INTRODUCTION
Sports-related concussions are a major public health concern as 1.6 to 3.8 million concussions are estimated to occur each year in the United States only (Langlois, Rutland-Brown, & Wald, Reference Langlois, Rutland-Brown and Wald2006). The Consensus Statement from the 4th International conference on concussion in sport defined a sports-related concussion as a complex pathophysiological process affecting the brain, induced by biomechanical forces applied either directly or indirectly (e.g., neck) to the brain (McCrory et al., Reference McCrory, Meeuwisse, Aubry, Cantu, Dvořák, Echemendia and Raftery2013). Although the physical, emotional, and cognitive symptoms following a concussion usually resolve within 7 to 10 days (McCrory et al., Reference McCrory, Johnston, Meeuwisse, Aubry, Cantu, Dvorak and Schamasch2005), the potentially serious and persistent sequelae to the brain following multiple concussions are now well documented (Bombardier et al., Reference Bombardier, Fann, Temkin, Esselman, Barber and Dikmen2010; Chen, Johnston, Petrides, & Ptito, Reference Chen, Johnston, Petrides and Ptito2008; Guskiewicz et al., Reference Guskiewicz, Marshall, Bailes, McCrea, Harding, Matthews and Cantu2007).
Among others, persistent depression after concussion is associated with higher-order cognitive function impairments (Chen et al., Reference Chen, Johnston, Petrides and Ptito2008; Mainwaring, Hutchison, Comper, Richards, & Trenerry, Reference Mainwaring, Hutchison, Comper, Richards and Trenerry2012). The sports-related concussion literature has highlighted sex differences in the recovery of athletes who sustained a concussion. In addition to the proportionately higher concussion rate in females in sports played by both sexes (Marar, McIlvain, Fields, & Comstock, Reference Marar, McIlvain, Fields and Comstock2012), recent reports suggest that concussed female athletes could be more likely than their male counterparts to experience persistent symptoms, including depression (Broshek et al., Reference Broshek, Kaushik, Freeman, Erlanger, Webbe and Barth2005; Dick, Reference Dick2009; Farace & Alves, Reference Farace and Alves2000; Fenton, McClelland, Montgomery, MacFlynn, & Rutherford, Reference Fenton, McClelland, Montgomery, MacFlynn and Rutherford1993).
In parallel, alterations of the ability to identify and discriminate emotional facial expressions (EFEs) were found among people who sustained a traumatic brain injury tested either during the acute (<3 months) or the chronic post-injury phase (>3 months) (Green Turner, & Thompson, Reference Green, Turner and Thompson2004; Henry, Phillips, Crawford, Ietswaart, & Summers, Reference Henry, Phillips, Crawford, Ietswaart and Summers2006; Léveillé, Guay, Blais, Scherzer, & De Beaumont, Reference Léveillé, Guay, Blais, Scherzer and De Beaumont2016). EFEs are known to be particularly salient stimuli to humans as they are thought of as immediate indicators of affective dispositions in other people (Holmes, Kiss, & Eimer, Reference Holmes, Kiss and Eimer2006). EFE processing alterations following a concussion can result in socioaffective impairments (Carton, Kessler, & Pape, Reference Carton, Kessler and Pape1999), affecting one’s capacity to manage post-concussion symptoms. Post-concussion symptoms management is crucial for athletes as the latter represents a major obstacle keeping them from returning to competition (Moore, Terryberry-Spohr, & Hope, Reference Moore, Terryberry-Spohr and Hope2006; Soo, Tate, & Rapee, Reference Soo, Tate and Rapee2012). Furthermore, sex differences in EFE processing are abundantly documented, as females tend to be more accurate and faster in identifying EFEs than males (Hall & Matsumoto, Reference Hall and Matsumoto2004; Hampson, Vananders, & Mullin, Reference Hampson, Vananders and Mullin2006).
A recent study demonstrated significant sex-related differences on the processing of emotional stimuli after concussion (Léveillé et al., Reference Léveillé, Guay, Blais, Scherzer and De Beaumont2016). Whereas emotion recognition ability was equivalent in female athletes regardless of concussion history, male concussed athletes showed emotion recognition alterations and needed significantly more emotional intensity than unconcussed male counterparts to identify these emotions. To this day, however, very little is known about the neurophysiological underpinnings of this male-specific concussion effect on the processing of emotional stimuli.
Event-related brain potentials (ERPs) were particularly useful to deepen our understanding of the neurophysiological underpinnings of EFE processing. ERPs are changes in the brain electrical activity in response to an event, such as the appearance of a stimulus, or performing a manual response (Hajcak et al., Reference Hajcak, Weinberg, MacNamara, Foti, Luck and Kappenman2012). ERP components are labeled after their polarity (P for positive and N for negative) and latency. Studies have shown that when faces are attended, emotional processing elicits a greater frontal positivity, starting approximately 100 ms after stimulus onset, suggesting that cortical circuits involved in the detection of emotionally significant events can be triggered rapidly by EFE (Halgren, Baudena, Heit, Clarke, & Marinkovic, Reference Halgren, Baudena, Heit, Clarke and Marinkovic1994; Streit et al., Reference Streit, Ioannides, Liu, Wölwer, Dammers, Gross and Müller-Gärtner1999). Another study suggested that emotional stimuli are detected pre-attentively, and may capture attention automatically, irrespective of the current focus of spatial attention (Holmes, Vuilleumier, & Eimer, Reference Holmes, Vuilleumier and Eimer2003). It is, therefore, not surprising to note that ERP components related to early visual perception and attention were shown to be involved in EFE processing such as the P1 and the N1 waveform components, which are two attention-related components.
