bader, Alenina, Pelli, Lenglet, Galan, Montecucco, Mach, Santos and Santos2013). Furthermore, Mas deletion increased contextual fear memory and slowed down its extinction (Lazaroni et al., Reference Lazaroni, Bastos, Moraes, Santos and Pereira2016). These studies suggested that Mas KO mice may be useful to evaluate the neurobiology of post-traumatic stress disorders.
Resembling the ACE2/Ang-(1-7)/Mas receptor axis, Alamandine through MrgD has shown important protective functions in cardiovascular regulation and behaviour (Passos-Silva et al., Reference Passos-Silva, Brandan and Santos2015; Santos et al., Reference Santos, Oudit, Verano-Braga, Canta, Steckelings and Bader2019; Almeida-Santos et al., Reference Almeida-Santos, de Melo, Gonçalves, Oliveira Amaral, Santos, Campagnole-Santos and Kangussu2021). MrgD was found in several areas of the mouse brain (Oliveira et al., Reference Oliveira, Peluso, Qadri, Alenina, Bader and Santos2015; Hami et al., Reference Hami, von Bohlen Und Halbach, Tetzner, Walther and von Bohlen Und Halbach2021), such as cortex, insular cortex, hippocampus, amygdala, hypothalamus, habenular nuclei, striatum and pallidum, trigeminal tract, cerebellum, and in some nuclei of the brainstem.
We have previously shown that Alamandine acting through MrgD was able to induce antidepressant-like effect in transgenic rats with low brain angiotensinogen (Almeida-Santos et al., Reference Almeida-Santos, de Melo, Gonçalves, Oliveira Amaral, Santos, Campagnole-Santos and Kangussu2021). However, in this study, we did not observe changes in depression-related behaviour in mice with genetic deletion of MrgD, both in FST and TST. The first hypothesis raised was a possible compensatory activity of Mas in this effect. To test it, we performed acute Mas blockade in MrgD KO mice. Confirming our thesis, after acute icv injection of A779, a Mas antagonist, animals lacking MrgD showed depressive-like behaviour.
In order to advance into possible mechanisms underlying stress-coping behaviour of these animals, we evaluated BDNF levels. BDNF plays several functions in CNS, with critical roles in neuronal development, differentiation and survival, dendritic arborisation, synaptogenesis, and synaptic plasticity – for reviews, see Huang and Reichardt (Reference Huang and Reichardt2001), Greenberg et al. (Reference Greenberg, Xu, Lu and Hempstead2009) and Lu et al. (Reference Lu, Pang and Woo2005). Many studies have demonstrated that BDNF is decreased in depression (Eisch et al., Reference Eisch, Bolaños, de Wit, Simonak, Pudiak, Barrot, Verhaagen and Nestler2003; Martinowich et al., Reference Martinowich, Manji and Lu2007; Björkholm and Monteggia, Reference Björkholm and Monteggia2016; Yang et al., Reference Yang, Nie, Shu, Kuang, Chen, Cheng, Yu and Liu2020). Ang-(1-7) increased BDNF production in the 6-hydroxydopamine model of Parkinson’s disease (Rabie et al., Reference Rabie, Abd El Fattah, Nassar, El-Abhar and Abdallah2018) and, in agreement, ACE2 KO mice present lower mRNA and protein levels of BDNF (Wang et al., Reference Wang, Iwanami, Min, Tsukuda, Nakaoka, Bai, Shan, Kan-No, Kukida, Chisaka, Yamauchi, Higaki, Mogi and Horiuchi2016). Corroborating the results of behavioural analysis with FST and TST, we observed that lack of Mas resulted in decreased BDNF levels in PFC and HP. On the other hand, MrgD KO mice did not present alterations in BDNF levels.
The limitations of this study should be taken into consideration when discussing our data. For instance, we performed only two behavioural tests, the FST and TST. It would be interesting to confirm our data in different preclinical tests; however, it is important to highlight that FST and TST are highly reproducible and widely used for assessing depressive-like behaviour in rodents (Steru et al., Reference Steru, Chermat, Thierry and Simon1985; Cryan et al., Reference Cryan, Mombereau and Vassout2005; Petit-Demouliere et al., Reference Petit-Demouliere, Chenu and Bourin2005; Kraeuter et al., Reference Kraeuter, Guest and Sarnyai2019). Besides, the present study was performed only in male mice. We are aware of the importance of using female animals or to make gender comparisons, especially related to the response to anxiety/depression-like behaviours. Of note, depression has a higher incidence in women (Kessler, Reference Kessler2003); however, preliminary data from our laboratory showed no difference between males and females neither in WT nor in Mas KO mice submitted to FST. Nevertheless, future studies will have to systematically address these questions. Finally, it is important to mention that recent discussions (Molendijk and de Kloet, Reference Molendijk and de Kloet2015; Gururajan et al., Reference Gururajan, Reif, Cryan and Slattery2019) have questioned the usefulness of experimental models for the study of depression, especially FST, mainly due to the multifactorial nature and heterogeneity of this disorder. We cannot lose sight, however, of the fact that animal models are not, and never were, designed to represent the human condition in its entirety. Thus, the role of these preclinical models in the discovery of new antidepressant drugs is still critical to the development of innovative or fast-acting therapies that provide much-needed relief to patients around the world.
Collectively, our findings indicate that Mas has a pivotal role in the neurobiology of depression. Mas KO male mice presented depressive-like behaviour in FST and TST, besides a reduction of BDNF in PFC and HP. On the other hand, although we previously showed that alamandine induces antidepressant-like effects, mice with genetic deletion of MrgD did not display depressive-like behaviour or altered levels of BNDF in the brain. This phenotype may be explained, at least in part, by a compensatory activity of Mas in MrgD mice. In agreement, Mas blockade revealed a depression-like behaviour in MrgD KO male mice. Finally, our results reinforce the importance of RAS components in pathophysiology of neuropsychiatric disorders.
Author contributions
LB: performed experiments, analysed data, prepared figures, interpreted results of experiments and drafted manuscript; MLAF: analysed data and drafted manuscript; SCAG: performed experiments and analysed data; MB: interpreted results of experiments, edited and revised manuscript; RASS: interpreted results of experiments, edited and revised manuscript; MJCS: analysed data, prepared figures, interpreted results of experiments, drafted manuscript, edited and revised manuscript; LMK: conception and design of research, analysed data, prepared figures, interpreted results of experiments, drafted manuscript, edited and revised manuscript. All authors contributed to and approved the final version of manuscript.
Financial support
This work was supported by Pró-Reitoria de Pesquisa da Universidade Federal de Minas Gerais, Conselho Nacional de Ciência e Tecnologia (CNPq), and Coordenação de Aperfeiçoamento de Pessoal de Nível Superior (CAPES) through PROBRAL-CAPES-DAAD Program (Grant# 57598273 to MB and Grant# 88881.198677/2018-01 to MJC-S).
Conflict of interest
None.