The P1 component is the first positive-going component peaking at around 100–130 ms after the onset of a visual stimulus over occipito-temporal sites (Meeren, van Heijnsbergen, & de Gelder, Reference Meeren, van Heijnsbergen and de Gelder2005). The P1 component is associated with the processing of low-level properties of any type of attended visual stimulus, namely visuospatial processing (Hopf & Mangun, Reference Hopf and Mangun2000). This attentional component may index early stages of face processing as its amplitude is influenced by emotional stimuli (Batty & Taylor, Reference Batty and Taylor2003; Halit, de Haan, & Johnson, Reference Halit, de Haan and Johnson2000; Itier & Taylor, Reference Itier and Taylor2002). This suggests that cortical circuits involved in the detection of configural aspects of emotional facial stimuli can be activated at an early stage of visual processing (Olivares, Iglesias, Saavedra, Trujillo-Barreto, & Valdes-Sosa, Reference Olivares, Iglesias, Saavedra, Trujillo-Barreto and Valdes-Sosa2015). ERPs studies have also demonstrated asymmetries in EFE processing, with a maximal P1 amplitude peaking over the right parietal and occipito-temporal regions (Batty & Taylor, Reference Batty and Taylor2003; Itier & Taylor, Reference Itier and Taylor2002; Itier & Taylor, Reference Itier and Taylor2004; Proverbio, Brignone, Matarazzo, Del Zotto, & Zani, Reference Proverbio, Brignone, Matarazzo, Del Zotto and Zani2006; Rossion et al., Reference Rossion, Delvenne, Debatisse, Goffaux, Bruyer, Crommelinck and Guérit1999).
The visual N1 component is the first negative-going deflection peaking at around 150–200 ms after stimulus onset. The anterior N1 waveform is elicited when visual stimuli are presented in attended locations, especially during a discrimination task. Most of the literature on ERPs and EFE has looked at the modulation of the anterior N1 response (Bar-Haim, Lamy, & Glickman, Reference Bar-Haim, Lamy and Glickman2005; Holmes et al., Reference Holmes, Vuilleumier and Eimer2003; Luo, Feng, He, Wang, & Luo, Reference Luo, Feng, He, Wang and Luo2010). N1 amplitude is enhanced by emotional content of visual stimuli for both aversive and pleasant content, compared to neutral stimuli (Luck & Kappenman, Reference Luck and Kappenman2011). However, ERP studies of emotional facial expression have demonstrated that this effect is suppressed when visual stimuli are presented unexpectedly to participants, suggesting that attention plays an important role in emotional processing of facial expressions (Holmes et al., Reference Holmes, Vuilleumier and Eimer2003; Holmes, Winston, & Eimer, Reference Holmes, Winston and Eimer2005).
ERPs were also shown to be highly sensitive to the long-term effects of concussion on cognition (De Beaumont, Brisson, Lassonde, & Jolicoeur, Reference De Beaumont, Brisson, Lassonde and Jolicoeur2007; Gosselin et al., Reference Gosselin, Saluja, Chen, Bottari, Johnston and Ptito2010; Gosselin, Thériault, Leclerc, Montplaisir, & Lassonde, Reference Gosselin, Thériault, Leclerc, Montplaisir and Lassonde2006; Thériault, De Beaumont, Gosselin, Filipinni, & Lassonde, Reference Thériault, De Beaumont, Gosselin, Filipinni and Lassonde2009). Persistent alterations of ERP waveforms such as the P3, the sustained posterior contralateral negativity (SPCN) and event-related negativity (ERN) were documented after concussion despite the resolution of post-concussion symptoms and normal performance on neuropsychological tests (Gaetz, Goodman, & Weinberg, Reference Gaetz, Goodman and Weinberg2000; Thériault et al., Reference Thériault, De Beaumont, Gosselin, Filipinni and Lassonde2009; Theriault, De Beaumont, Tremblay, Lassonde, & Jolicoeur, Reference Theriault, De Beaumont, Tremblay, Lassonde and Jolicoeur2011).
Furthermore, ERPs have also been particularly useful in documenting the cumulative effects of recurrent concussions (i.e., concussion effects on ERP are accentuated as a function of the number of concussions sustained) (De Beaumont et al., Reference De Beaumont, Brisson, Lassonde and Jolicoeur2007; Gaetz et al., Reference Gaetz, Goodman and Weinberg2000; Thériault et al., Reference Thériault, De Beaumont, Gosselin, Filipinni and Lassonde2009). Moreover, a study conducted among elite symptomatic and asymptomatic athletes revealed that having sustained multiple concussions induces significant N1 amplitude reduction when performing an auditory attention task (Gosselin et al., Reference Gosselin, Thériault, Leclerc, Montplaisir and Lassonde2006). More recently, two studies revealed a decrease in early sensory processing in concussed athletes. These studies highlighted a reduction in N1 as well as in P1 amplitude during a visual task relative to sub-concussed or control athletes (Moore, Broglio, & Hillman, Reference Moore, Broglio and Hillman2014; Moore, Lepine, & Ellemberg, Reference Moore, Lepine and Ellemberg2017). Taken together, these results suggest that concussions could affect early evoked potentials. To our knowledge, very few, if any, studies have investigated early electrophysiological components of visual perception and attention on EFE in concussed athletes.
Here, we investigated the cumulative and long-term effects of concussions on early ERP measures (P1 and N1) of EFE processing in asymptomatic, multi-concussed athletes during an EFE identification task. We hypothesized that concussed athletes would show alterations in early ERP measures (P1 and N1). Another objective was to study sex differences on P1 and N1 components in an EFE identification task in multi-concussed and control athletes. Considering the known sex-related differences in concussion outcome and recovery coupled with emerging data on sex-related differences in emotion recognition in multi-concussed athletes, we hypothesized that concussion effects on P1 and N1 components would be significantly modulated by sex. We also hypothesized that females, whether concussed or not, would outperform males on emotional facial recognition accuracy.
METHODS
Participants
All 83 participants were active male and female athletes from Canadian universities and collegiate sports organizations who were recruited with the collaboration of team coaches. Participants were included if they met all of the following criteria: no history of alcohol and/or substance abuse; no medical condition requiring daily medication; no previous history of psychiatric illness, affective disorder, learning disability, neurological history (brain tumor, seizure, central nervous system neoplasm), or traumatic brain injury unrelated to contact sports. None of them had suffered a concussion within the 3 months preceding testing and they were all asymptomatic on the Post-Concussion Symptoms Scale (PCSS). The study was approved by the local ethics committee and all participants provided written informed consent before testing. Volunteers received a financial compensation of $50 CDN for their participation.
The two concussion groups included 22 female and 21 male athletes who reported having sustained at least two sports-related concussions that occurred at least 3 months before testing. The number of reported concussions sustained ranged from two to eight (M=3.04 and SD=1.29). Information on concussions was self-reported, using a standardized concussion history questionnaire (Hecht & Kent, Reference Hecht and Kent2005). Concussion classification was based on the definition provided by the Consensus Statement of the 4th International Conference on concussion in sport (McCrory et al., Reference McCrory, Meeuwisse, Aubry, Cantu, Dvořák, Echemendia and Raftery2013). All brain injuries were classified as “mild” on the Glasgow Coma Scale (GCS; scores ranging from 13 to 15). At the time of testing, concussed athletes were asymptomatic, reporting few (if any) symptoms on the PCSS. The control groups consisted of 20 male and 20 female athletes who presented with no prior history of head injury, sports-related or otherwise.
Table 1 shows demographic as well as clinical information on the four groups included in the present study. Two-way independent ANOVAs with Group (concussed or not) and Sex (male or female) as between-groups factors were conducted to verify potential differences on demographic variables. There was a statistically significant Group * Sex interaction for age (F(1,79)=4.00; p=.05; η p 2=.048) and post hoc analyses revealed that concussed female athletes were significantly older than female control athletes (p=.02) and male control athletes (p=.05). There was no statistically significant interaction for Education level (F(1,79)=4.00; p=.09), but we found a significant main effect of sex (F(1,79)=4.28; p=.04; η p 2=.051), indicating a higher level of education in females (M=14.79; SD=2.44) than males (M=13.80; SD=1.72). As for the BDI scores, there was no statistically significant interaction (F(1,79)=0.84; p=.36), but we found a significant main effect of Group (F(1,79)=12.56; p=.001, η p 2=.137), where concussed athletes reported more depressive symptoms (M=6.09; SD=4.95) than control athletes (M=2.98; SD=2.57).
Table 1 Demographic and clinical information across groups

Finally, there was a statistically significant interaction for the BAI, (F(1,79)=6.48; p=.01; η p 2=.076) and post hoc tests revealed that control male athletes scored significantly lower than either concussed male athletes (p=.005) or control female athletes (p=.01). Independent t tests computed on clinical variables revealed that concussed females sustained more concussions on average than concussed males (t(41)=-2.22; p=.03; d =.68), but did not differ both in terms of the time elapsed since the last concussion (t(41)=0.15; p=.88) and on the PCSS (t(41)=0.69; p=.49).
EFE Identification Task
A computerized identification task was used to assess EFE recognition. The stimuli used in this study were taken from recently published work from our group (D’Hondt et al., Reference D’Hondt, Lassonde, Thebault-Dagher, Bernier, Gravel, Vannasing and Beauchamp2017). EFE stimuli consisted in photographs of 10 female and 10 male faces (angry, neutral, and happy). All photographs were selected from the NimStim Set of Facial Expressions (Rigoulot, D’Hondt, Honoré, & Sequeira, Reference Rigoulot, D’Hondt, Honoré and Sequeira2012; Tottenham et al., Reference Tottenham, Tanaka, Leon, McCarry, Nurse, Hare and Nelson2009), which is a standardized database of photographs of professional actors portraying various emotional expressions. Extrafacial characteristics were retained, but were standardized so that all three facial expressions portrayed by the same face differed only in terms of the emotion displayed, thus giving us more naturalistic stimuli (Refer to D’Hondt et al., Reference D’Hondt, Lassonde, Thebault-Dagher, Bernier, Gravel, Vannasing and Beauchamp2017, for a detailed description of the stimuli).
During each trial, a central fixation cross was presented for a random duration of 500 to 1500 ms, alerting the participant of the imminent presentation of the picture, which was projected for 500 ms. To control for habituation effects, the interval between two successive stimuli varied randomly between 1.5 and 2.5 s. The task included five blocks of 60 images (20 angry, 20 neutral, and 20 happy) that were always presented centrally in pseudo-random order so that no more than three successive pictures with the same emotion could be displayed (Refer to Supplementary material available online for examples of stimuli and the time course of trials).
Participants were asked to respond as fast as possible to the emotional facial expression depicted by the image by pressing a corresponding right-hand key with the EFE shown (for angry, for neutral, for happy). Reaction time (RT) was defined as the time interval between stimulus onset and the corresponding key press. Two main behavior measures were collected: RTs, which were only computed for correctly identified stimulus, and accuracy scores.
Procedure
The experiment consisted of a single 2.5-hr testing session and included the administration of a general health questionnaire, a concussion history questionnaire, the PCSS, the Beck Depression Inventory (BDI-II), the Beck Anxiety Inventory (BAI), and the computerized EFE identification task. Participants were sitting on a straight-back chair and performed the EFE identification task running with Presentation V13 (Neurobehavioral Systems) while EEG was recorded from 64 electrodes.
Electrophysiological Data Acquisition
The EEG was recorded from 64 active Ag/AgCl electrodes (ActiCAP, Brain Products) positioned according to the standard 10–10 system, with the exception of electrodes TP9 and TP10, which were replaced by reference electrodes placed at the mastoids. EEG data were re-referenced offline to the algebraic average of the left and right mastoids (Handy, Reference Handy2005; Luck, Reference Luck2005). Additional cutaneous electrodes were used to monitor electrooculographic activity; two placed on external canthi to record the horizontal electrooculogram (HEOG), and two placed on infra/supraorbital regions to record the vertical electrooculogram (VEOG). All electrode impedances were kept below 15 kΩ. The EEG was digitized at 500 Hz, high-pass filtered at 0.01 Hz and low-pass filtered at 225 Hz during the recording.
ERP Analysis
Using the software BrainVision Analyzer 2.0 (Brain Products, Germany), EEG signals were high-pass filtered at 0.1 Hz and low-passed at 20 Hz offline. Trials with eye blinks (VEOG>100 µV), large horizontal eye movements (HEOG>35 µV), and/or artifacts (>80 µV) at 1 of the 62 recording electrodes (64 electrodes with the exception of the two reference electrodes) were excluded from further analyses using an automated screening procedure. The EEG was segmented relative to the emotion expressed by the faces and the onset of the stimulus in each task to create stimulus-locked epochs of 1200 ms that included a 200 ms pre-stimulus period. Epochs were baseline corrected relative to the mean signal amplitude between -200 and 0 ms before stimulus presentation. Separate averaged waveforms were computed for each correctly identified EFE (neutral, happy, and angry).
Early components (P1 and N1) were measured at electrodes where brain activity was maximal in each hemisphere, as determined with the use of grand-averaged waveforms and scalp topographies distribution of brain activity for each of these components. As recommended in international ERP guidelines, latencies were taken at the electrode where the amplitude was maximal over each hemisphere, and the amplitude was measured at the determined latency on other electrodes over that hemisphere (Picton et al., Reference Picton, Bentin, Berg, Donchin, Hillyard, Johnson and Rugg2000). P1 was quantified as the mean amplitude recorded at lateral posterior electrodes PO7 and PO8, between 85 and 120 ms post-stimulus onset. N1 was measured at Fz between 85 and 95 ms post-stimulus onset. These time windows and electrodes for each waveform component of interest were consistent with those reported in the literature (Bar-Haim et al., Reference Bar-Haim, Lamy and Glickman2005; Itier & Taylor, Reference Itier and Taylor2002; Stekelenburg & de Gelder, Reference Stekelenburg and de Gelder2004; Utama, Takemoto, Koike, & Nakamura, Reference Utama, Takemoto, Koike and Nakamura2009).
Statistical Analyses
Statistical analyses were conducted using IBM SPSS Statistics for Windows, Version 25.0 (IBM, United States). Given the statistically significant differences in BDI and BAI scores and its potential effects on the results and their interpretation, mixed factorial analyses of covariance (ANCOVAs) were conducted on behavioral data (reaction times and accuracy) and EEG data (P1 and N1 components). ANCOVAs on behavioral data and the N1 component were computed with all three types of EFE (neutral, happy, angry) as within-subject factor, with Sex (male or female) and Group (concussed or not) as between-groups factors, and BDI and BAI as covariates. The ANCOVA on the P1 component was computed with the same factors and covariates, but Lateralization (PO7 and PO8) was also added as a within-subject factor.
Given that both anxiety and depressive symptoms have been found to modulate responses to EFE-like stimuli (Demenescu, Kortekaas, den Boer, & Aleman, Reference Demenescu, Kortekaas, den Boer and Aleman2010), we included these variables as covariates in the proposed ANCOVA model. It is important, however, to bear in mind that mean BDI and BAI scores for each group were far from reaching the established clinical thresholds (i.e., Mean score of 6.09 in concussed as opposed to 2.98 in controls for the BDI scores, where the clinical threshold for moderate depression is 19; as for the BAI, clinical threshold for moderate anxiety is 16 and only male controls had significantly lower score (M=2.95) compared to other groups (M ≈6.15) (Beck, Epstein, Brown, & Steer, Reference Beck, Epstein, Brown and Steer1988; Beck & Steer, Reference Beck and Steer1993).
Given that Age and Level of education differences were minimal (≤ 1.5 year) and that the latter were unlikely to modulate EFE processing at such a young age, we did not include them as covariates. Two-tailed Pearson correlations were computed between relevant clinical variables (BAI, BDI, number of concussions, PCCS, and time since last concussion) and electrophysiological measures of EFE processing (P1 and N1 components), and behavioral measures. We used Greenhouse-Geisser correction whenever sphericity assumption was violated when computing ANCOVAs. Statistical significance was set at p<.05 and the false discovery rate (FDR) procedure was used when multiple comparisons corrections were needed.
RESULTS
EFE Task: Behavioral Measures
Descriptive data of reaction times and accuracy scores across groups can be found in Table 2. We first conducted a mixed factorial ANCOVA on reaction times with EFE (neutral, happy, angry) as within-subject factor and Sex (male or female) and Group (concussed or not) as between-groups factors. None of the interaction or main effects were found to reach statistical significance [Interactions of EFE * Sex * Group, F(2,152)=0.03, p=.97; EFE * Sex, F(2,152)=0.42, p=.66; EFE * Group, F(2,152)=0.15, p=.86; EFE * BDI, F(2,152)=0.1.42, p=.24; EFE * BAI, F(2,152)=0.11, p=.89; Group * Sex, F(1,76)=0.37, p=.55; and main effects of EFE, F(2,152)=0.14, p=.87; Group, F(1,76)=0.77, p=.38; Sex, F(1,76)=0.09, p=.77; BDI, F(1,76)=0.19, p=.66; BAI, F(1,76)=1.29, p=.26].
Table 2 Behavioral measures for angry, neutral and happy emotional facial expressions for each group and across sex

We then computed an ANCOVA with the same factors but this time, on accuracy scores. Figure 1 shows mean accuracy scores across groups. Only a main effect of Sex reached statistical significance, F(1,78)=15.31, p<.001, η p 2=.168, indicating that females were more accurate (M=91.88; SD=4.72) than males (M=86.23; SD=11.61). All the remaining effects were not statistically significant [Interactions of EFE * Sex * Group, F(1.48, 112.62)=1.69, p=.20; EFE * Sex, F(1.48, 112.62)=0.19, p=.76; EFE * Group, F(1.48, 112.62)=0.25, p=.71; EFE * BDI, F(1.48, 112.62)=0.14, p=.81; EFE * BAI, F(1.48, 112.62)=0.98, p=.36; Group * Sex, F(1, 76)=3.01, p=.09; and main effects of EFE, F(1.48, 112.62)=0.74, p=.44; Group, F(1, 76)=0.08, p=.78; BDI, F(1, 76)=0.11, p=.74; BAI, F(1, 76)=0.29, p=.59].

Fig. 1 Mean accuracy scores of the three emotional facial expressions across groups. ***=p<.001
EFE Task: Electrophysiological Measures
P1 component
First, we computed a mixed factorial ANCOVA with EFE (neutral, happy, angry) and Lateralization (PO7, PO8) as within-subject factors, Sex and Group as between-group factors, and BDI and BAI as covariates to verify if we could detect the lateralization effect observed in healthy controls (Batty & Taylor, Reference Batty and Taylor2003; Proverbio et al., Reference Proverbio, Brignone, Matarazzo, Del Zotto and Zani2006). Figure 2 depicts averaged P1 waveforms and scalp topographies recorded at electrodes PO7 and PO8 for each group. We found a statistically significant Group * Lateralization interaction, F(1,72)=9.11, p=.004, η p 2=.112. Post hoc analyses revealed that the amplitude of the P1 waveform component of controls, including both males and females, was significantly larger at PO8 (M=6.69; SD=4.43) relative to PO7 (M=4.77; SD=4.19) when the three EFE types (neutral, happy, angry) were combined t(37)=3.37, p=.002, d=0.45. Indeed, concussed athletes did not show the expected right-hemisphere lateralization of electrophysiological activity to EFE stimuli [Right: PO8 (M=4.75; SD=4.18) compared to Left: PO7 (M=4.88; SD=3.42), t(39)=-0.21, p=.89].
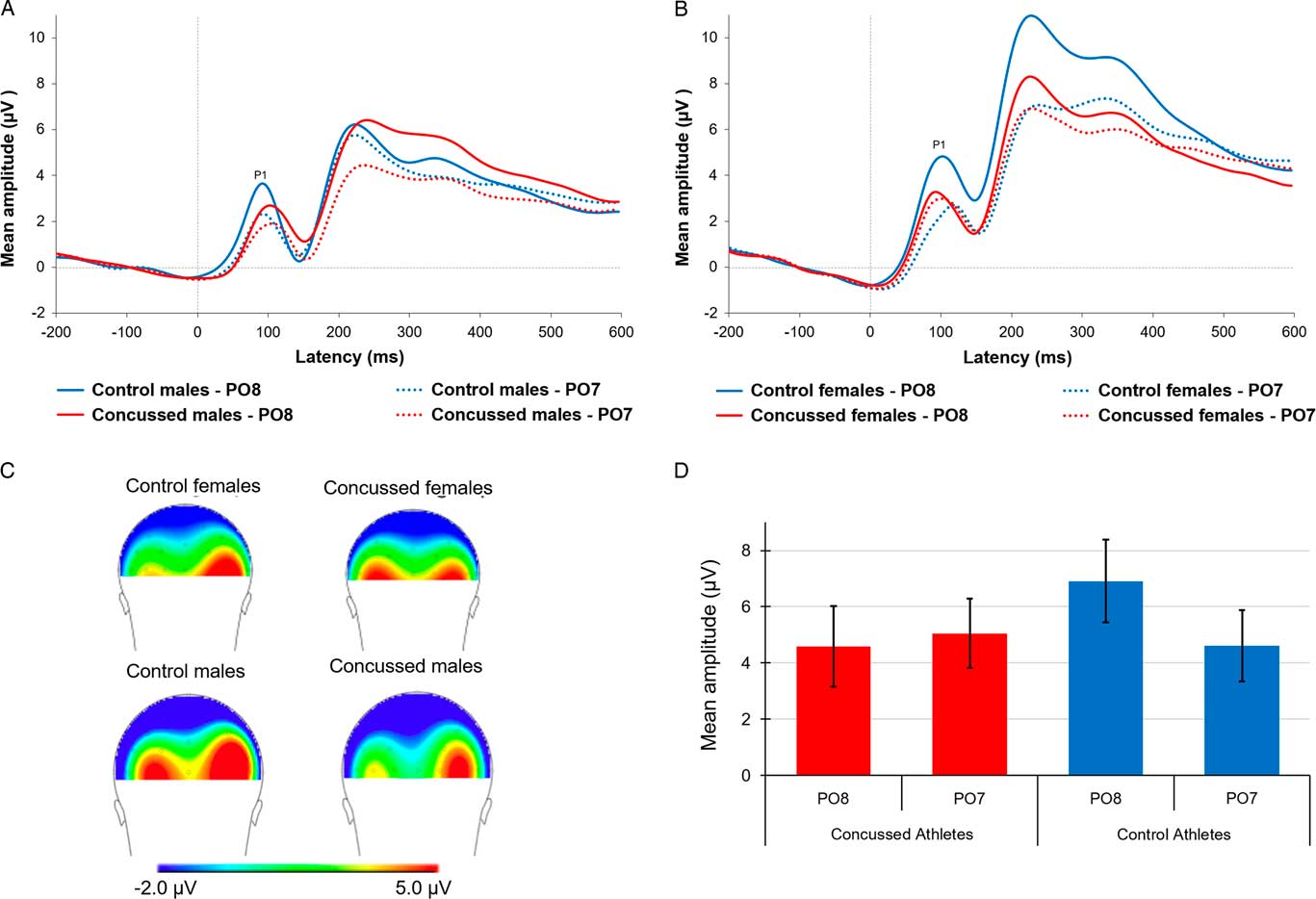
Fig. 2 P1 waveforms recorded at electrodes PO7 and PO8 reflecting the average response to the three emotional facial expressions in (A) concussed and control males and (B) concussed and control females. (C) Back view of scalp topographies for each group. (D) Bar graph representing averaged P1 amplitude recorded at electrodes PO7 and PO8 for each group. ** p< .01 and *p<.05
More importantly, we wanted to verify whether the P1 component differed across groups at PO8 as it was previously shown to be the electrode recording maximal P1 response to EFE processing (Proverbio et al., Reference Proverbio, Brignone, Matarazzo, Del Zotto and Zani2006). We found a significant between-group difference on P1 amplitude recorded at PO8 (t(76)=2.00, p=.05, d=0.45), such that the P1 amplitude was significantly suppressed in concussed relative to control athletes. P1 amplitude recorded at PO7 did not differ across groups t(76)=-.12, p=.90. The ANCOVA also revealed a significant main effect of Sex (F(1,72)=3.87, p=.05, η p 2=.051), such that males (M=4.48; SD=3.61) exhibited significantly smaller P1 responses to EFE stimuli than females (M=6.10; SD=3.62). All other statistics of the ANCOVA on P1 amplitude were not statistically significant, F-ratios ranging from the F(2,144)=2.63, p=.08 for the EFE * Group interaction to F(2,144)=.19, p=.83 for the Lateralization * EFE * Group * Sex interaction.
This first analysis result suggests that control athletes exhibited the expected right hemisphere dominance of the P1 amplitude, whereas concussed athletes did not, in addition to showing significantly suppressed right posterior activity relative to controls, irrespective of Sex, Type of EFE, and BAI and BDI scores (see Figure 2).
N1 component
Figure 3 depicts averaged N1 waveforms and scalp topographies recorded at Fz for each group. We conducted another mixed factorial ANCOVA with EFE (neutral, happy, angry) as within-subject factor, Sex and Group as between-group factors, and BDI and BAI as covariates on the N1 ERP component recorded at Fz. We found a statistically significant Group * Sex interaction (F(1,77)=4.06; p=0.05, η p 2=.051). Post hoc tests revealed that the concussed male athletes group (M=-3.56; SD=2.57) exhibited a N1 waveform component of significantly smaller amplitude than concussed females (M=-6.57; SD=3.31), (t(41)=-3.32; p=.01; d=1.02), and control males (M=-5.85; SD=2.93), (t(39)=-2.67; p=.03; d=.83), but only a trend toward significance when compared to control females (M=-5.69; SD=3.84), (t(39)=-2.10; p=.08). Differences were not statistically significant between control females and concussed females (t(40)=0.79; p=.56) or control males (t(38)=0.15; p=.88), and between concussed females and control males (t(40)=-0.74; p=.56) (see Figure 4).

Fig. 3 (A) Averaged N1 waveforms and (B) scalp topographies recorded at electrode Fz for each group when averaging all three emotional facial expressions.

Fig. 4 Bar graph representing averaged N1 amplitude recorded at electrode Fz for each group when averaging all three emotional facial expressions.
As expected from previous studies, we also found a significant main effect of Sex (F(1,77)=4.17; p=0.05, η p 2=.050), suggesting that females athletes (M=-6.15; SD=3.25) exhibited a greater N1 component amplitude relative to males (M=-4.69; SD=3.24). Taken together, these results suggest that the significant N1 amplitude reduction found in male concussed athletes is driving the Group × Sex interaction. No other interaction or main effects were found to reach statistical significance [Interactions of EFE * Sex * Group, F(2,154)=0.67, p=.51; EFE * Sex, F(2,154)=0.05, p=.95; EFE * Group, F(2,154)=1.95, p=.15; EFE * BDI, F(2,154)=0.24, p=.79; EFE * BAI, F(2,154)=0.03, p=.97; and main effects of EFE, F(2,154)=1.01, p=.37; Group, F(1,77)=.56; p=.46; BDI, F(1,77)=.08; p=.78; BAI, F(1,77)=.06; p=.81].
Correlational Analyses
All two-tailed Pearson correlations computed in concussed athletes between relevant clinical variables (Number of concussions, PCCS, Time since last concussion, BDI, and BAI) and electrophysiological measures of EFE processing (P1 at PO8 and N1 at Fz), and behavioral measures (Accuracy and Response time) did not reach statistical significance. However, when computing correlations in concussed males and females separately, we found that accuracy score was negatively correlated with the number of post-concussion symptoms in females (r(21)=-.44; p=.05). In males, we found a positive association between the time elapsed since the last concussion and accuracy scores (r(19)=.51; p=.03) (See Table 3).
Table 3 Correlations between clinical, behavioral, and electrophysiological measures in concussed male athletes, concussed female athletes, and concussed athletes as a group

Note. p-Values are presented in parentheses.
*=significant correlation at the .05 level.
DISCUSSION
The present study investigated the effects of sports-related concussions on the early neurophysiological substrates of EFE processing in asymptomatic and multi-concussed male and female athletes tested more than 3 months post-injury.
Results from this study reveal that multi-concussed male and female athletes exhibit a significant suppression of the P1 waveform component over P08, the right-hemisphere electrode site recording maximal P1 amplitude. These findings suggest that multiple concussions alter the dominant right-hemisphere response to early EFE processing (Batty & Taylor, Reference Batty and Taylor2003; Eimer & Holmes, Reference Eimer and Holmes2007; Halit et al., Reference Halit, de Haan and Johnson2000; Itier & Taylor, Reference Itier and Taylor2002). This right-hemisphere dominance suppression in early stages of EFE processing found in multi-concussed athletes could contribute to the known persistent alterations of the ability to identify and discriminate emotions in multi-concussion athletes (Léveillé et al., Reference Léveillé, Guay, Blais, Scherzer and De Beaumont2016).
The lateralized brain activity pattern to EFE found in control athletes is consistent with previous evidence highlighting a right-hemisphere dominance in emotional processing (Batty & Taylor, Reference Batty and Taylor2003; Itier & Taylor, Reference Itier and Taylor2004; Proverbio et al., Reference Proverbio, Brignone, Matarazzo, Del Zotto and Zani2006; Rossion et al., Reference Rossion, Delvenne, Debatisse, Goffaux, Bruyer, Crommelinck and Guérit1999). According to the right-hemisphere hypothesis, the right side of the brain specializes in perception, expression and experience of emotions, regardless of valence (Borod et al., Reference Borod, Cicero, Obler, Welkowitz, Erhan, Santschi and Whalen1998; Tucker, Reference Tucker1981). ERP studies have provided robust evidence to this hypothesis as right-hemisphere electrophysiological response to EFE is disproportionately elevated relative to that of the left hemisphere (Kestenbaum & Nelson, Reference Kestenbaum and Nelson1992; Laurian, Bader, Lanares, & Oros, Reference Laurian, Bader, Lanares and Oros1991; Münte et al., Reference Münte, Brack, Grootheer, Wieringa, Matzke and Johannes1998).
This right-hemisphere specialization to EFE processing is consistent with influential studies on the predominant role of the right superior temporal sulcus in EFE (Adolphs, Reference Adolphs2002; Adolphs, Damasio, Tranel, & Damasio, Reference Adolphs, Damasio, Tranel and Damasio1996; Narumoto, Okada, Sadato, Fukui, & Yonekura, Reference Narumoto, Okada, Sadato, Fukui and Yonekura2001). Further support to the right-hemisphere hypothesis comes from lesion studies reporting a relative sparing of facial expression recognition in patients with lesions of the left hemisphere relative to severe facial expression recognition impairments in patients with right hemisphere brain damage (Adolphs et al., Reference Adolphs, Damasio, Tranel and Damasio1996; Borod et al., Reference Borod, Cicero, Obler, Welkowitz, Erhan, Santschi and Whalen1998; Cicone, Wapner, & Gardner, Reference Cicone, Wapner and Gardner1980).
Of interest, a similar pattern of right posterior activity suppression during EFE processing is found in depression (Heller, Nitschke, & Miller, Reference Heller, Nitschke and Miller1998). In depression, compromised right-hemisphere dominance was associated with alterations of right-hemisphere function (Heller et al., Reference Heller, Nitschke and Miller1998). Knowing that multi-concussed athletes are particularly at risk of presenting with post-concussion symptoms including depressive symptoms (Fenton et al., Reference Fenton, McClelland, Montgomery, MacFlynn and Rutherford1993; Guskiewicz et al., Reference Guskiewicz, Marshall, Bailes, McCrea, Harding, Matthews and Cantu2007), the current study findings raise the intriguing possibility that the observed suppression of electrophysiological mechanisms of EFE perceptual processing might contribute to the known increased risk of depression symptoms in multi-concussed athletes (Chen et al., Reference Chen, Johnston, Petrides and Ptito2008; Guskiewicz et al., Reference Guskiewicz, Marshall, Bailes, McCrea, Harding, Matthews and Cantu2007). Future concussion studies should investigate both the functional as well as the structural connectivity of the right-hemisphere in relation with EFE processing.
As expected, we found a main effect of sex on behavioral data. Indeed, female athletes, whether concussed or not, were more accurate than their male counterparts to identify EFEs. This finding is consistent with previous evidence suggesting that females tend to outperform males in EFE identification (Chen et al., Reference Chen, Johnston, Petrides and Ptito2008; Hall & Matsumoto, Reference Hall and Matsumoto2004; Hampson et al., Reference Hampson, Vananders and Mullin2006; Léveillé et al., Reference Léveillé, Guay, Blais, Scherzer and De Beaumont2016). The absence of a Group by Sex interaction on EFE identification accuracy contrasts with recent findings from our group suggesting that concussed males, and not females, were significantly impaired in recognizing negative emotions compared to same-sex controls (Léveillé et al., Reference Léveillé, Guay, Blais, Scherzer and De Beaumont2016).
It is important to note, however, that the observed EFE identification alterations were obtained when performing a morphed emotion recognition task known for its sensitivity to detect subtle changes of EFE processing in which pictures of a male face expressing basic emotions (anger, disgust, fear, happiness, sadness, surprise) were morphed with another emotion. In the current study, however, the EFE identification task was adapted to allow optimal ERP recordings while not modulating task difficulty as a function of the degree of morph between two emotional facial expressions. Indeed, a marked ceiling effect was found on accuracy scores across groups and emotion types, which hampered the sensitivity of the proposed EFE identification task to potential concussion effects. Future studies adapting the morphed emotion recognition task to an ERP protocol would allow to investigate potential associations between P1 amplitude alterations found herein and EFE identification in concussed athletes.
In addition, our results reveal that male concussed athletes show a suppressed N1 component in comparison with all three other groups, across emotion types, whereas no significant N1 component difference was found in female concussed athletes relative to same-sex controls. This indicates that the effects of having sustained multiple concussions more than 3 months before testing on the anterior N1 component during an EFE identification task, which is thought to reflect early discrimination of emotional content, is specific to male athletes.
Of interest, the observed amplitude reduction of the N1 component found in concussed male athletes is in line with previous studies reporting reduced N1 component amplitude in depressed patients (Burkhart & Thomas, Reference Burkhart and Thomas1993; Dai & Feng, Reference Dai and Feng2011). Further studies including concussed male and female athletes reaching the clinical threshold for depressive disorders are needed to validate the relationship between the size of the N1 component evoked by an EFE identification task and depression symptoms. In addition, future longitudinal studies could investigate the potential functional effects of these sex specific N1 alterations pattern found in concussed athletes (Guskiewicz et al., Reference Guskiewicz, Marshall, Bailes, McCrea, Harding, Matthews and Cantu2007).
Finally, findings from this study are in line with previous evidence of durable concussion effects on P1 and N1 components elicited by classic attentional tasks stimuli (Gosselin et al., Reference Gosselin, Thériault, Leclerc, Montplaisir and Lassonde2006; Moore et al., Reference Moore, Broglio and Hillman2014, Reference Moore, Lepine and Ellemberg2017). On the other hand, P1 and N1 components elicited by emotional stimuli in this study generated a distinct pattern of right-hemisphere dominance for the P1 component as well as a significant N1 deflection over anterior electrode sites. The latter pattern of maximal brain activity for emotional stimuli contrasts sharply with maximal activity recorded centrally and posteriorly for the P1 and N1 components, respectively, when concussed athletes were presented with non-emotional stimuli (Gosselin et al., Reference Gosselin, Thériault, Leclerc, Montplaisir and Lassonde2006; Moore et al., Reference Moore, Broglio and Hillman2014, Reference Moore, Lepine and Ellemberg2017), therefore, supporting the novelty of current study findings.
One of the main limitations of this study is having to rely on concussion history self-reports as opposed to medical records to address consequences of a sports concussion that occurred months or even years before testing. The impact of this methodological limitation was attenuated by excluding participants who reported being uncertain about their concussion history. Prospective studies conducted with young male and female athletes are needed to validate the residual effects of concussions on electrophysiological markers of EFE processing. Finally, larger-scale studies including a more heterogeneous sample of concussed athletes, including single-concussed athletes, tested at different time points after their concussion, would be necessary if we are to validate findings from the current study. Nonetheless, this study provides new evidence that multiple concussions induce long-term changes to early perceptual and processing stages of EFE processing in asymptomatic athletes and that the neurophysiological mechanisms underlying the processing of emotional stimuli are distinctively affected across sex.
ACKNOWLEDGMENTS
The authors declare no conflict of interests. This work was supported by the UQTR Research Chair on the Neurobiology of traumatic brain injury awarded to LDB. Authors Statement: This manuscript has not been published elsewhere and has not been submitted simultaneously for publication elsewhere.
Supplementary material
To view supplementary material for this article, please visit https://doi.org/10.1017/S1355617718000231